Myeloproliferative neoplasm (MPN) animal models accurately re-capitulate human disease in mice and have been an important tool for the study of MPN biology and therapy. Transplantation of BCR-ABL transduced bone marrow into irradiated syngeneic mice established the field of MPN animal modeling. Genetically engineered MPN animal models have enabled detailed characterization of the effects of specific MPN-associated genetic abnormalities on hematopoietic stem and progenitor cells (HSPCs). Xenograft models have allowed the study of primary human MPN-propagating cells in vivo. JAK2V617F , the most common molecular abnormality in BCR-ABL negative MPN, has been extensively studied using retroviral, transgenic, knock-in and xenograft models.
- •
Retroviral transduction of BCR-ABL into murine bone marrow cells followed by transplantation into irradiated syngeneic mice established the field of myeloproliferative neoplasm (MPN) animal modeling.
- •
The effects of the JAK2V617F mutation in hematopoietic cells has been extensively modeled in vivo using retroviral, transgenic, knock-in, and xenograft murine models.
- •
The considerable phenotypic differences observed between broadly similar JAK2V617F murine models highlights the inherent variability in murine models that can occur as a result of multiple factors, such as promoter, oncogene expression level, murine versus human protein, and mouse strain.
- •
Mutant oncogenes found in human acute myelogenous leukemia (AML), such as RAS and FLT3 , induce MPNs in mice, indicating that these genetic lesions are insufficient to cause AML and suggesting that additional cooperating genetic events are required for AML development.
- •
As the increasing genetic complexity of MPNs has become apparent, additional genetic models have been developed to investigate the functional effects and therapeutic susceptibilities of compound genetic lesions in MPNs.
Introduction
Animal models have been used extensively in the study of myeloproliferative neoplasms (MPNs) and have played a key role in advancing the biologic understanding of these diseases ( Boxes 1–3 ). In general, these models have faithfully recapitulated human MPNs in mice, enabled detailed characterization of the effects of specific MPN-associated genetic abnormalities on the hematopoietic stem and progenitor cell (HSPC) compartment, and provided excellent in vivo models for testing novel MPN therapeutic agents. In this review, the authors focus primarily on murine models of the JAK2V617F mutation and on the insights these have provided, and also briefly outline the central role BCR-ABL models played in establishing and developing the field. Models of additional genetic lesions found in human MPNs are discussed, and genetic models that induce an MPN phenotype in mice are outlined. The authors describe the use of JAK2V617F models in the preclinical development of janus kinase 2 (JAK2) inhibitors and other MPN therapies. Studies of the bone marrow microenvironment that have been performed using MPN models are summarized, and some thoughts are provided as to how MPN animal models might be used in the future.
In the retroviral bone marrow transplantation (BMT) assay, bone marrow is harvested from donor mice that have been stimulated with 5-fluorouracil (5-FU). The 5-FU–stimulated bone marrow cells are then transduced ex vivo with a retroviral construct expressing the gene of interest. This results in stable but random integration of the transgene into the host cell genome. The transduced cells are then transplanted into irradiated syngeneic mice, where hematopoietic reconstitution is polyclonal. Transgene expression is generally high (nonphysiologic), and differences in the site of retroviral integration may result in variation in transgene expression level. Because retroviruses preferentially transduce mitotically active cells, quiescent long-term hematopoietic stem cells (LT-HSCs) are relatively resistant to retroviral integration.
Genetically engineered murine models can be classified as transgenic or endogenous. Transgenic mice express the gene of interest under the control of ectopic promoter and enhancer elements. They are generated by pronuclear injection of the transgene into a single cell of a mouse embryo, in which it randomly integrates into the mouse genome. Knock-in mice express the gene of interest from their native promoters and thus represent endogenous genetically engineered mice. They are generated through the modification of embryonic stem (ES) cells using a DNA construct that contains sequences homologous to the target gene. The relevant mutation is thus introduced to the gene of interest under the control of its endogenous promoter via homologous recombination in ES cells. Conditional knock-in mice use site-specific recombinases, such as Cre, to control the timing and tissue-specificity of gene expression. Inducible transgenic and knock-in models use exogenous ligands (eg, doxycycline or interferon) to reversibly control the timing of target-gene expression. In general, transgenic models result in overexpression of the gene of interest through the use of exogenous promoters, whereas expression is at physiologic levels in knock-in models, in which the gene of interest is expressed from its endogenous promoter. Knock-out mice are genetically engineered mice, in which the gene of interest is inactivated by replacing it or disrupting it with an artificial piece of DNA. This is achieved in ES cells via homologous recombination. Because germline homozygous gene deletions can be embryonically lethal, conditional knock-out mice are often generated to circumvent this problem.
In xenograft murine MPN models, human MPN CD34 + cells are transplanted and propagated in immunodeficient mice. To achieve engraftment, investigators have used NOD/SCID (nonobese diabetic/severe combined immunodeficiency) mice absolutely deficient in B and T cells and having impaired natural killer cell function. Further impairment of the murine immune system has been achieved by depletion of natural killer cells using CD122 antibodies or by crossing with a mouse strain deficient for the common gamma chain of the interleukin (IL)-2 receptor (NSG mice). Although these models allow the study of primary human MPN cells in vivo, some aspects of the bone marrow microenvironment are not recapitulated because of the absence of immune cells and species incompatibility for some cytokines and cytokine receptors.
MPN murine models
BCR-ABL
The faithful modeling of human MPNs in mice began in 1990 with the demonstration that retroviral transduction of BCR-ABL into murine bone marrow cells, followed by transplantation into irradiated syngeneic mice, recapitulated human chronic myelogenous leukemia (CML) in mice. These groundbreaking experiments established the retroviral BMT assay and, with it, the ability to accurately model human hematologic malignancies in mice.
Retroviral
The retroviral BCR-ABL model has provided several important insights into the molecular pathophysiology of CML. These include the demonstration that BCR-ABL expression in vivo is sufficient to induce CML, the identification of key BCR-ABL activated downstream signaling pathways in CML stem cells, and the provision of an accurate preclinical model that continues to be used in the evaluation of novel CML therapeutics.
Transgenic
Several BCR-ABL transgenic models have been developed and used primarily to investigate the kinetics and therapeutic susceptibilities of CML-propagating stem cells. Here, we discuss 2 models that have been particularly informative in this regard. Perez-Caro and colleagues generated transgenic mice that expressed BCR-ABL under the control of the Sca-1 promoter, thus restricting transgene expression to the Sca-1–positive cell population, which includes HSCs. The mice developed a CML-like disease characterized by neutrophilia and hepatosplenomegaly as a result of extramedullary hematopoiesis, with most animals progressing to blast crisis. A subset of mice developed additional solid tumors, indicating that Sca-1 –driven BCR-ABL expression is not restricted to hematopoietic cells. The course of the CML-like disease was not modified by treatment with imatinib, suggesting that the HSC compartment in CML is insensitive to ABL kinase inhibition.
Koschmieder and colleagues developed an inducible BCR-ABL transgenic model in which the tetracycline transactivator protein was placed under the control of the murine stem cell leukemia ( SCL ) gene 3′ enhancer, and double transgenic SCL– tetracycline transactivator protein/ BCR-ABL mice were generated. With tetracycline withdrawal, BCR-ABL expression was induced in the HSPC compartment. These mice developed a CML-like disease that is reversible and reinducible, suggesting that sustained BCR-ABL expression is required for the development of a CML disease phenotype. The CML-initiating cell population was found to be present in the lineage low Sca1 + Kit high (LSK) compartment, and more recently, this population has been further refined and shown to be present solely in the most immature LT-HSC compartment. BCR-ABL –positive LT-HSCs outcompete normal LT-HSCs and aberrantly mobilize to the spleen in this model. Finally, CML-propagating stem cells have been found to be resistant to treatment with imatinib alone, whereas the combination of imatinib with histone deacetylase inhibitors seems to mitigate some of this resistance, in this BCR-ABL –inducible murine model.
Xenograft
Xenograft models of chronic-phase CML have been problematic in that most SCID-repopulating cells are BCR-ABL negative, a finding thought to relate, at least in part, to the presence of a reservoir of normal HSCs in chronic-phase CML. These findings suggest that BCR-ABL –positive SCID-repopulating cells (SRCs) from chronic-phase CML do not have a proliferative advantage over wild-type SRCs. A major caveat of these experiments is the incompatibilities between cytokine receptors on human cells and cytokines produced by a murine microenvironment. Transplanting BCR-ABL –positive cells taken from long-term culture-initiating cell assays results in more durable leukemic cell engraftment in NOD/SCID mice, as does the transplantation of cells from patients with CML in accelerated or blast phase.
JAK2V617F
JAK2V617F is the most common molecular abnormality in BCR-ABL –negative MPNs, and it has been extensively studied in vivo using retroviral, transgenic, knock-in, and xenograft murine models. In general, these models reliably recapitulate the clinical features of human MPNs in mice ( Figs. 1 and 2 , Table 1 ), and they have helped advance the biologic understanding of JAK2V617F -mediated MPNs in humans.
First Author | JAK2 Origin | Strain | Type | VF:WT Expression | Homozygotes | Phenotype | Myelofibrosis |
---|---|---|---|---|---|---|---|
Akada | Mouse | 129Sv/C57Bl/6 | Conditional | <1:1 | Yes | PV-like | Yes |
Marty | Mouse | 129Sv/C57Bl/6 | Constitutive | ∼1:1 | No | PV-like | Yes |
Mullally | Mouse | 129Sv/C57Bl/6 | Conditional | <1:1 | No | PV-like | Yes, with BMT |
Li | Human | 129Sv/C57Bl/6 | Conditional | ∼1:1 | No | ET-like | No |
Retroviral
Immediately following the discovery of the JAK2V617F mutation, retroviral models were generated to assess the phenotypic effects of JAK2V167F in vivo. Mice transplanted with JAK2V617F expressing donor bone marrow developed striking polycythemia after a short latency with a high degree of penetrance. Leukocytosis, splenomegaly resulting from extramedullary hematopoiesis, and the development of myelofibrosis were also seen. All groups observed constitutive activation of Jak2 kinase signaling and Stat5 phosphorylation in addition to cytokine-independent colony formation. These models demonstrated that JAK2V617F expression in vivo was sufficient to induce an MPN phenotype and indicated that genetic modifiers of JAK2V617F -mediated fibrosis exist (more prominent fibrosis was seen in the Balb/c strain compared with C57Bl/6 strain).
Transgenic
Three groups generated transgenic JAK2V617F models in 2008, with the main goal of correlating disease phenotype with differences in JAK2V617F gene dosage. Tiedt and colleagues conditionally expressed human JAK2V617F from the endogenous human JAK2 promoter and crossed with Vav-Cre or Mx1-Cre transgenic mice to induce hematopoietic specific JAK2V617F expression. The ratio of human JAK2V617F to murine wild-type Jak2 expression was quantified in mRNA. Xing and colleagues expressed human JAK2V617F from the Vav promoter, whereas Shide and colleagues expressed murine Jak2V617F from the H-2Kb promoter.
In the model of Tiedt and colleagues, JAK2V617F Vav-Cre mice had constitutive and sustained Cre expression, low transgene copy number, and a JAK2 mutant–to–wild-type ratio of approximately 0.5. In JAK2V617F MxCre mice, Cre expression was induced following treatment with polyinosinic:polycytidylic acid (pI:pC) to activate the interferon response. Transgene copy number was higher than in JAK2V617F Vav-Cre animals and the JAK2 mutant–to–wild-type mRNA ratio was approximately 1.0. The lower copy number in Vav-Cre compared with MxCre was attributed to sustained Cre expression in the Vav-Cre model, leading to more efficient deletion of additional transgene copies. The phenotype of JAK2V617F Vav-Cre mice was more consistent with essential thrombocythemia (ET) (high platelets, normal white blood cell count, and hemoglobin), whereas the phenotype JAK2V617F MxCre animals more closely recapitulated polycythemia vera (PV) (elevated hemoglobin with suppressed erythropoietin, high white blood cell count and high platelets). In the model of Xing and colleagues, the level of mutant JAK2 expression was extremely low and the phenotype most consistent with ET. In the model of Shide and colleagues, the MPN was incompletely penetrant in the founder line in which mutant Jak2 was expressed at a lower level than wild-type Jak2. In a second founder line in which mutant Jak2 expression was higher than wild-type Jak2, an MPN with myelofibrotic transformation developed.
The major conclusion to be drawn from the transgenic models is that the level of JAK2V617F expression influences the MPN phenotype, with the suggestion that lower JAK2V617F expression results in an ET phenotype and higher JAK2V617F expression gives rise to a PV phenotype. However, some of the MPN phenotypic differences observed by Tiedt and colleagues could be related to tissue-specific Cre effects (ie, Vav-Cre versus Mx1-Cre). JAK2V617F expression is driven by exogenous promoters in some of these transgenic models, which could also influence the MPN phenotype.
Knock-in
Four JAK2V617F knock-in models were published in 2010. These were generated with the major goals of (1) recapitulating human MPN through physiologic Jak2V617F expression, (2) assessing the impact of Jak2V617F gene dosage on disease phenotype, and (3) evaluating the effects of Jak2V617F on the HSPC compartment.
The models of Akada, Marty, and Mullally and their colleagues all expressed murine Jak2V617F from the endogenous murine Jak2 promoter but each in a slightly different manner. Akada and colleagues and Mullally and colleagues generated conditional Jak2V617F knock-in models, whereas Jak2V617F expression was constitutive in the model of Marty and colleagues. Human JAK2V617F was conditionally expressed from the endogenous murine Jak2 promoter in the model of Li and colleagues. In all of the models, the ratio of mutant to wild-type Jak2 expression was 1:1 or less. The model of Akada and colleagues was the only one in which homozygous Jak2 mutant mice were generated, but because the Jak2 mutant allele expressed at approximately 50% of the wild-type allele, the level of Jak2V617F expression in homozygous mice was relatively low. A summary of the Jak2V617F knock-in models is outlined in Table 1 , and a schematic representation of the targeting strategies for each of the models is given in a recent review by Li and colleagues.
The phenotype of the 3 models that expressed murine Jak2V617F was broadly similar. In each of these models, mice heterozygous for the Jak2V617F mutation had profound erythrocytosis, leukocytosis, splenomegaly caused by extramedullary hematopoiesis, and myelofibrosis that developed over time (although this was only observed in transplant recipients by Mullally and colleagues ). Compared with the other models, the hematologic phenotype was relatively mild in the model of Li and colleagues. Only a modest increase in platelets and hemoglobin, more reminiscent of ET than of PV, was observed (erythropoietin levels were not suppressed), whereas approximately 10% mice developed marked erythrocytosis with prolonged follow-up. In this model, human JAK2V617F was expressed from the endogenous murine Jak2 promoter, and whether human JAK2V617F signals differently in murine cells remains undetermined.
In all of the models, the MPN was transplantable into secondary recipients, indicating that the MPN is cell autonomous. The ability to transplant the disease enabled a functional analysis of the stem cell properties of Jak2V617F-expressing HSCs. Recent reports on this analysis suggest that Jak2V617F mutant HSCs have a clonal advantage over wild-type HSCs. Mullally and colleagues found that Jak2V617F mutant HSCs have a subtle competitive repopulating advantage over normal HSCs, whereas Marty and colleagues and Lundberg and colleagues recently described a stronger competitive advantage for Jak2V617F mutant HSCs in a knock-in model and a transgenic model, respectively. The HSC phenotype in the knock-in model of Li and colleagues differs from that of the other researchers in that LSK cell numbers were reduced in JAK2V617F mice and demonstrated decreased cell cycle and increased senescence. In competitive repopulation experiments, JAK2V617F mutant HSCs were outcompeted by wild-type cells in primary BMT recipients as early as 5 weeks post transplantation, preventing transplantation of the MPN. It remains unclear whether this finding is related to expression of human JAK2V617F from the endogenous murine Jak2 promoter or to another cause such as increased replicative stress or immunologic rejection. Finally, by transplanting sorted populations of progenitor cells, Mullally and colleagues found that expanded Jak2V617F progenitor cell populations such as megakaryocytic erythroid progenitor cells are incapable of reconstituting MPN in a transplanted animal. These results are consistent with previously published reports indicating that oncogenic kinase alleles are incapable of transforming progenitor cells.
The main conclusions from the Jak2V617F knock-in models are that (1) physiologic heterozygote expression of murine Jak2V617F in mice causes a polycythemia phenotype, (2) the Jak2V617F mutation seems to provide a clonal advantage to HSCs, and (3) Jak2V617F does not confer self-renewal properties to committed myeloid progenitor cells. Although these models closely recapitulate human MPNs, some differences remain, including the fact that although Jak2V617F expression is driven by the endogenous Jak2 promoter in these knock-in models, the mutant allele is expressed in all hematopoietic cells, instead of in an MPN clone as occurs in humans. It is also important to note that reconstitution in competitive repopulation experiments is polyclonal and occurs in a bone marrow niche that has been perturbed by irradiation.
Xenograft
To study BCR-ABL –negative MPN cells in vivo, xenograft models have been generated. These models enable a functional assessment of the repopulating capacity of primary human MPN CD34 + cells, which possess the full germline and somatic genotype of human MPNs.
Peripheral blood CD34 + cells from patients with myelofibrosis engraft NOD/SCID mice and show clonal hematopoiesis with myeloid skewing. Two independent groups have found relatively poor engraftment of JAK2V617F mutant CD34 + cells from patients with PV and ET, and the ratio of JAK2V617F to JAK2 wild-type SRCs was found to be higher in myelofibrosis than in PV. Functionally, JAK2V617F SRCs did not gain a proliferative advantage compared with wild-type SRCs over time.
The major conclusions from these studies are that (1) JAK2V617F is found in a functionally competent LT-HSC population, (2) the functional JAK2V617F LT-HSC compartment is expanded in myelofibrosis compared with PV, and (3) poor JAK2V617F CD34 + engraftment in xenografts may be related to down-regulation of chemokine receptor 4 (CXCR4). Its important to note that incompatibilities between human cytokine receptors and murine cytokines in xenograft models may be particularly relevant in assessing the in vivo functional effects of the JAK2V617F allele, which activates cytokine receptor signaling. In general, reliable xenotransplantation across a wide spectrum of genetically diverse MPN requires further optimization.
MPLW515L
A somatic activating mutation in MPL ( MPLW515L ) was originally identified in JAK2V617F –negative myelofibrosis patients and MPLW515L or MPLW515K mutations were subsequently found in approximately 5% and 1% of patients with myelofibrosis and ET, respectively. In a retroviral BMT assay, MPLW515L induced an MPN in mice, characterized by leukocytosis, thrombocytosis, splenomegaly, and reticulin fibrosis. This model has subsequently been used to provide preclinical evidence in support of the use of JAK2 kinase inhibitors in patients with MPN who carry the MPLW515L mutation.
TET2
TET2 deletions and loss-of-function mutations occur in up to 12% of patients with MPN. Initial studies by Delhommeau and colleagues indicated that TET2-JAK2V617F co-mutated CD34 + cells from patients with MPN show an increased capacity compared with JAK2V617F -mutated CD34 + cells to repopulate NOD/SCID mice, suggesting that loss of TET2 enhances HSC self-renewal. These findings have been validated with the publication by several groups of Tet2 conditional knock-out mice, which demonstrate that Tet2 null HSCs have a competitive repopulating advantage compared with wild-type HSCs. Future studies will likely focus on murine modeling of compound mutants (eg, Jak2V617F/Tet2 ) to evaluate the impact of loss of Tet2 on the MPN phenotype, on MPN-propagating stem cell function, and on the therapeutic susceptibilities of Jak2V617F/Tet2 compound mutant hematopoietic cells.
LNK
Heterozygote loss-of-function mutations in the inhibitory adaptor protein LNK were originally reported in JAK2 wild-type patients with MPN. Overall, the frequency of LNK mutations in MPN is low, and although mutations in LNK have been reported in JAK2V617F –positive MPN, it is not known if both mutations occur in the same clone. Knock-out mice for Lnk were generated before the identification of LNK mutations in MPNs; Lnk null mice exhibit an MPN phenotype (CML-like disease), whereas heterozygous Lnk mice have an intermediate disease phenotype. Lnk negatively regulates Mpl through its SH2 domain. Consistent with this, Lnk null mice exhibit potentiation of Jak2 signaling in response to thrombopoietin, increased HSC quiescence, and decelerated HSC cell-cycle kinetics. Lnk retains the ability to bind and inhibit Jak2V617F and, consistent with this, loss of Lnk exacerbates JAK2V617F-mediated MPN and accelerates the development of JAK2V617F-induced myelofibrosis, through the potentiation of JAK2V617F signaling. These studies in Lnk null mice have provided important insights into the role of the thrombopoietin/Mpl/Jak2/Lnk pathway in regulating HSC self-renewal and quiescence and informed the understanding of MPN stem cell kinetics.
c-CBL
Mutations in c-CBL were identified in patients with myelofibrosis in a region of acquired uniparental disomy on chromosome 11q. Most variants were missense substitutions in the RING or linker domains that abrogated CBL ubiquitin ligase activity. These mutations were modeled in mice using retroviral overexpression in c-Cbl null cells and were demonstrated to further augment the enhanced cytokine sensitivity of c-Cbl null HSPCs, indicating a gain-of-function activity greater than that seen in c-Cbl null cells alone. Subsequent c-Cbl mutant knock-in models have demonstrated that mutations in the c-Cbl RING finger domain (but not germline deletion of c-Cbl ) cause loss of E3 ubiquitin ligase activity and an inability of c-Cbl to interact with target receptor tyrosine kinases, such as Flt3. This, in turn, causes activation of downstream signaling pathways and the development of an MPN in vivo, which is dependent on Flt3 signaling for the maintenance of disease.
Other Genetic Lesions in MPNs
Other genetic lesions have been described in the epigenetic machinery in MPNs such as in ASXL1 , EZH2, and DNMT3A . IDH1 and IDH2 mutations have been identified at low frequency in MPNs. Genetic murine models exist for some of these genetic abnormalities, whereas others are in development.
Other MPN Animal Models
Additional murine models have an MPN phenotype, although they are driven by alleles that are not common, somatically mutated drivers of MPN in humans. These include the K- Ras G12D knock-in model, in which oncogenic K- Ras was conditionally expressed from its endogenous murine promoter, and the FLT3 internal tandem duplication (ITD) knock-in model, in which an FLT3 -ITD mutation was engineered into the Flt3 locus and expressed from the endogenous murine Flt3 promoter. RAS and FLT3 are both recurrently mutated in human AML, but K- Ras G12D and Flt3 ITD mice both develop MPNs, indicating that these genetic lesions alone are insufficient to induce AML. K- Ras G12D mice develop an MPN characterized by leukocytosis, extramedullary hematopoiesis, and growth factor hypersensitivity, whereas Flt3 ITD mice develop MPNs reminiscent of human chronic myelomonocytic leukemia, with prominent monocytosis and splenomegaly.
The activator protein 1 transcription factor, JunB, is a transcriptional regulator of myelopoiesis and its expression is downregulated in AML. Inactivation of JunB in postnatal mice results in LT-HSC expansion and an MPN resembling early CML. Murine models of some of the genetic lesions found in human juvenile myelomonocytic leukemia have been developed, and these also result in an MPN phenotype in mice. Conditional deletion of Nf1 and conditional activation of Ptpn11 D61Y both induce an MPN characterized by leukocytosis and hypersensitivity to granulocyte-macrophage colony-stimulating factor.
Each of these in vivo models has advanced the biologic understanding of these genetic abnormalities, particularly with respect to their effects on the HSPC compartment, and they continue to serve as useful preclinical models for testing novel pharmacologic agents.
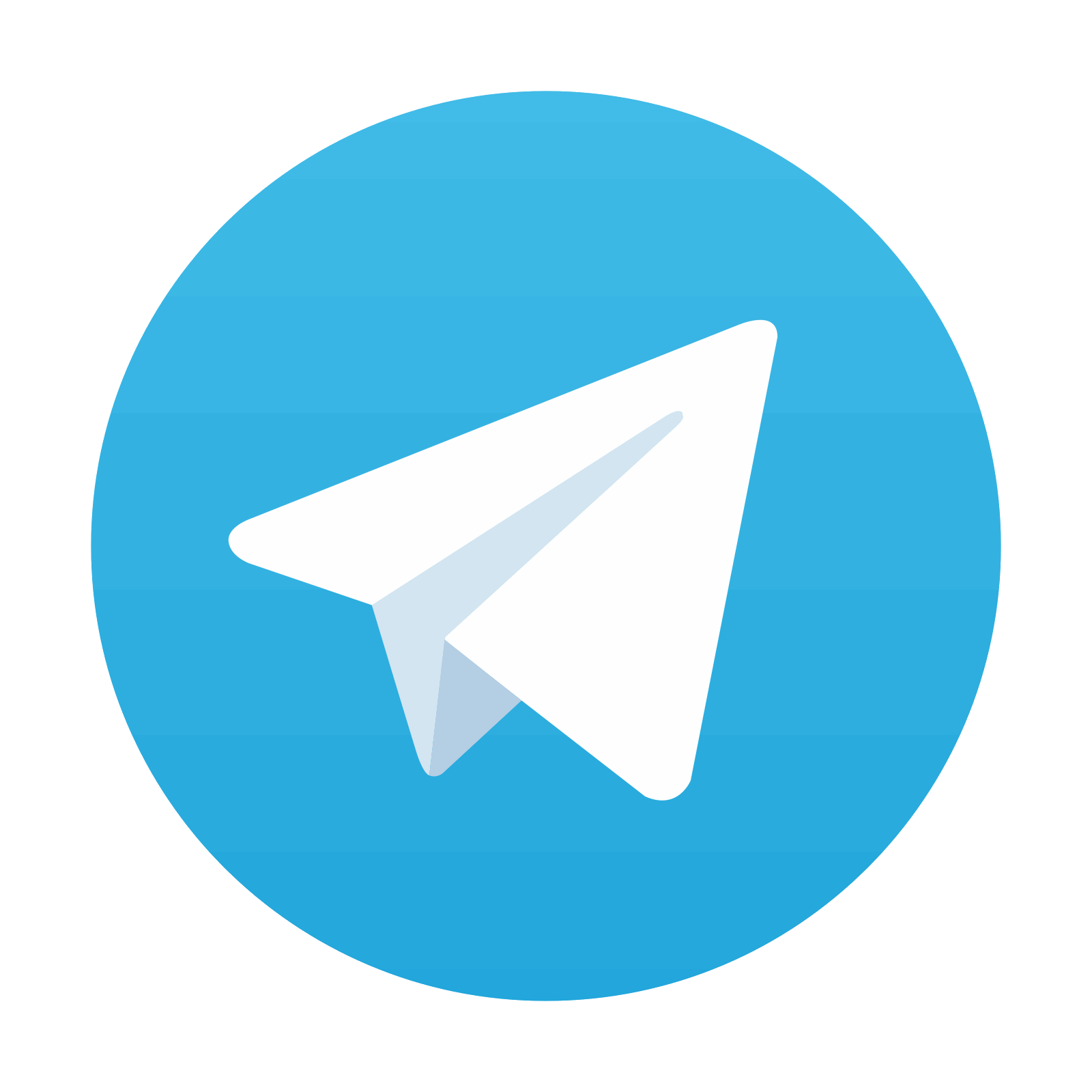
Stay updated, free articles. Join our Telegram channel

Full access? Get Clinical Tree
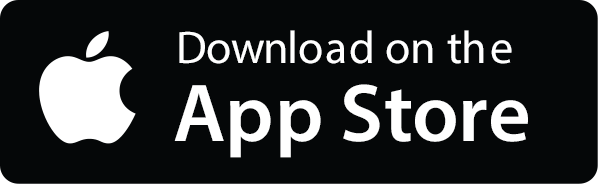
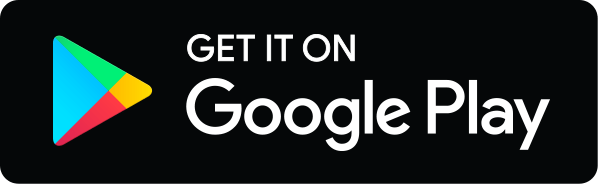