Patients with brain tumors are some of the most complex patients in the medical system, necessitating treatment teams of multiple subspecialists for optimal care. This article examines the roles of these subspecialists, with the goal of summarizing standard-of-care practices, recent therapeutic advances, and ongoing clinical investigations within each subspecialty.
Key points
- •
Patients with brain tumors have complex medical problems that require multidisciplinary subspecialty care.
- •
Symptoms will vary based on tumor histology and location, necessitating individualized treatment plans.
- •
Medical issues can arise from the brain tumor itself or the treatment plan.
Introduction
Despite only representing about 2% of all cancer diagnoses, patients with primary brain tumors are some of the most complex patients in the medical system. Medical personnel from different disciplines, including physicians, nurses, rehabilitation therapists, family counselors, and social workers, all contribute to the care of patients with brain tumors. This clinic focuses on the roles of different physician subspecialists in the care of these patients, with the goal of summarizing standard-of-care practices, recent therapeutic advances, and ongoing clinical investigations within each subspecialty. Each patient requires an individualized treatment plan developed by a team of these physicians in consultation with the patient, with different subspecialties playing a larger role at different points during treatment.
Introduction
Despite only representing about 2% of all cancer diagnoses, patients with primary brain tumors are some of the most complex patients in the medical system. Medical personnel from different disciplines, including physicians, nurses, rehabilitation therapists, family counselors, and social workers, all contribute to the care of patients with brain tumors. This clinic focuses on the roles of different physician subspecialists in the care of these patients, with the goal of summarizing standard-of-care practices, recent therapeutic advances, and ongoing clinical investigations within each subspecialty. Each patient requires an individualized treatment plan developed by a team of these physicians in consultation with the patient, with different subspecialties playing a larger role at different points during treatment.
The role of the neurosurgeon
The identification and resection of brain tumors is often the first intervention in the treatment of patients with both benign and malignant lesions. Interval monitoring, radiation-based treatments, and chemotherapy all offer promise in the treatment of certain subpopulations of patients; but for those suffering from a high burden of disease, a mass effect from tumor expansion, the need for definitive pathologic diagnosis, or for those with intolerable presenting symptoms, surgery is a critical first-line intervention.
Preoperative Assessment
Proper neurosurgical preoperative assessment takes into consideration both patient anatomy and overall health. Assuming a patient is healthy enough to undergo an operation, the neurosurgeon must create an operative plan that takes into consideration a tumor’s intrinsic anatomy; proximity to adjacent vital structures, such as cranial nerves, vessels, and motor and sensory areas; and the surgical approach with the greatest likelihood of maximizing the extent of resection and minimizing operative morbidities.
It is also important to acknowledge that goals of care may vary depending on the benign versus malignant nature of a tumor. For example, subtotal resection of a vestibular schwannoma may be enough to preserve hearing without compromising adjacent cranial nerves, such as the facial nerve, whereas the greater extent of resection for glioblastoma multiforme (GBM) has a significant effect on the length of survival. As opposed to high-grade tumors, in which the extent of resection becomes the most important predictor of survival, the treatment of benign tumors should be based on the amelioration of symptoms and comorbidities. In conjunction with other members of the treatment team, meaningful postoperative quality of life, prognosis, and the likelihood of morbidity should all be discussed at length with patients before any surgical intervention.
Biopsy and Resection
Because of the highly precise nature of tumor resection, the use of the operating microscope has greatly improved the ability of neurosurgeons to obtain more complete resections without compromising healthy brain structures. Additionally, the concomitant usage of neuro-navigation techniques, such as StealthStation (Medtronic, Minneapolis, MN) and VectorVision (Brainlab, Feldkirchen, Germany), allows for real-time correlation of intraoperative findings with magnetic resonance imaging (MRI), further facilitating resection of tumor tissues without extension beyond tumor margins.
Recently, evidence has shown a possible role for adjuvant measures that enhance the ability of neurosurgeons to obtain more extensive resection: intraoperative MRI and dyes that highlight neoplastic tissues for removal. In a randomized clinical trial, the use of intraoperative MRI led to higher rates of complete tumor resection without increasing the rate of operative neurologic complications, which is a finding that match those reported in prior nonrandomized trials. In a prospective, randomized controlled trial of patients assigned 5-aminolevulinic acid, a prodrug that leads to fluorescent accumulation in GBM tissues, the use of this dye led to more complete resections, in turn resulting in longer progression-free survival. Both of these findings make it clear that techniques to enhance the neurosurgeon’s ability to more completely resect GBM allows for better patient outcomes.
A subset of patients presents with tumors arising from or involving eloquent brain areas, including language centers, such as the Broca and Wernicke areas, the motor strip, and the sensory strip. The development of cortical mapping techniques, performed with both awake and asleep patients, has allowed for safer resection of such tumors that were previously deemed untreatable.
Practice with such techniques has allowed for better understanding of the inherent interpatient variability seen in language localization; consequently, smaller craniotomies can be performed with negative mapping strategies in which the cortex overlying tumors can be safely removed when it is shown that local depolarizing stimulation does not cause a loss of language ability. Resecting at least 1 cm away from stimulation-established language sites is safest ; in a large series of patients who underwent dominant hemisphere tumor resection with language mapping, less than 2% of patients suffered language deficits at 6 months, demonstrating the utility of such mapping techniques in avoiding postoperative deficits. Subcortical mapping and diffusion tensor imaging–based tractography have additional utility in mapping motor pathways, whereas techniques, such as functional MRI, positron emission tomography scanning, and magnetoencephalography, remain too imprecise at present to have major surgical utility.
Postoperative Complications
As a highly invasive intervention, neurosurgery for resection of brain tumors carries several important complications and risks, including infection, hematomas, hydrocephalus, pseudomeningocele, posterior fossa syndrome (mutism, emotional lability, difficulties initiating movement), and other focal neurologic deficits. Much of the complication profile following the resection of brain tumors arises from approach selection, tumor size, and operator skill.
Despite anecdotal reports that postoperative infection may, in fact, prolong survival, presumably because of the presence of local inflammation with antitumor effects, this remains controversial. Hemostasis remains an important intraoperative goal to reduce the onset of hematomas, whereas cerebrospinal fluid–related complications, such as hydrocephalus and pseudomeningocele, remain rare complications. Focal neurologic deficits can largely be avoided through the use of intraoperative mapping and a reluctance to resect tumor from vital adjacent structures, such as vessels and nerves.
Second Look Surgery
The use of second look surgery to rule out residual disease is not typically performed in the postoperative management of brain tumors. The close relationship between neurosurgeons, neuro-oncologists, and radiation oncologists typically ensures the close follow-up of patients, with interval scans being performed to document the absence or presence of either tumor recurrence or progression, which can necessitate repeat craniotomy for resection in select cases.
The role of the radiation oncologist
Radiation therapy (RT) is an integral and a highly effective component in the multidisciplinary management of patients with brain tumors. RT plans are designed and their implementation overseen by specially trained physicians, radiation oncologists. RT is a procedure whose outcome (balance between treatment benefits and side effects) depends on the skill of the treating physician. Because of this and because RT plans are specific to a treatment device, radiation treatment often cannot be easily transferred from one facility to another. This point is important for patients to understand before embarking on a treatment course so that treatment breaks and/or delays are avoided.
The choice of RT modality is determined by details of the clinical scenario, including patient factors (eg, performance status, severity of symptoms), tumor type and extent, tumor location in the brain (eg, proximity to eloquent brain areas or critical normal structures), and prior treatment history. RT comes in different forms, broadly divided into external and internal RT.
External Beam RT
External beam RT (EBRT), also known as teletherapy by its older name, is the most common form of RT. During EBRT, the patient is positioned on a flat table and immobilized in a treatment position using a thermoplastic mask to minimize movement during treatment. X rays are produced by a medical linear accelerator (linac) that can rotate and, in conjunction with the movable treatment table, can generate multiple beams that are projected at the tumor target from outside the patient. In general, multiple beams are used to treat a tumor target so that no one beam delivers all (or most) of the intended radiation; it is at the intersection of the beams where the intended radiation dose accumulates.
To calculate and control the distribution of the radiation dose, patients must complete a computed tomography (CT) simulation scan, a scan of patients in the simulated treatment position. A CT scan gives radiation oncologists information about the relative density of tissues within the patients’ body and, when imported into an RT planning computer, permits accurate calculation of radiation dose distribution, a process called planning. This form of EBRT, when coupled with the ability to mimic or conform to the tumor (target) shape, is called 3-dimensional conformal RT. A more sophisticated variant of this treatment technique, intensity-modulated RT (IMRT), can also vary or modulate the intensity of each beam and deliver doses that match complex tumor shapes. Furthermore, most modern linacs are equipped with a wide array of on-board imaging tools that permit real-time or near real-time monitoring of patient setup and/or tumor (or organ) motion. When these imaging techniques are used in conjunction with IMRT delivery, terms such as image-guided radiotherapy or image-guided adaptive radiotherapy can be used to describe them.
Most EBRT treatment courses extend over several weeks and allow radiation oncologists to treat tumors that often involve or invade critical brain structures (eg, anterior optic pathways) without injuring them. This selective injury of tumor and relative preservation of normal brain structures can be achieved by delivering small doses of radiation daily (5 days per week) for several weeks until the cumulative dose known to be effective for a particular tumor type is reached. The basis of fractionation is the concept that a daily dose is insufficient to cause lethal injury in cells with intact DNA damage repair mechanisms (normal cells) but adequate to cause tumor cell death where the intracellular repair machinery is aberrant. For these reasons, fractionated EBRT is considered to be a form of nonablative therapy.
Stereotactic Radiosurgery
Stereotactic radiosurgery (SRS) is a highly precise form of RT with an application that is limited to select cases when target obliteration is desired. Unlike EBRT, radiosurgery treatments are delivered with submillimeter accuracy to high doses given over 1 to 5 days. Because high doses of radiation are delivered, a high level of precision and accuracy is mandated. This level is achieved by the application of a rigid immobilization device, like a head frame, or by using robotics to automatically adjust for any deviations in the patient’s position that are seen on imaging. Both of these approaches rely on the use of a stereotactic coordinate system that is referenced to the immobilization device and/or treatment couch. For example, Gamma Knife (Elekta, Stockholm, Sweden) uses a rigid frame that is attached to the patient’s skull for immobilization and localization, whereas the CyberKnife (Accuray, Sunnyvale, California) and Novalis (Varian Medical Systems Inc, Palo Alto, CA and Brainlab, Feldkirchen, Germany) (a modified radiosurgical linac) rely on the use of more rigid thermoplastic masks and imaging to adjust for deviations in the patient’s position. Furthermore, SRS techniques use many beamlets, each of which does not transport much energy, that converge on the target. With this approach, the dose within the tumor is much higher than the dose in the surrounding normal brain tissue as a result of a sharp dose gradient (dose falloff) achieved by the multiple intersecting beams of radiation. Because the normal tissue interface is larger and the dose falloff is less rapid for larger lesions, increasing the exposure of the surrounding normal brain tissue, the prescribed dose is inversely proportional to the maximum tumor diameter. This physical phenomenon largely limits the application of SRS to lesions 3 cm or less in diameter.
SRS applications are generally limited to small tumor targets where obliterative, high doses can be delivered safely. The most common applications are brain metastases, benign brain tumors (eg, meningiomas, schwannomas), and select cases of recurrent malignant tumors.
Brachytherapy
Brachytherapy is a form of internal RT whereby the source of radiation is either permanently or temporarily implanted into tumor-bearing tissue. Depending on the application, different radioisotopes can be used. Most commonly, iodine-125 seeds are used for brain applications ; but increasingly, phosphorous-32–impregnated polymer film and other formulations are being used. The primary rationale for brachytherapy is that it can deliver highly localized radiation over the effective life of the radioisotope to a well-defined target, thereby preserving the surrounding normal tissue from dose. Brachytherapy applications in the brain are generally limited to cases of recurrent tumors, although different applications are actively being explored.
Radiation Sensitizers
Radiation sensitizers are drugs that enhance the sensitivity of tumor cells to radiation. Many compounds demonstrated promise in preclinical studies and have also been tested in humans with disappointing results. Halogenated pyrimidines and the nitroimidazoles have been most extensively studies; but more recently, hypoxic cell sensitizers, such as tirapazamine and RSR-13 (2-[4-[[(3,5-dimethylanilino)carbonyl]methyl]phenoxyl]-2-methylpropionic acid), have been evaluated. Other interesting compounds include motexafin gadolinium, which is a synthetic porphyrinlike agent that is thought to alter redox state of tumor cells. Topoisomerase inhibitors are another class of drugs that have both direct antitumor activity but additionally prevent DNA repair of radiation-induced DNA lesions. Other agents are actively being investigated.
Side Effects of RT
RT effects are concentrated on the tumor and surrounding involved tissue. For this reason, side effects of RT are primarily focal and dependent on the brain location being treated and often cannot be reliably distinguished from the effects of the tumor. The impact of treatment location, dose, target volume, and technique on the expected side-effect profile must always be considered when attempting to extrapolate one patient’s experience to another.
Acute side effects of EBRT include common effects (occurring in >50% of patients), such as partial alopecia, fatigue, scalp erythema, and less common effects (occurring in <20% of patients), such as otitis externa, impaired sense of taste, nausea, and headache. Early delayed and late side effects of RT may include tanning of the scalp, alopecia, hearing loss, neurocognitive decline, behavioral changes, somnolence syndrome, cataracts, and radiation necrosis. Neurocognitive effects of RT are a well-recognized phenomena; however, much of the available literature with few notable exceptions relies on the use of a Mini-Mental State Examination that is a poor outcome measure. Radiation Therapy Oncology Group and other groups have developed a brief, sensitive, repeatable, and highly standardized test battery to assess neurocognitive function. These tests have been shown to be sensitive to the impact of tumors and the neurotoxic effects of therapy.
Acute side effects from SRS include common complications, such as pin-site soreness and headache after frame removal in the case of Gamma Knife, as well as less frequent complications (occurring in <5% of patients), such as pin-site infection, short-term exacerbation of neurologic symptoms, and seizures. Later side effects, either early delayed or late, are uncommon (<5%–10% of patients) and include brain edema, radiation necrosis, and the worsening of preexisting neurologic deficits or the development of new ones.
It is important to again note that all forms of RT are procedures and, therefore, highly dependent on the design and implementation of the treatment plan by the treating radiation oncologist and his or her team. For these reasons, side-effect profiles can vary considerably.
The role of the neuro-oncologist
The role of the neuro-oncologist varies between patients with different histology. In addition, the physician-patient relationship can change over the course of treatment. For example, the natural history of low-grade gliomas is often characterized by an initial period of slow growth. During this time, patients undergo surveillance imaging and may intermittently take chemotherapy that maintains quality of life. At some point, however, these low-grade gliomas undergo malignant transformation and begin a period of rapid growth. Chemotherapy may become more intense, and patients may have more frequent office visits and hospitalizations with complications from these treatments. Death can occur many years after the initial diagnosis; by this time, patients have undergone multiple therapies.
Chemotherapy
Ultimately, treatment plans are individualized to the patient and differ based on tumor histology, location, and goals of care. For many tumors, chemotherapy is primarily given as adjuvant therapy to surgery and radiation. An exception is germinomas, which are highly chemosensitive and the outcome is not improved by surgical resection. Chemotherapy can also provide the primary means of disease control in some patients whose tumors are unresectable and radiation is not an option. In patients with optic pathway gliomas, low-dose metronomic chemotherapy can be used to delay radiation and the resulting endocrinopathies. In patients less than 3 years of age, neuro-oncologists often use chemotherapy to avoid radiation at such a young age because of the known neurocognitive sequelae. In adults, high-dose chemotherapy followed by stem cell transplant has generally not been shown to be effective and is not well tolerated.
Not all tumors respond to chemotherapy. Controversy exists about the role of the blood-brain barrier in chemotherapy delivery in patients with brain tumors. Brain tumors interrupt the blood-brain barrier, and chemotherapy delivery can be inconsistent. Yet many chemotherapy agents do cross the blood-brain barrier, such as nitrosoureas, platinum agents, and procarbazine. Intraventricular and intrathecal chemotherapy has been tried with mixed results. Chemotherapy-impregnated wafers, such as lomustine wafers, are directly implanted into the resection bed and used for adult patients with malignant gliomas.
Regardless of its delivery, traditional chemotherapy agents are not just toxic to tumor cells but also affect healthy tissue. Although patients are primarily concerned about hair loss, nausea, and fatigue, physicians manage many other side effects. This management can be challenging in patients with brain tumors because some of the chemotherapy agents can cause neurologic symptoms that may otherwise be mistaken for tumor progression. For example, vincristine can cause neuropathic pain and foot drop leading to gait abnormalities or other neuropathies that can present as weakness. Vincristine, carboplatin, and cisplatin can cause syndrome of inappropriate antidiuretic hormone, leading to hyponatremia and seizures. Because patients with brain tumors have a lower seizure threshold, they may have seizures with even mild hyponatremia. Knowledge about these side effects and appropriate monitoring is necessary.
Novel Therapies
The forefront of cancer therapy is the development of targeted therapies against cancer cells with the goal to increase efficacy with fewer side effects. The field is rapidly changing, and the following sections are intended as an introduction to some of these therapies in various stages of development.
The rapid rate of tumor proliferation requires the tumor to form new blood vessels to bring in the necessary nutrients for growth. The development of bevacizumab, a monoclonal antibody that inhibited vascular endothelial growth factor (VEGF), was the first antiangiogenic agent. It is generally well tolerated but has been associated with an increased risk of bleeding. A significant portion of patients with glioblastoma have a 3- to 6-month survival advantage with this treatment. Unfortunately, patients will inevitably progress and relapse. Bevacizumab is now a mainstay in glioblastoma therapy, and current studies are now evaluating the efficacy of bevacizumab in combination with other agents to try to prolong survival.
Although bevacizumab attacks a ligand likely to be important in multiple tumor types as well as some healthy tissues, more targeted therapy would be desirable. Drug development is now focused on drugs that work against specific mutations in particular tumor types or subtypes. Small molecule inhibitors targeting the sonic hedgehog pathway, a signaling pathway important in cell growth in a specific subset of medulloblastomas, are now entering the clinic. One of the first published cases using such an inhibitor reported on a remarkable tumor regression in a patient with metastatic medulloblastoma; however, the tumor eventually returned because of an additional mutation that developed in the tumor cells. Ongoing trials are currently testing if combination strategies with multiple agents can prevent the development of resistance and achieve sustained responses.
Despite significant advances in our understanding of the underlying molecular pathways leading to brain tumors, many patients with brain tumors will eventually progress through standard therapies and must decide if they would like to participate in clinical trials with investigational agents. Oncologists can inform patients about different trial options and consent them to study or refer them to an academic center with an open trial. However, patients who choose to participate must understand that there is limited knowledge about the efficacy and toxicity of many agents, particularly those still in early phase I trials. Furthermore, not all patients who desire to participate will be eligible. Clinical trials typically require a minimum performance score, and many trials exclude patients older than 70 years. Participation in clinical trials also varies by age, with a larger proportion of pediatric patients with brain tumors participating in at least one clinical trial during their treatment course. In a large survey of adults with malignant glioma, only 15% participated in a clinical trial. Part of this may be because of increasing cognitive decline that occurs with tumor progression, making study consent challenging.
Palliative Care
With the poor prognosis of brain tumors, many times all therapeutic options are exhausted, even investigational agents. Although palliative care specialization is growing in the United States, currently many neuro-oncologists and primary care physicians are often the main directors of palliative care for their patients. End-of-life and goals-of-care discussions should be started early in patients with brain tumors with grim prognoses before tumors may have progressed to the point that the patients can no longer express their wishes. A Dutch study found that approximately half of the patients with brain tumors were not deemed competent to participate in end-of-life decisions in the last weeks of life. However, the assessment of competence may be challenging. A study in patients with a recent diagnosis of malignant glioma (<6 months) found that more than 50% of patients already had markedly diminished understanding and reasoning of medical choices, although they were still able to express a treatment choice as well as controls.
Overall, palliative care of patients with brain tumors focuses on symptom relief. Pain control is often the top concern for patients and their families. Patients with brain tumors often have headaches, which can be managed with nonsteroidals and opioids. Neuropathic pain can be managed with gabapentin. Corticosteroids can reduce edema around the tumor and allow further symptom relief.
Other than pain, dysphagia is the most prevalent symptom with tumor progression, and the most common end-of-life decisions concern withholding hydration. Medications also have to be changed to rapidly dissolving sublingual forms or changed to transdermal or intravenous formulations.
It is important to note that family members and caregivers often have to deal with a rapidly changing neurocognitive status. Increasing neurologic impairment and dependency may increase mood fluctuations, adding further stress. Psychotherapy and support groups may be helpful in these situations.
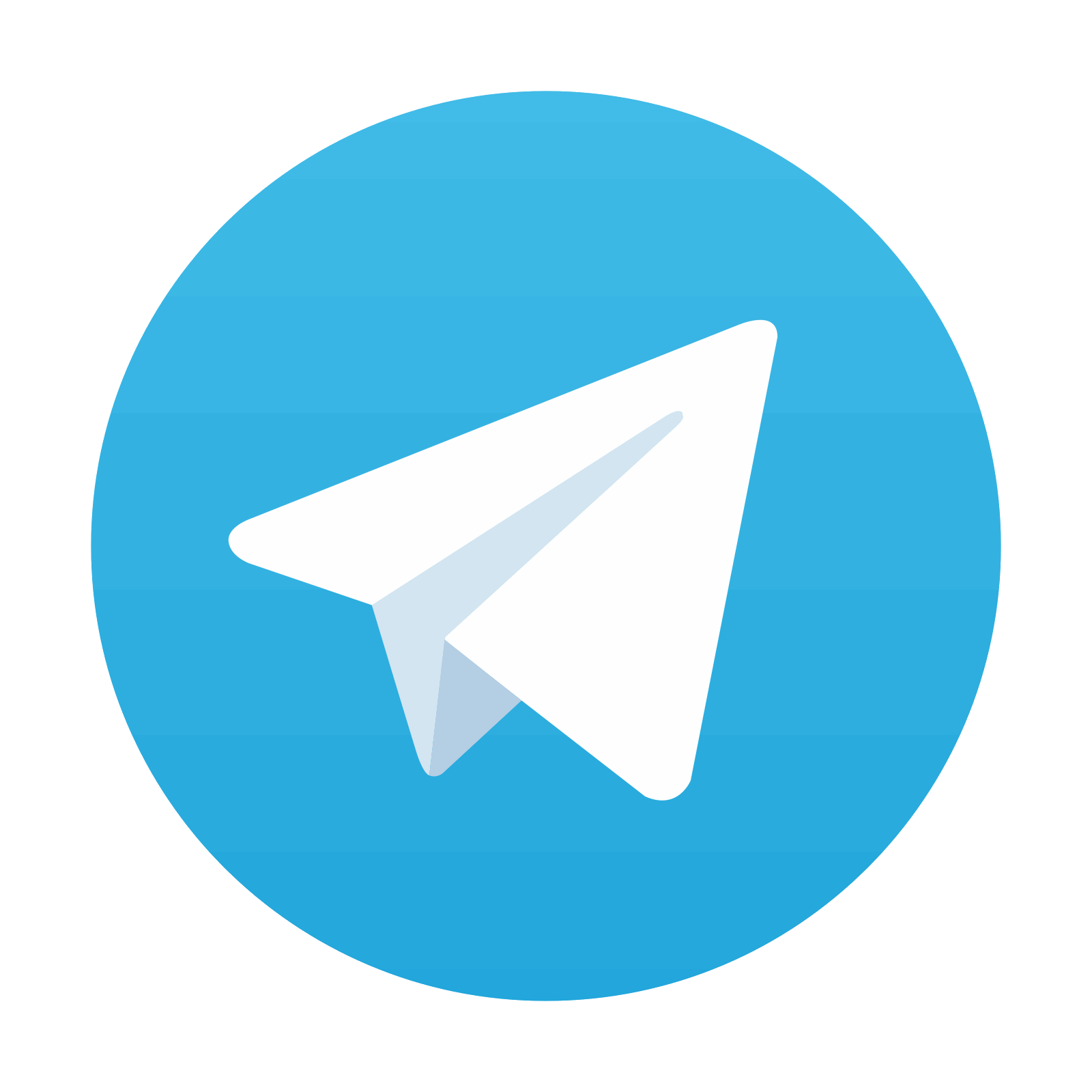
Stay updated, free articles. Join our Telegram channel

Full access? Get Clinical Tree
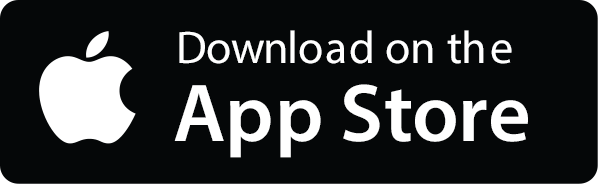
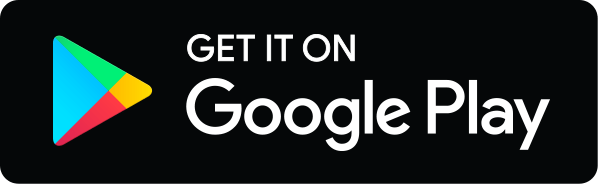