Subtype
% of total cases
MTC
(%)
Pheo
(%)
HPT
(%)
Associated diseases
MEN2A
91
98
Classical
46
100
50
25
Hirschsprung’s disease
5
100
20
Hirschsprung’s disease
Cutaneous lichen amyloidosis
5
100
50
Cutaneous lichen amyloidosis
FMTC
35
95
0
0
MEN2B
9
100
50
0
Ganglioneuromatosis, marfanoid habitus
Based on a model that utilizes these genotype–phenotype correlations, RET mutations have been stratified into three risk levels (moderate, high, highest) concerning the aggressiveness of MTC [2]. This stratification system was reclassified recently [2]; the former four-level categories were now subdivided into three risk groups [3]. Genetic testing detects nearly 100 % of mutation carriers and is considered the standard of care for all first-degree relatives of patients with newly diagnosed MTC. Recommendations on the timing of prophylactic/early thyroidectomy and extent of surgery are based on classification of RET mutations into these three risk levels (Table 4.2). The main factors influencing survival are stage of disease at diagnosis, the different RET mutations, but also the postoperative calcitonin level.
Table 4.2
Risk groups of RET mutations based on age at manifestation, the aggressiveness of MTC and penetrance of pheochromocytoma or primary hyperparathyroidism
ATA risk levela | Moderate | High | Highest |
---|---|---|---|
RET mutation (common codon) | 533, 609, 611, 618, 620, 630, 631, 768, 790, 804, 891, 912 | 634, 883 | 918 |
MEN2 subtype | MEN2A/FMTC | MEN2A/MEN2B | MEN2B |
MTC aggressiveness | Moderate | Higher | Highest |
Recommended age for genetic testing | 5 years | 3 years | As soon as possible (first year of life) |
MTC age of onset | 5 years to young adults | Before the age of 5 years | First year of life |
Timing of early thyroidectomy | Age 5 or 10 years or when calcitonin rises | Before the age of 5 years | As soon as possible, first months of life |
Screening for Pheo | Start at 16 years, periodically | Start at 11 years, annually | Start at 11 years, annually |
Screening for HPT | Start at 16 years, periodically | Start at 11 years, annually | – |
4.2 Phenotype: Clinical Syndromes of MEN2
MEN2 (OMIM 171400) is an autosomal dominant tumor syndrome with an estimated prevalence of 1 per 30,000 in the general population. MEN2 syndrome occurs in two clinically distinct varieties with MTC as a common manifestation. Affected individuals initially develop primary C-cell hyperplasia (CCH) that progresses to invasive MTC. Only familial primary CCH is a preneoplastic lesion. Secondary CCH, which has been associated with chronic lymphocytic thyroiditis, hypergastrinemia, near other follicular cell-derived thyroid tumors, and even ageing, has a much lower, if any, potential for malignancy [4]. The two subtypes of MEN2 differ with respect to incidence, genetics, age of onset, association with other diseases, aggressiveness of MTC and prognosis [2, 3, 5] (Table 4.1).
4.2.1 MEN2A Syndrome
MEN2A syndrome is characterized by MTC in combination with pheochromocytoma and/or multiple tumors of the parathyroid glands in a single patient or the presence of two or more tumor types in multiple members of a single family. It is the most common form of all MEN2 syndromes, representing 55 % of cases [6]. The frequency of MTC is over 95 % among patients with MEN2A, while the frequencies of pheochromocytoma and multiple parathyroid gland hyperplasia are 40–50 % and 10–20 %, respectively [7, 8]. MTC is generally the first manifestation of MEN2A and presents when patients are between 5 and 25 years of age. MEN2 is further subdivided into four groups [2]: (i) classical MEN2A, which includes MTC with pheochromocytoma or hyperparathyroidism or both; (ii) MEN2A with Hirschsprung’s disease; (iii) MEN2A with cutaneous lichen amyloidosis; and (iv) familial MTC (FMTC). FMTC, formerly considered a distinct variant of MEN2A, affects families as well as individuals who have RET germline mutations and who mainly show MTC (and rarely pheochromocytoma). Nowadays, FMTC is viewed as a phenotypic continuum of MEN2A rather than a distinct disease with decreased penetrance of pheochromocytoma and primary hyperparathyroidism. In general, the clinical course of MTC in FMTC is more benign than that of MEN2A or MEN2B, and some FMTC subtypes have late onset or no clinically manifest disease. Usually FMTC carries a good prognosis [2, 9, 10].
4.2.2 MEN2B Syndrome
MEN2B syndrome is the most rare and aggressive form of MEN2 and accounts for 5–10 % of MEN2 cases. It is characterized by MTC, pheochromocytoma, the absence of hyperparathyroidism, visible physical stigmata such as raised bumps on the lips and tongue (due to cutaneous neuromas), ganglioneuromas of the intestine and marfanoid habitus with skeletal deformations and joint laxity. Patients with MEN2B typically have disease onset during the first year of life and have a more aggressive form of MTC with higher morbidity and mortality rates than do patients with MEN2A. Multivariate analysis suggests that the higher mortality rate of MEN2B reflects a more advanced tumor stage at presentation rather than a more aggressive tumor behaviour once established [3]. Patients with MEN2B often do not have a family history of the disease; in more than 50 % of cases, the syndrome is due to de novo germline RET mutation primarily paternal in origin, which reflects the role of RET in spermatogonial stem cell renewal [11].
4.2.3 Diagnostic Procedure
A family history is often inadequate for diagnosing familial disease; more thorough genetic and biochemical screening often reveals a family history of MTC in patients originally thought to have the sporadic form of the disease. About 1–7 % of apparently sporadic cases have identifiable germline RET mutations, including about 2–9 % with de novo germline mutations [3, 12]. Strong genotype–phenotype correlations are recognized between specific mutations and MEN2 disease phenotypes, and these correlations have proved to be valuable in predicting disease onset and prognosis, as well as in guiding therapeutic intervention and patient management [8]. Earlier identification of patients with MTC by RET mutation analysis has changed the presentation from clinical tumors to preclinical disease, resulting in a high cure rate for affected patients and a much better prognosis.
4.3 Genotype: RET Proto-Oncogene Structure, Function and Abnormalities
4.3.1 RET Receptor Structure and Function
The RET proto-oncogene is localized in the pericentromeric region on chromosome 10q11.2, has 21 exons and encodes a receptor tyrosine kinase that appears to transduce growth and differentiation signals in several developing tissues, including those derived from the neural crest [13, 14] (Fig. 4.1). The protein consists of a large extracellular segment with a cadherin (Ca2+-dependent cell adhesion)-like domain, which is important for stabilizing RET dimers and a cysteine-rich domain that is positioned near the cell membrane, which is crucial for protein conformation and ligand binding. RET protein has a single transmembrane domain and an intracellular segment with two tyrosine kinase subdomains, TK1 and TK2. It is activated by ligand-induced dimerization [14–17]. RET binds ligands of the glial-derived neurotrophic factor (GDNF) family in conjunction with a co-receptor, designated GDNF family receptor α (GFRα). Upon binding of the ligand–co-receptor complex, RET dimerization and autophosphorylation on intracellular tyrosine residues recruit adaptor and signalling proteins to stimulate multiple downstream pathways like the RAS–MAPK and PI3K–AKT pathways [15, 18]. These signals are important for many of the RET-mediated functions, including kidney and nervous system development, neuronal survival and differentiation, and maintenance of spermatogonial stem cells [19, 20]. In addition, phospholipase Cγ (PLCγ) binding to RET and the activation of protein kinase C (PKC) signalling are crucial for kidney morphogenesis and the migration of neuro-progenitors in the developing brain [21, 22]. The RET receptor is expressed at its highest levels in early embryogenesis and decreases to relatively low levels in normal adult tissues [23, 24]. RET is expressed in several neural and neuroendocrine cell lineages [25]. It is found in neuroendocrine-derived cell types in many organs, such as the thyroid C cells, the precursors of MTC, and adrenal chromaffin cells, precursors of pheochromocytomas. RET signalling also maintains the enteric nervous system, and loss of RET function in these neurons leads to Hirschsprung’s disease [26], in which neuroblasts fail to migrate to the developing gut and neurons and ganglia of the enteric nervous system fail to mature owing to a lack of RET signals [27] resulting in a aganglionosis of the colon. RET loss-of-function mutations have also been recognized in patients with congenital abnormalities of the kidney and urinary tract.
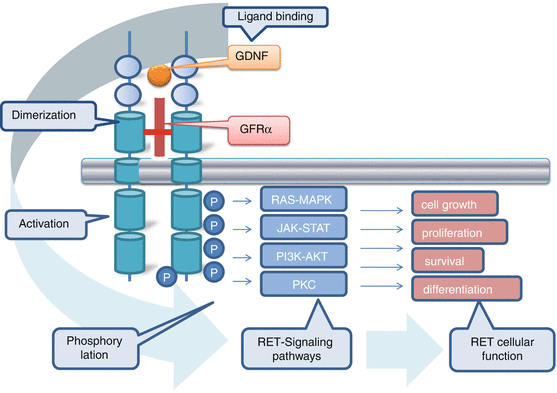
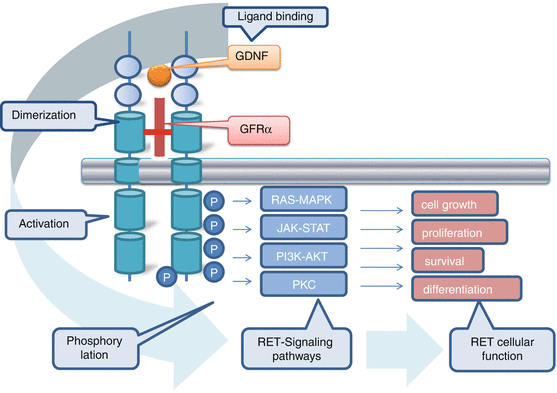
Fig. 4.1
RET receptor interaction and signal transduction network
RET oncogene mechanism implicates either through gain-of-function mutations that lead to constitutive activation or through aberrant expression or activation of wild-type receptors. Oncogenic RET mutations can be either activating point mutations within the full-length RET protein or genomic rearrangements that produce chimeric RET oncoproteins that are generally localized in the cytosol. While RET mutation has been primarily associated with thyroid cancer, somatic structural RET alterations or changes of its expression have been described in lung, breast and pancreatic cancers [28–30].
4.3.2 RET Mutations in MEN2
Hereditary MTC is caused by autosomal dominant gain-of-function mutations in the RET proto-oncogene. The MEN2 gene was localized by genetic linkage analysis in 1987 [31, 32]. Subsequently, point mutations of the RET proto-oncogene were identified in MEN2A and MEN2B in eight exons 5, 8, 10, 11, and 13–16 (Fig. 4.2). Analysis of RET in families with MEN2A revealed that nearly all of these families have germline mutations and that only those family members with the germline missense mutations have the disease. More than 80 different RET mutations have been associated with hereditary MTC [33].
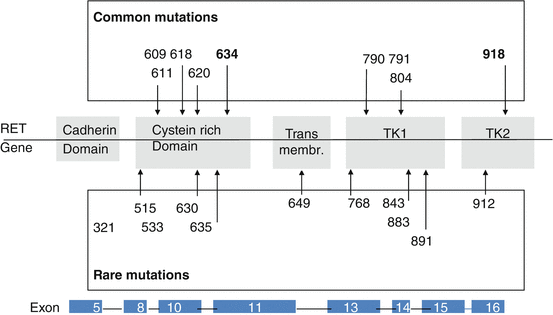
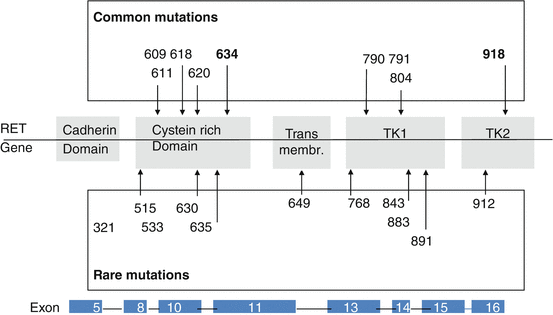
Fig. 4.2
Germline mutations in the RET proto-oncogene associated with multiple endocrine neoplasia type 2 and familial medullary thyroid carcinoma. Numbers indicate mutated codons of the RET gene
Mutations present in the extracellular domain (exons 8, 10, 11) are known to target highly conserved cysteine residues. Because these residues are normally involved in intramolecular disulphide bond formation, mutation generates an unpaired cysteine residue with free sulphydryl group. Mutant RET receptors in close proximity will then form intermolecular disulphide bonds, which function to stabilize dimerization even in the absence of the ligand and enhanced phosphorylation of intracellular substrates [34]. The most common mutations are substitutions of RET C634 which occur in patients with the classical MEN2A subtype and are associated with greater risks of pheochromocytoma and parathyroid hyperplasia. These classical MEN2A disease phenotypes are relatively rare in patients with other mutations of the extracellular domain (exons 10, 8, 5). In rare cases, a subgroup of these RET cysteine variants (affecting C609, C611, C618 and C620), termed ‘Janus mutations’, can confer both RET gain-of-function and loss-of-function phenotypes [35]. Although these mutations lead to constitutive RET activation and are associated with MEN2A phenotypes, the mutant proteins are poorly matured and are expressed at lower levels on the cell surface [36]. As a result, in tissues such as the enteric nervous system that require GDNF-mediated RET activation for appropriate formation [37], the reduced mature protein expression mimics loss-of-function RET mutations that occur in HSCR, and both MEN2 and HSCR phenotypes can co-occur in some families [8, 38].
Mutations of the intracellular tyrosine kinase domain of RET (codons 13–16) are known to target regions associated with the ATP-binding pocket, activation loop and substrate-binding pocket. Depending on their specific location, they induce structural changes and alter RET substrate specificity. This results in phosphorylation of alternative intracellular proteins [17]. Therefore, the mutated receptor no longer needs dimerization to become active.
The two specific amino acid substitutions in the RET kinase domain, M918T and A883F, are found exclusively in patients with the MEN2B phenotype. These residues flank the activation loop of the RET kinase and are associated with more complex functional outcomes, including protein conformational changes that decrease autoinhibition, increase kinase activity and ATP binding by tenfold, and might modify substrate recognition. Although M918T proteins are the most transforming RET mutants in vitro and are associated with the most severe disease phenotypes, the natural history of A883F mutants might be somewhat more indolent [10, 39].
Other mutations that affect the RET intracellular domain (residues E768, L790, Y791, V804 and S891) are predominantly associated with more indolent MEN2A phenotypes, later onset or a reduced penetrance of MTC and rarely pheochromocytoma [40], although there can be considerable interfamilial and intrafamilial variation associated with a single mutant. Mutations of Y791, which were originally detected in patients with FMTC and MEN2A, have also been identified in HSCR-only families and have been described in about 1 % of control populations, which is suggestive of a polymorphism rather than a pathological variant [41–43]. However, molecular studies have confirmed that the Y791F RET mutant proteins are constitutively activated and autophosphorylated as protein monomers and can induce downstream signalling and transformed phenotypes [44–47]. The tandem occurrence of Y791F with other RET substitutions (such as L790F or C634Y) seems to increase the aggressiveness and/or penetrance of MEN2 phenotypes [42, 48]. Although the molecular mechanisms that underlie this remain to be elucidated, the data are consistent with Y791F functioning on the one hand as a low-penetrance modifier or enhancer of other oncogenic mutations and, on the other hand, producing a receptor that is unable to respond to ligand activation or, therefore, to chemotactic signals, and this confers a risk of HSCR phenotypes. Other intracellular mutations have varying functional effects; for example, substitutions of V804 affect RET–ATP-binding [49], co-occurrence of the V804M mutation in cis with other kinase domain variants (Q781R, E805K, Y806C, or S904C) can result in additional conformational changes.
4.3.3 Rare and New RET Mutations: Polymorphisms
In newly detected germline RET sequence changes, it is mandatory to clarify if this ‘mutation’ is causative of MEN2 and segregates with the MEN2 disease symptoms within a family. The web-based ARUP online Scientific Resource RET database (http://www.arup.utah.edu/database/MEN2/MEN2_welcome.php) [33] uses the following classification definition: mutation, polymorphism and variant of unknown significance (VUS).
Mutation is defined as causative for the disease and segregates with the disease in (i) at least two affected family members in which at least one has MTC and the other one has a clinical feature of MEN2 or (ii) if at least three unrelated individuals with MTC have the same germline RET sequence variant [33].
Polymorphisms are benign germline RET sequence changes that are not causative for MEN2 like G691S, L679L, S836S, S904S and intron 14 c.2608-24G > A. These RET polymorphic sequence variants might have some implications as either low-penetrance risk alleles for RET-associated cancers or as genetic modifiers of oncogenic risk. A G691S substitution in the RET intracellular juxtamembrane region is overrepresented in sporadic MTC, pancreatic cancer and cutaneous and desmoplastic malignant melanomas [50–52]. This polymorphisms might also modify the age at which tumors develop in patients with MEN2A in association with other RET mutations [53, 54]. This is not confirmed by others: in a study with 150 patients with sporadic MTC carriers and noncarriers of the RET variants G691S, L767L, S836S and S904S appeared clinically and pathologically indistinguishable [55]. The functional mechanisms that underlie these phenotypic effects are not yet clear.
Variants of unknown significance (VUS) or uncertain are RET sequence changes in which there is not enough clinical evidence to indicate a causative role and further clinical studies are necessary to clarify if this sequence change is a mutation or a polymorphism. Prediction of disease association for novel mutations and uncertain gene variants may be performed using in silico and in vitro analysis. There is a positive correlation between the in silico risk score and in vitro focus formation units [45].
4.3.4 Somatic Mutations in MTC
Approximately 23–60 % of sporadic MTCs have an acquired somatic RET M918T mutation in tumor tissue. This mutation is identical to the germline mutation found in MEN2B [56, 57]. The prevalence of somatic M918T RET mutations varies depending on tumor size: small tumors (<1 cm) rarely have the mutation (11.3 %), while M918T is found in 58.8 % of patients with tumors >3 cm [58]. Patients with sporadic MTC with M918T have more aggressive tumor growth and a poor prognosis [56, 59, 60]. It is important to recognize that somatic mutations are heterogeneous. For instance, in the context of metastatic sporadic MTC, some metastatic foci have a codon 918 mutation, while the primary tumor or another focus can be devoid of this mutation [56]. RET does not seem to be the early initiator of tumor growth in sporadic MTC; rather, RET is activated later in oncogenesis as a driver of tumor growth, and other genes must play a significant role in MTC onset. It was recently discovered that 18–80 % of sporadic MTCs lacking somatic RET mutations have somatic mutations in KRAS, HRAS or, rarely, in NRAS [61]. No other common genetic mutation has been detected in subsequent exome sequencing studies of MTCs [62].
4.4 Genotype–Phenotype Correlation in MEN2
Clear associations have been documented between specific RET mutations (MEN2 genotype) and the age of onset and aggressiveness of MTC and the presence or absence of other endocrine neoplasms (MEN2 phenotype), such as pheochromocytoma or hyperparathyroidism. Approximately 98 % of index patients with MEN2 have an identifiable RET mutation [63]. Some overlap exists between RET mutations and the resulting clinical subtype of MEN2. This genotype–phenotype correlation between specific mutations and MEN2 disease phenotype has been proved to be valuable in predicting disease onset and prognosis, as well as in guiding therapeutic intervention and patient management [2, 38].
Initially, the frequency of RET codon 634 mutations in patients with ‘classical’ MEN2A reached 85 %; mutations in codons 609, 611, 618 and 620 accounted for an additional 10–15 % of cases [8] (Fig. 4.2). In recent years, there has been a change in the spectrum of RET mutations detected in patients with hereditary MTC. Specifically, there has been a shift from the ‘classical’ mutation at codon 634 in exon 11 to more cases with mutations in exons 13–15 and less aggressive disease [6]. Our recent analysis of the RET proto-oncogene in patients with hereditary MTC provides evidence for this change in the spectrum of detected mutations. Exon 13–15 mutations, so-called rare mutations, were diagnosed in 39 % of families, exon 10 and 11 mutations in 54 % and exon 16 mutations in 6 % (Fig. 4.3). This change in the frequency of diagnosed mutations in MEN2A families from high-risk mutations to the so-called mild mutations in codons 13, 14 and 15 with late manifested and slowly growing MTC and rarely developing pheochromocytoma and hyperparathyroidism has changed the clinical presentation with a better prognosis of hereditary MTC. The reasons underlying this change in mutation spectrum detected may include the routine RET diagnostics in all patients diagnosed with MTC, the discovery of hereditary cases in apparently sporadic (4–7 %) cases [12], and the extension of the analyses to include mutations other than the known “hot spots” [42]. In addition, there is a distinct distribution of RET mutations in different parts of the world that depend on the genetic background of the local population, which may also affect the detection rates of specific RET mutations.