Multimodality Theranostics and Molecular Imaging in the Diagnosis and Treatment of Lung Cancer
Kirtee Raparia
Kelvin Wong
Stephen Wong
King C. Li
Philip T. Cagle
Development of image-guided, multimodality diagnostic and therapeutic systems is underway and promises to merge pathology and radiology for improved survival of lung cancer. This novel system could be potentially used for early diagnosis and treatment of peripheral lung cancer, which constitutes more than half of lung cancer cases.1 Molecular imaging and electromagnetic (EM) tracking can be used to guide a steerable needle that allows imageguided diagnosis along with diagnostic and therapeutic devices including radiofrequency ablation (RFA) needle, fiberoptic fluorescence imaging probe for fluorescence molecular imaging,2 and laser-induced photothermal therapy; in conjunction with molecular contrast agents including 18F-fluoro-deoxy-glucose, fluorescence molecular imaging contrast agents, and metallic nano-shells (Figs. 20-1 and 20-2). The lung cancer diagnostic and therapeutic system can potentially be used to provide a cost-effective diagnosis and treatment of cancer, in addition to the more established minimally invasive tool such as RFA.
OPTICAL IMAGING FOR IN VIVO MOLECULAR IMAGING
Despite advances in imaging, current techniques such as computerized tomography (CT) cannot visualize tumor morphology and architecture at the cellular level. Currently, in clinical situations a surgical tissue biopsy or needle aspiration is required for histopathologic diagnosis. Because many tumors are heterogeneous, it can be difficult to determine the optimal site to biopsy and sampling error may lead to incorrect diagnoses. Therefore, a minimally invasive technique to provide in vivo data on cellular morphology and molecular expression in real time would radically improve clinical diagnosis and monitoring tumor response. Optical molecular imaging technologies can meet this important clinical need by delivering real-time, in vivo images with subcellular resolution, which can be further enhanced by using targeted optical contrast agents. Nuclear and cellular morphology is observed in thin optical sections, which is similar to conventional
histology but without removing tissue, sectioning, and staining. The optical contrast agents can enhance the visualization of the molecular changes, such as expression of oncogenes or tissue-specific receptors. This information can significantly benefit patient care, by providing earlier and more specific detection of disease. The use of molecular imaging also has the potential to develop targeted therapeutics agents.3
histology but without removing tissue, sectioning, and staining. The optical contrast agents can enhance the visualization of the molecular changes, such as expression of oncogenes or tissue-specific receptors. This information can significantly benefit patient care, by providing earlier and more specific detection of disease. The use of molecular imaging also has the potential to develop targeted therapeutics agents.3
Optical coherence tomography (OCT) is a novel noninvasive technique, which can detect tissue structure at a resolution of up to 10 to 20 μm. OCT imaging system has been shown to clinically distinguish normal and abnormal cervical tissues, including dysplasia and cancer.4,5,6 and 7 Confocal scanning laser microscopy has been shown to identify benign and malignant pigmented skin lesions in vivo.8,9 and 10 There is also preliminary data on its role in diagnosing mycosis fungoides.11 It may also be a promising tool in the diagnosis of nonneoplastic skin lesions such as psoriasis, molluscum contagiosum, discoid lupus erythematosis, folliculitis, and allergic contact dermatitis.12,13,14,15,16 and 17 Real-time confocal reflectance microscopy can also be potentially used to evaluate the margins
in vivo in Mohs surgery for nonmelanoma skin cancers.18,19 This could avoid the use of frozen sections in these situations, which takes longer time. Confocal microscopy has also been reported to be of significance in detection of oral cavity neoplasia.20 It also allowed imaging of the urothelium, the superficial and deep portions of the lamina propria, the muscularis propria, and the serosa of the bladder wall of the rats in vivo.21
in vivo in Mohs surgery for nonmelanoma skin cancers.18,19 This could avoid the use of frozen sections in these situations, which takes longer time. Confocal microscopy has also been reported to be of significance in detection of oral cavity neoplasia.20 It also allowed imaging of the urothelium, the superficial and deep portions of the lamina propria, the muscularis propria, and the serosa of the bladder wall of the rats in vivo.21
Although there is limited study of these advanced imaging techniques in lung cancer, these may be a promising tool for in vivo imaging of lung lesions. Confocal microscopy has been shown to visualize normal alveolar ducts, alveolar macrophages,22,23 and human lung cancer cell lines.24 A recent study of total of 281 OCT images and the corresponding bronchial biopsies suggested that autofluorescence bronchoscopy-guided OCT imaging of bronchial lesions is technically feasible.25 Quantitative measurement of the epithelial thickness showed that invasive carcinoma was significantly different than carcinoma in situ (p = 0.004) and dysplasia was significantly different than metaplasia or hyperplasia (p = 0.002) in the bronchial lesions.25 In another study OCT identified in situ morphologic changes associated with inflammation, squamous metaplasia, and tumor in 15 patients undergoing lung resections for cancer.26 OCT imaging enabled the characterization of the multilayered histology of the airways, with a maximum penetration depth of up to 2 to 3 mm and 10-μm spatial resolution.26,27
Therefore, there is significant clinical potential for confocal microscopy and OCT to be a sensitive and specific method for precancerous and cancerous lesions in the lung. It could be used for clinical diagnosis of the lesions, evaluation of the margins, and to monitor response to therapeutic treatment.
CONTRAST AGENTS FOR MICROENDOSCOPY
With the exception of reflectance confocal microscopy and OCT, most microendoscopes rely on the use of exogenous contrast agents to generate high-contrast images of biological tissues with good signal-to-noise ratio. Several FDA-approved contrast agents such as indocyanine green and fluorescein are in routine clinical use, which provide both absorption- and fluorescence-based contrast. In addition, many targeted contrast agents (tumor specific) are in development for optical molecular imaging.28,29,30 and 31 The use of these peptide-dye conjugate in optical imaging combines the specificity of ligand/receptor interaction with near-infrared (NIR) fluorescence detection and can be used in diagnosis of cancers. Activatable fluorescence imaging probes to sense enzyme activity have a potential to be used to image protease activity, as well as to study the therapeutic use of protease inhibitors in various diseases in patients.32,33 and 34 Molecular specific imaging using fluorescein isothiocyanate-labeled antibodies35 for detection of melanomas in mice and indocyanine green N-hydroxysulfosuccinimide ester (ICG-sulfo-OSu)-labeled antibodies36 in gastric carcinomas have been studied.
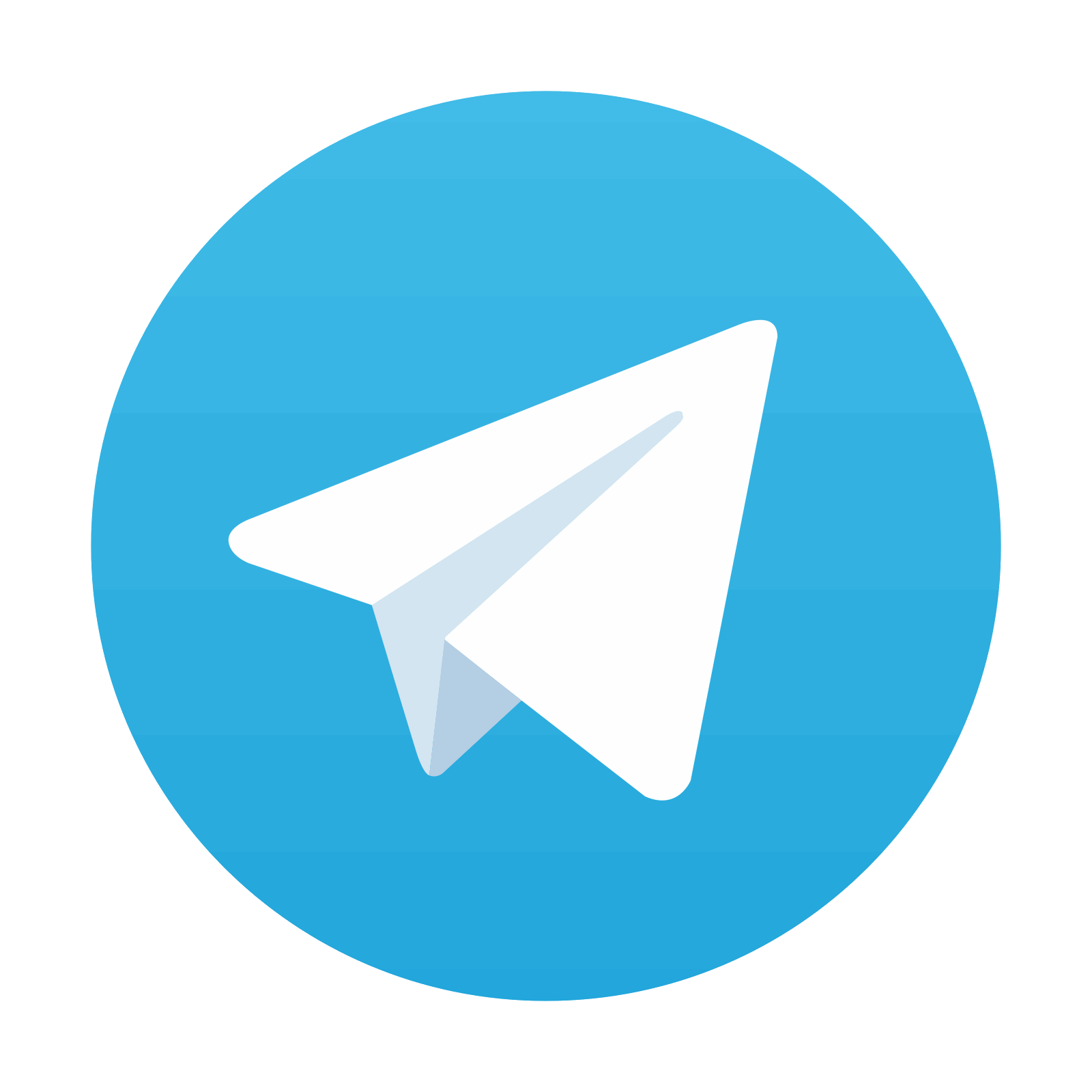
Stay updated, free articles. Join our Telegram channel

Full access? Get Clinical Tree
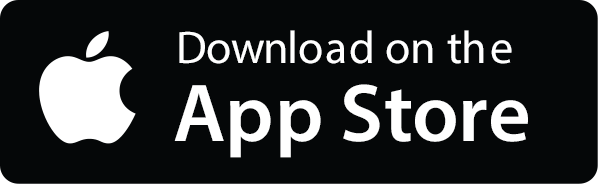
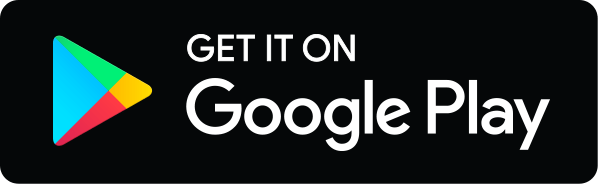