Fig. 2.1
Representation of all reported pheochromocytoma/paraganglioma susceptibility genes, specifying the year of publication, with special attention to the genes involved in the hereditary predisposition to the disease (opaque colored boxes). Genes recently reported or lacking further demonstration of their involvement are grouped in “other genes.” Question mark indicates that other unknown genes are pending to be uncovered
While initially it was thought that only 10 % of cases were caused by germline mutations, the discovery of mutations in several additional susceptibility genes during the last 15 years has brought the percentage of hereditary cases up to approximately 40 %. Genes such as VHL, RET, NF1, SDHA, SDHB, SDHC, SDHD, SDHAF2, MEN1, KIF1Bβ, EGLN1, EGLN2, TMEM127, MAX, EPAS1 (HIF2A), FH, and MDH2 are involved in PPGL susceptibility [1–3]. Recent findings have uncovered new candidate genes involved in chromatin remodeling [4], and the contribution of this genetic pathway to disease etiology requires further exploration.
Approximately 40 % of hereditary PPGLs develop primarily in the context of three familial tumor syndromes: von Hippel–Lindau disease (VHL), multiple endocrine neoplasia type 2 (MEN2), and familial PPGL. Patients diagnosed with multiple endocrine neoplasia type 1 (MEN1) and those with neurofibromatosis type 1 (NF1) can also develop PCC, but do so less frequently. PGLs present almost exclusively as part of familial PPGL, although there are very rare tumor syndromes in which these tumors are part of the clinical features (Table 2.1).
Table 2.1
Genetic and clinical characteristics of syndromes associated with the development of PCC/PGL
Syndrome | Gene | Inheritance | Biochemical phenotype | Associated pathology |
---|---|---|---|---|
MEN2 | RET | Autosomal dominant | E | MTC, PCC, infrequently PGL Type 2A: PHPT, cutaneous lichen amyloidosis Type 2B: Marfanoid body habitus, mucocutaneous neuromas, intestinal ganglioneuromatosis |
VHL | VHL | Autosomal dominant | NE, D | HB (CNS and retina), ccRCC, neuroendocrine pancreatic tumors, cysts and cystadenoma of the pancreas, kidney epididymis, or broad ligament, renal cysts, endolymphatic sac tumors, PCC, PGL, etc. |
PGL1 | SDHD | Autosomal dominant (paternal transmission) | NS or NE | PGL (head and neck, thoracic and abdominal), PCC, infrequently GIST, pituitary adenoma, infrequently ccRCC |
PGL3 | SDHC | Autosomal dominant | NS or NE | PGL (head and neck), infrequently PCC or GIST, infrequently pituitary adenoma |
PGL4 | SDHB | Autosomal dominant | NE, D | PGL, PCC, infrequently ccRCC, GIST, pituitary adenoma or PTC |
NF1 | NF1 | Autosomal dominant | E | Neurofibromas, cafe au lait spots, axillary freckling, optic gliomas, pigmented hamartomas of the iris, PCC |
MEN1 | MEN1 | Autosomal dominant | E | PHPT, pituitary adenoma, neuroendocrine gastroenteropancreatic tumors, PCC |
PGL2 | SDHAF2 | Autosomal dominant (paternal transmission) | NS | PGL (head and neck) |
PGL5/FPCC1 | TMEM127 | Autosomal dominant | E, NE | PCC, infrequently PGL (head and neck); ccRCC |
PGL6 | SDHA | Autosomal dominant | NE | PGL (head and neck, thoracic and abdominal), PCC; GIST, infrequently ccRCC and pituitary adenoma |
PGL7/FPCC2 | MAX | Autosomal dominant (paternal transmission) | NE, E | PCC (single, bilateral, multiple); up to 20 % of patients also develop PGL (thoracic and abdominal) |
PGL8 | FH | Autosomal dominant | NE | PGL (head and neck, thoracic and abdominal), PCC; cutaneous and uterine leiomyoma, type 2 papillary renal carcinoma |
KIF1B | KIF1B | Autosomal dominant | NE | PCC (bilateral); neuroblastoma |
PHD1/2 | PHD1/2 | Autosomal dominant | NE | PGL (multiple), PCC (bilateral); polycythemia |
MDH2 | MDH2 | Autosomal dominant | NE | PGL, PCC; no other associated tumors |
Pacak–Zhuang | EPAS1 | Somatic mosaicism | NE | Polycythemia, somatostatinoma, CNS HB, PGL (multiple), PCC (single) |
Between 10 and 20 % of patients with PPGL are diagnosed during childhood or adolescence [5–7], and PCC is the most frequently diagnosed endocrine tumor in children [8]. The proportion of pediatric patients with a germline mutation in one of the known susceptibility PPGL genes is higher than that found in adults. Recent studies have reported that up to 70–80 % of children with PCC are mutation carriers, regardless of their family history [5, 6, 9]. In addition, a proportion of patients with clinical characteristics indicative of hereditary disease (bilateral PCC, multiple PGLs, family history, and/or early-onset disease) do not carry mutations in any of the known genes, suggesting that other loci remain to be discovered (Fig. 2.1). The task of identifying new susceptibility genes is complicated by the fact that this disease can follow an autosomal dominant model, with or without preferential paternal transmission [10–14], and that post-zygotic somatic events have also been observed [4, 15]. It is probable that other models of inheritance, such as recessive, occur in some families with the disease. While uncovering this unexplained heritability of PPGL remains a substantial challenge, new approaches based on next-generation sequencing have begun to shed new light on the comprehensive biology of this tumor. It is paramount to correctly genetically classify each patient in order to be able to offer them the most appropriate clinical follow-up.
2.1.1 Syndromic PCC
Some patients develop PCC or PGL as part of a hereditary tumor syndrome; they present with other clinical signs that can help identify the gene most likely to be involved and therefore be used to prioritize genetic testing. Such patients have often developed other neoplasms or have a family history indicative of a strong genetic etiology, as is the case for PCC associated with multiple endocrine neoplasia type 2 (MEN2), von Hippel–Lindau (VHL), or neurofibromatosis type 1 (NF1) and to a lesser extent other syndromes such as Carney triad, Carney–Stratakis syndrome, and neoplasia endocrine multiple type 1 (MEN1). Patients with a germline mutation in RET have more often been previously diagnosed with medullary thyroid carcinoma (MTC), while those from MEN1 families tend to have had primary hyperparathyroidism (PHPT) and those from NF1 families cafe au lait spots. As described in detail below, one exception to this tendency to have particular comorbidities are patients with specific germline mutations in VHL, who tend to develop PCC as the sole manifestation of their disease.
2.1.1.1 MEN2-Associated PCC
MEN2 (OMIM 171400) has an estimated annual incidence of 5 per 10,000,000 persons and a prevalence of 1 in 30,000. MEN2 follows an autosomal dominant mode of inheritance. MEN2 patients can develop MTC (medullary thyroid carcinoma), PCC, and/or PHPT (primary hyperparathyroidism), the latter resulting from hyperplasia or from parathyroid adenomas. The syndrome is classified into three subtypes, MEN2A, MEN2B, and familial MTC (FMTC), each defined according to the combination of pathologies developed by the individuals affected (Table 2.1). MEN2A patients may develop all three pathologies. They are also more likely to develop “cutaneous lichen amyloidosis,” a pruritic skin lesion in the upper area of the back caused by the uncontrolled deposition of amyloid protein between the dermis and epidermis. In addition, these patients may occasionally develop Hirschsprung disease (HSCR). Patients are classified as MEN2B if they develop, in the absence of parathyroid disease, MTC; PCC; multiple mucocutaneous neuromas involving the lips, tongue, and eyelids; corneal nerve myelination; intestinal ganglioneuromas (hyperganglionic megacolon); or marfanoid habitus, including skeletal deformities and hypermobility of joints. Finally, families in which affected members have developed only MTC or C-cell hyperplasia (CCH) are considered to have the third subtype, FMTC, but only if more than ten members have MTC. A less conservative definition has been proposed by [16], based on the presence in at least four family members of MTC without other manifestations of MEN2A. Defining and distinguishing FMTC from MEN2A is challenging, and an exhaustive clinical follow-up of these families is required to rule out the presence of other tumors characteristic of MEN2, especially in older family members.
In the early 1990s, activating mutations in the rearranged during transfection (RET) proto-oncogene were identified as the genetic basis for MEN2A, MEN2B, and FMTC [17–20]. Since then, germline RET mutations have been identified in 98 % of patients with MEN2A, 95 % of patients with MEN2B, and 88 % of patients with FMTC [21].
RET is located on chromosomal band 10q11.2 and encodes a tyrosine kinase receptor that is mainly expressed in cells derived from the neural crest (C cells, parafollicular thyroid cells, and adrenal medulla cells, among others) and in urogenital system precursor cells [22]. The genetic testing of RET is relatively simple, since the mutations associated with the development of MEN2 are mainly located on exons 10, 11 and 13–16. Additional, less frequent mutations on exon 5 and 8 have been also reported in MEN2 patients. Sequencing of the entire coding region of RET is recommended only for those who meet clinical criteria for MEN2 but in whom initial sequencing of selected exons gives negative results [23] or if there is a discrepancy between the MEN2 phenotype and the expected genotype [24]. If a RET variant is detected at a noncanonical position, it is important to consult different databases for evidences that the variant may be a polymorphism with no clinical significance [25]. A sequence change in RET is considered to be a causative MEN2 mutation if it segregates with the clinical expression of disease within a family including at least two affected individuals having the MEN2 phenotype.
The established genotype–phenotype relationships for MEN2 syndrome are based on the classification of individual mutations according to their transforming ability, and therefore the expected aggressiveness with which the disease they cause will develop [24]. The impact of RET mutation testing on the management of MEN2 patients is without doubt one of the most robust examples of the utility of genetic diagnosis in personalizing clinical follow-up [24].
Approximately 50 % of MEN2 patients develop PCC in their lifetime, and the mean age at diagnosis is 35 years. A PCC is the first manifestation of MEN2 in only 12–15 % of cases, and so RET explains relatively few cases of non-syndromic disease (around 5 %), compared to other syndromes [26, 27]. RET mutations are very rarely found in patients diagnosed with PCC before age 20 [5–7]. Thus, RET is not a priority in the genetic testing of pediatric patients, although it should still be included in genetic diagnosis algorithms [5]. PCCs developed by RET mutation carriers are bilateral in 50–80 % of patients, they tend to be epinephrine-secreting tumors, and very few (no more than 5 %) are malignant.
2.1.1.2 VHL
VHL (OMIM 193300), with an incidence of 1 in 36,000 live births, is a dominantly inherited familial cancer syndrome caused by germline mutations in the VHL tumor suppressor gene [28, 29]. This gene encodes three gene products: a protein comprising 213 amino acids and two shorter isoforms, one produced by alternative splicing (excluding exon 2) and the other by alternative initiation. While the protein (pVHL) is involved in multiple processes, its best characterized role is the regulation of the proteasomal degradation of hypoxia-inducible factors (HIFs) [30]. Under normal oxygen tension, the α subunits of HIF (HIF-1α, HIF-2α, and HIF-3α) are hydroxylated by the dioxygenases PHD (PHD1, PHD2, and PHD3). The hydroxylated HIF-α are then targeted by pVHL for proteasomal degradation [31, 32]. Under hypoxia conditions, HIF-α is stabilized and binds to the HIF-β subunit to form an active transcription factor that regulates expression of a large repertory of genes involved in angiogenesis, cell survival, erythropoiesis, and tumor progression [30]. This explains the highly vascularized nature of the tumors associated with VHL syndrome [33].
The penetrance of causal mutations is age dependent, and the disease demonstrates marked phenotypic variability. Patients with VHL are at a higher risk of developing hemangioblastomas (HBs) of the retina and central nervous system (CNS), PCC and/or PGL, clear cell renal cell carcinoma (ccRCC), renal and pancreatic cysts (serous cystadenoma), neuroendocrine pancreas tumors, endolymphatic sac tumors, pancreatic serous cystadenomas, and papillary cystadenomas of the epididymis in men and of the broad ligament in women (Table 2.1) [27, 33, 34]. After identifying VHL in 1993, the phenotype associated with VHL gene mutations was expanded to include VHL disease, dominantly inherited familial PCC, and, in cases with particular mutations, autosomal recessive congenital polycythemia (also known as familial erythrocytosis-2; MIM# 263400) [35, 36]. It has been suggested that VHL accounts for approximately a third of patients with a CNS HBs, >50 % of patients with a retinal angioma, 1 % of patients with RCC, 50 % of patients with apparently isolated familial PCC, and 11 % of patients with an apparently sporadic PCC [37].
The disease is classified into four subtypes (1, 2A–2C) based on the clinical phenotype. VHL type 1 families have a relatively low risk of developing PCC, but may present with any of the other tumors associated with the disease. VHL type 2 is subdivided into three categories corresponding to a low (2A) or high risk (2B) of developing ccRCC or to a higher risk of PCC and PGLs as the only clinical sign of the disease (2C) (Table 2.2).
Table 2.2
Clinical classification of VHL patients
Clinical features | Subtype | ||||
---|---|---|---|---|---|
VHL-1A | VHL-1B | VHL-2A | VHL-2B | VHL-2C | |
HB (CNS, retina) | |||||
ccRCC | Low risk | High risk | |||
Cysts and cystadenoma of the pancreas | |||||
PCC |
Systematic characterization of germline VHL mutations has led to the identification of genotype–phenotype correlations such that germline mutations causing amino acid changes on the surface of pVHL are associated with a higher risk of PCC [34]. VHL germline deletions, mutations predicted to cause a truncated protein, and missense mutations that disrupt the structural integrity of the VHL protein are associated with a lower risk of PCC (and therefore associated with type 1 VHL syndrome). However, phenotypes related to VHL gene deletions appear to be influenced by the retention of some genes surrounding VHL. In particular, VHL deletions associated with the loss of the actin regulator HSPC300 gene (also known as BRICK1) are associated with protection against ccRCC [38–40]. These clinical findings led McNeill et al. (2009) to suggest that the subclassification of VHL syndrome should take into account not only clinical phenotype but also VHL mutation data [40] (Table 2.2).
PCCs/PGLs Associated with VHL
Approximately 20 % of patients with VHL develop PCC or PGL (sympathetic and parasympathetic), although the latter is much less frequent. Tumors are exclusively norepinephrine-secreting, related to a low, or no, expression of phenylethanolamine N-methyltransferase (PNMT), multifocal or bilateral in 43–45 % of cases and malignant in less than 5 % [41–43]. The median age at diagnosis of PCC/PGL is 29 years, which is lower than for other syndromes and particularly relevant for genetic testing since between 12 and 32 % of patients with PCC diagnosed during childhood are found to carry a germline mutation in VHL [44]. Also of note is that PCC (principally) or PGL (occasionally) is the first manifestation for 30–50 % of patients with VHL [41]. For these reasons, VHL mutation screening is essential in patients diagnosed before age 18. Furthermore, VHL has a high mutation rate (20–21 %) [45, 46], and so germline mutation testing of this gene is recommended specifically for patients with single tumors and non-syndromic cases. As we will review in this chapter, the role of VHL is also relevant in sporadic presentation, as a notable proportion of tumors develop as consequence of somatic VHL mutations [47].
The development of VHL-related tumors has been linked to the alteration of interactions between pVHL and other proteins with which it forms complexes, specifically pVHL-ElonginC-ElonginB complexes (CBCVHL). The most accepted hypothesis in this regard is that the development of PCC in the context of VHL is associated with a partial retention in the function of pVHL to assemble at least to some extent into CBCVHL [48, 49], which protects them from rapid intracellular degradation [50–52]. A hot spot in VHL that is associated with the development of PCC affects residue 167, located in the alpha domain. This domain has the role of interacting with other proteins, so that mutations giving rise to amino acid changes in this region do not result in loss of function of pVHL. The finding that 23 % (7/30) of patients with PCC who carry deleterious germline variants in VHL, but have no signs of either VHL or MEN2, have a mutation that affects this residue is consistent with this hypothesis [53]. On the basis of the above findings, it has been proposed that the measurement of change in pVHL stability could be used as an additional tool to understand the clinical features developed by a VHL patient [52]. Indeed, the use of this tool led to the identification of an association between ccRCC and missense mutations that significantly alter pVHL stability. A subsequent study classified these mutations as “surface” or “deep,” depending on the location of the affected residue in the protein structure, and found a clear difference between them in the associated risk of PCC [34].
2.1.1.3 Neurofibromatosis Type 1
Neurofibromatosis type 1 (NF1), formerly known as von Recklinghausen disease, is a common hereditary disease with an incidence of 1 per 2,500–3,300 newborns that primarily involves the skin and nervous system. The condition is usually diagnosed in early childhood, when cutaneous manifestations are apparent. It is characterized by the appearance of multiple neurofibromas, cafe au lait spots, freckling in the armpits and groin, iris hamartomas (Lisch nodules), bone lesions such as scoliosis, sphenoid dysplasia or pseudoarthrosis, macrocephaly, learning disorders, cognitive deficits, predisposition to optic and CNS glioma, and leukemia [27, 54]. Other malignancies occur less frequently in patients with NF1, including PCC, rhabdomyosarcoma, leukemia, and brain tumors other than optic gliomas [55]. Some features of neurofibromatosis 1 are present at birth, and others are age-related manifestations, which means that periodic monitoring is required to address ongoing health and developmental problems and to minimize the risk of serious medical complications [56].
The gene responsible for NF1, NF1 (17q11.2), encodes the protein neurofibromin, which is expressed primarily in the nervous system and has the role of suppressing cell proliferation by inactivating RAS proteins. Loss-of-function mutations in NF1 lead to the activation of RAS and the PI3K/AKT/mTOR pathway, which depends on RAS [54].
The detection of mutations in NF1 by DNA analysis has proven to be challenging because of the gene’s large size (it has 58 exons), the lack of mutation hot spots, and the existence of pseudogenes. NF1 mutations are predominantly truncating and often accompanied by loss of the wild-type allele in the tumor. Although molecular technology is now available to detect most mutations in NF1 [57], it is typically not required because in 95 % of cases a diagnosis of NF1 can be made by age 11 years on the basis of clinical findings alone. NF1 has one of the highest rates of spontaneous mutation of any gene in the human genome. This in part explains why between 30 and 50 % of patients have de novo mutations, which if they occur post-zygotically, can give rise to mosaic phenotypes [58]. Events of germline mosaicism are very rare in this condition [59].
PCCs Associated with NF1 Disease
An estimated 0.1–5.7 % of NF1 patients develop PCC, although this figure is 3.3–13 % based on autopsy studies. Therefore, NF1 is not a common diagnosis in PPGL patients [60]. These tumors are more prevalent among NF1 patients with hypertension (20–50 %) [61]. NF1-associated PCCs tend to develop at a later age (mean 41 years), can be unilateral or bilateral, and are rarely extra-adrenal and slightly more often malignant (up to 12 %) than those in VHL and MEN2 cases [62]. The earliest recorded age at diagnosis of PCC is 7 years, but given the low penetrance of NF1 mutations for this tumor development, screening of the gene is only recommended in cases of hypertension or symptoms suggestive of disease [27].
2.2 Non-syndromic PCC/PGL
In addition to the syndromic forms, many genes have been described over the last few years related to susceptibility to develop PCC or PGL as the only disease manifestation. Associations with other tumors have been reported, but only in a limited number of patients. We will outline the functions of the SDH genes, as well as FH, MDH2, TMEM127 and MAX, and detail the clinical manifestations associated with mutations in each of these. Other genes will be also reviewed.
2.2.1 Non-syndromic PCC/PGL Associated with Mutations in the SDH Genes, FH, and MDH2
2.2.1.1 SDH Gene Function
The connection between the SDH genes and the development of neuroendocrine tumors was established in 2000 when germline mutations in SDHD were first described in patients with familial PGL [14]. The SDH genes encode complex II of the mitochondrial respiratory chain, also known as succinate dehydrogenase (SDH), which plays a key role in both the electron transport chain and the oxidation of succinate to fumarate at the tricarboxylic acid (TCA) cycle. SDH is a heterotetramer composed of four proteins: two catalytic (SDHA and SDHB) and two structural (SDHC and SDHD). The latter are responsible for attaching the SDH complex to the inner mitochondrial wall (reviewed on [63]).
An associated protein, SDHAF2, is a highly conserved cofactor of flavin adenine dinucleotide (FAD) which is implicated in the flavination of SDHA and is essential for SDH function. SDHAF2 mutations have been reported to be associated with the development of PPGLs, confirming the importance of this complex for the disease [12, 13].
Heterozygous mutations in the SDHA, SDHB, SDHC, and SDHD genes cause complex II destabilization affecting the ability of cells to detect oxygen. SDH dysfunction results in the accumulation of succinate [64], its TCA cycle substrate, which acts as a competitive inhibitor of the 2-oxoglutarate (2-OG)-dependent HIF prolyl hydroxylases [65, 66]. This stabilizes HIF-alpha and, mediated by pVHL, activates genes that facilitate angiogenesis and anaerobic metabolism [66]. This link between mutations in the SDH genes and the HIF-1α pathway is also corroborated by results from tumor expression profiling studies of PPGLs [67]. Mutations in the SDH genes, both catalytic and structural, cause defects in the enzymatic activity of the complex, which lead to accumulation of succinate [68], along with the absence of SDHB [69]. Thus, negative SDHB immunostaining indicates the likely involvement of these genes in disease etiology; these findings represent robust tools that can be used to select patients for genetic testing, if paraffin-embedded tumor material is available.
Global DNA hypermethylation has been described as a hallmark of tumors with TCA cycle abnormalities resulting from SDH genes and FH and MDH2 mutations [1, 70, 71]. This CpG island methylator phenotype (CIMP) has revealed that succinate acts as an oncometabolite, inhibiting 2-oxoglutarate-dependent dioxygenases, such as hypoxia-inducible factor prolyl hydroxylases and histone and DNA demethylases.
Prognosis associated with high CIMP is cell-type dependent. For instance, in glioblastoma high CIMP is associated with a more favorable prognosis, whereas poor prognosis has been reported for neuroblastoma and PPGL [70, 72]. SDH gene-related, and particularly SDHB-related, PPGLs have a higher risk of progressing to metastatic disease [73]. It has been found that although all SDH gene-mutated tumors displayed CIMP, the level of hypermethylation is significantly higher, and the expression of target genes significantly lower, in SDHB-mutated tumors. As target genes include genes implicated in neuroendocrine differentiation and epithelial-to-mesenchymal transition (EMT), this could explain the particular metastasis-prone nature of SDHB-mutated tumors [70].
Loss-of-function mutations in the four SDH complex subunits and SDHAF2 have been demonstrated to cause PPGL, though the frequency of mutations and associated tumor types vary by genes. In addition to PPGL, SDHB and SDHD mutations have been associated with ccRCC [74, 75] and thyroid carcinoma [76, 77]. In addition, mutations in SDHB, SDHC, and SDHD can give rise to Carney–Stratakis syndrome [78], characterized by the dyad of PGL and gastrointestinal stromal tumors (GISTs). These findings revealed a novel molecular mechanism underlying the development of GISTs, which are usually related to gain-of-function mutations in KIT or PDGFRA [79, 80]. It has more recently been recognized that SDH gene mutations are associated with the development of pituitary adenomas (PA) ([81–83] and reviewed in [84]).
2.2.1.2 Mutations in the SDH/FH/MDH2 Genes: Genotype–Phenotype Relationship
Clinical Presentation Associated with Mutations in SDHD
The hereditary syndrome PGL1 (OMIM ID: 168000) is caused by mutations in the SDHD gene. The estimated penetrance of germline mutations in SDHD (11q23.1) is 86 % to age 50 years, and carriers normally present with multiple PGLs at a mean age of 35 years. SDHD carriers primarily develop head and neck PGL (84 % of cases), although up to 22 % also develop thoracic and abdominal PGL and 12–24 % PCC, the latter rarely being bilateral [76, 85, 86] (Table 2.1). Although PCCs and extra-adrenal PGLs are relatively rare in patients with SDHD germline mutations, it has been described that the type of mutation influences the phenotype. SDHD mutations predicted to result in an absent or unstable SDHD protein were associated with an increased risk of PCCs and PGLs, compared to missense mutations or in-frame deletions, which were not predicted to impair protein stability [76].
SDHD-related disease follows an autosomal dominant mode of inheritance, with preferential paternal transmission [87]. That is, a mutation carrier will only develop the disease if their mutation came from their father; if it came from their mother, they will not be affected, although they will still be able to pass on the mutation to their children. While this pattern of inheritance suggests the existence of maternal genomic imprinting of this gene, the observed bi-allelic expression of SDHD in different normal tissues and in neural crest-derived tumors does not support this hypothesis [88–90]. In order to explain this SDHD-linked parental effect, it has been proposed that the loss of the entire maternal copy of chromosome 11, a hallmark of SDHD-linked tumors, leads to the simultaneous deletion of the SDHD gene and an exclusively maternally expressed gene [91, 92].
Regardless of the mechanism underlying this preferential paternal transmission, the hereditary nature of disease is masked in families in which by chance the mutation has been transmitted from generation to generation only from mother to child. The disease skips generations and these can only be identified in genetic counseling centers that collect information from second- and third-degree relatives.
A key issue in clinical follow-up is the fact that 3–10 % of carriers of a germline mutation in SDHD develop metastasis [76, 77, 93–95]. In the case of pediatric patients, despite the possible lack of family history, it has been suggested that a diagnosis of at least one head and neck PGL is sufficient to justify genetic testing; in fact, 8–16 % of patients under age 20 years carry a germline alteration in SDHD [5, 6].
In relation to the development of other tumors, it should be noted that there has been some controversy around two variants in SDHD, p.H50R, and p.G12S. Both were initially reported to be associated with the development of Merkel cell carcinoma and familial CCH and even Cowden-like syndrome. However, they were subsequently classified as polymorphisms, present in several healthy populations (http://www.lovd.nl/3.0/home), and their associations with the proposed diseases have therefore been ruled out [96].
Even though the earliest reported age at diagnosis is 5 years, screening is recommended from the age of 10 years [27].
Clinical Presentation Associated with Mutations in SDHB
The PGL4 syndrome (OMIM ID: 115310) is due to mutations in the SDHB gene (1p36.13). Overall, the SDHB gene is the most commonly mutated of all the SDH-related genes [97]. An estimated 67 % of patients carrying mutations in SDHB develop primarily thoracic and abdominal PGL, 27 % develop head and neck PGL, and 17–29 % develop adrenal PCC, which is rarely bilateral [69, 85, 86].
Although, to date, a clear genotype–phenotype relationship does not exist for SDHB mutations, an association between SDHB missense mutations and an increased risk of head and neck PGL have been described, compared to truncating mutations [76]. Large deletions seem to lead to similar phenotypes and penetrance to those patients with point mutations. Several large germline founder deletions in SDHB have been reported in multiple unrelated subjects from the Netherlands [98] and Spain [86, 99]. In these populations, the proportion of carriers of these founder mutations could be sufficiently high for the testing of large deletion in SDHB to be the first step in genetic screening.
At least 40 % of SDHB mutation carriers develop metastatic disease [97]. Thus, it is widely accepted that the identification of a mutation in SDHB is a marker of poor prognosis and more close clinically monitoring of the patient is required.
Of all the known susceptibility genes for hereditary PCC/PGL, SDHB constitutes a paradigm of heterogeneity in and of itself. Mutations in this gene are usually associated with the presence at diagnosis of a single retroperitoneal tumor [100]. In fact, only 25–40 % of all carriers will ever develop a tumor [101, 102]. This explains why most patients have no family history of disease at the time of their diagnosis. This fact, along with the frequent appearance of a single tumor in affected individuals, makes it very difficult to identify potentially hereditary cases. Although the underlying cause of reduced penetrance is unknown, possible genetic explanations include inhibition of cell proliferation due to secondary loss of vital genetic material in the proximity of the remaining normal allele or that additional loss of chromosome 11 is required (Hensen model) [63]. For all these reasons, and principally because of the high risk of developing metastases, all the algorithms currently used to guide genetic diagnoses include the testing of SDHB in patients with PPGL.
Although the earliest reported age at diagnosis is 6 years, screening is recommended from age 5 years [27].
Clinical Presentation Associated with Mutations in SDHC
Mutations in the SDHC gene (1q23.3) cause the PGL3 syndrome (OMIM #605373) [103]. Since relatively few mutations in SDHC have been described worldwide, the associated clinical manifestations have not been clearly defined; nevertheless, it is known that mutation carriers tend to develop PGL (93 % parasympathetic and 7 % sympathetic) and infrequently PCC or GIST. Up to 23 % of affected individuals have multiple PGLs and 25 % have a family history, suggesting that mutations have incomplete penetrance [85, 86, 104]. Thus, the clinical features of SDHC-associated cases are similar to those found in patients with sporadic head and neck PGLs. Mutation carriers typically present with solitary head and neck PGLs and a very low tendency to be malignant [105]. The mediastinum is the second most common location for SDHC-related PGL (10 % of all tumors), occurring in up to 13 % of patients [106]. The mean age at diagnosis is 38 years [62], and very little is known about the involvement of this gene in pediatric disease.
Epigenetic inactivation of SDHC is a recently discovered phenomenon in GISTs and PPGLs from patients with Carney triad syndrome [107, 108]. This event has been reported as the genetic cause of a patient that presented with two abdominal PGLs and an adrenocortical adenoma, providing evidence that SDHC promoter methylation can cause PGLs due to SDHC inactivation [109].
Clinical Presentation Associated with Mutations in SDHA
Mutations in the SDHA gene (5p15.33) cause the rare familial PGL5 syndrome (OMIM #614165) [110]. Based on currently available information, SDHA (5p15.33) appears to contribute little to PCC/PGL. Korpershoek and colleagues reported that 3 % of their series of 198 patients with apparently sporadic PCC or PGL were found to carry mutations in SDHA [69]. These carriers developed PCC, head and neck PGL, or thoracic and abdominal PGL (Table 2.1), but rarely metastatic disease. Although it has been established through biochemical analysis that nonsense SDHA mutations are associated with disease, these same mutations have been found in unaffected population controls, suggesting that these mutations have very low penetrance; these findings add additional complexity to the genetic counseling offered to carriers, most of which will not develop clinical symptoms [63].
Nevertheless, SDHA should be considered in genetic testing for patients presenting with clinical evidence of familial PPGL who test negative for the other known susceptibility genes. As previously mentioned, mutations in any of the SDH genes have the effect of suppressing the enzymatic activity of complex II and a key indicator that this has occurred is negative immunostaining for SDHB. Furthermore, it is now known that mutations in SDHA also give rise to negative immunostaining for SDHA [69]. This relatively easily implemented clinical screening tool should be incorporated into molecular diagnostic protocols to ensure that appropriate mutation testing is carried out in the most efficient and cost-effective manner.
Clinical Presentation Associated with Mutations in SDHAF2
SDHAF2, also known as SDH5, was identified as the susceptibility gene for the PGL2 syndrome (OMIM ID: 601650) [13]. SDHAF2 (11q12.2) is similar to SDHD in that it has an autosomal dominant mode of inheritance, with a preferential paternal transmission. To date only head and neck PGLs have been reported in SDHAF2 mutation carriers, most diagnosed at an early age and all with a family history of the disease ([12] and references contain therein). Few distinct SDHAF2 mutations have been described [12, 111, 112]. While available data suggest that mutations in SDHAF2 do not explain a substantial portion of cases (<1 %), further studies in different populations are required to determine their relevance. Nevertheless, genetic testing of SDHAF2 should be offered to patients with head and neck PGLs with negative tumor staining for SDHB and who test negative for mutations in SDHD, SDHC, and SDHB. While currently too few data are available to draw clear conclusions, no affected mutation carriers developed PGL before age 20 years, suggesting that mutations are not relevant to the development of pediatric tumors.
FH: Clinical Presentation Associated with Mutations in FH
FH is the TCA cycle enzyme involved in the reversible hydration/dehydration of fumarate to malate. It is known that germline mutations in FH (1q43) predispose to leiomyomas and papillary RCC in an autosomal dominant hereditary syndrome named hereditary leiomyomatosis and renal cell cancer (HLRCC) [113]. Inactivation of FH leads to accumulation of its substrate, fumarate, and inhibits α-ketoglutarate-dependent HIF prolyl hydroxylases, leading to HIF activation [64, 66]. Other dioxygenases, including histone demethylases and the TET (ten–eleven translocation) family of 5-methylcytosine (5 mC) hydroxylases, are also inhibited by succinate and fumarate accumulation [114]. Very recently, Letouze et al. [70] identified a germline mutation in FH by whole-exome sequencing applied to blood and tumor DNA obtained from a 63-year-old female presenting with one PCC. The patient was selected to be sequenced because the tumor showed a methylome- and transcriptome-based profile very similar to that found in tumors carrying mutations in the SDH genes. The subsequent screening of almost 600 patients with PPGL but no mutations in the major susceptibility genes revealed that five carried pathogenic germline FH mutations, providing further evidence of the involvement of this gene in the development of PPGL [115]. Clinically, a metastatic phenotype and multiple tumors were significantly more frequent in patients with FH mutations than those without such mutations. Recently, as previously mentioned for succinate, fumarate has been reported as an epigenetic modifier that elicits epithelial-to-mesenchymal transition [164]. FH should thus be added to the list of PPGL susceptibility genes and should be considered in mutation screening, to assess the risk of metastatic disease.
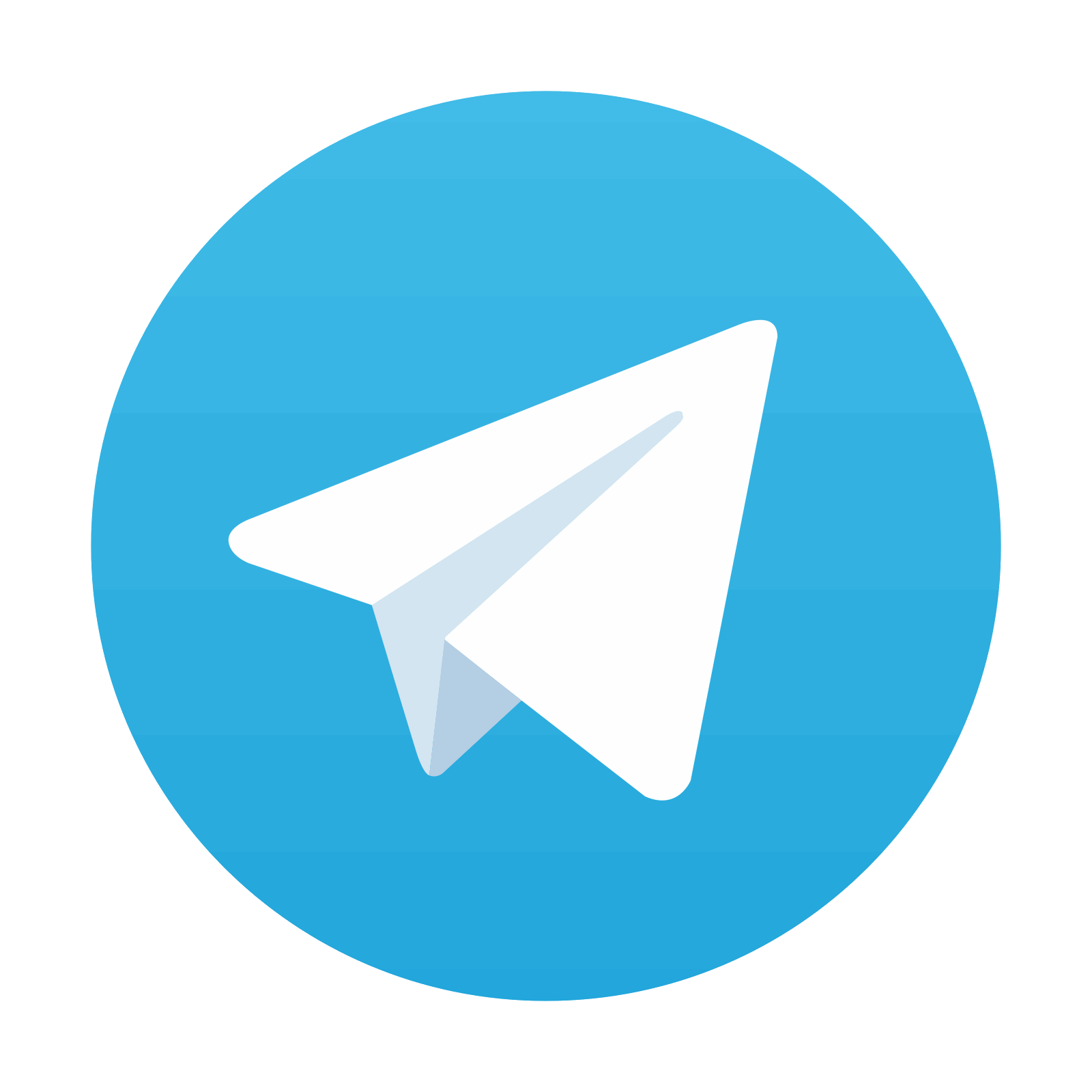
Stay updated, free articles. Join our Telegram channel

Full access? Get Clinical Tree
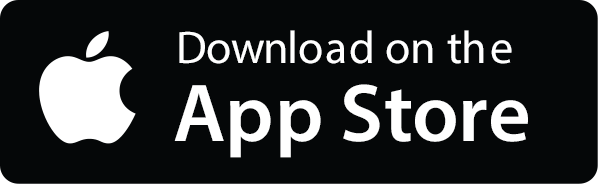
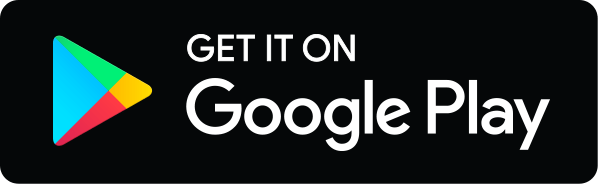
