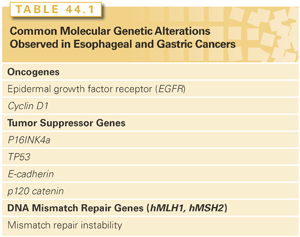
Epidermal Growth Factor Receptor
The epidermal growth factor receptor (EGFR) family of receptor tyrosine kinases stimulates a number of signal transduction cascades (e.g., Ras/Raf/MEK/ERK, PI3K/AKT) that regulate diverse cellular processes, such as proliferation, differentiation, survival, migration, and adhesion. These signaling pathways are important in normal cellular homeostasis, but aberrant activation of the EGFR members is crucial in esophageal carcinogenesis. This family of receptors comprises EGFR (also referred to as erbB1, erbB2, erbB3, and erbB4). The receptors have the ability to homo- or heterodimerize on engagement with one of several ligands: transforming growth factor α (TGF-α), EGF, amphiregulin, heparin-binding EGF-like growth factor, betacellulin, and epiregulin. The tyrosine phosphorylation of homo- or heterodimers of EGFRs creates docking sites for signaling proteins or adapter proteins. EGFR is commonly overexpressed in early-stage esophageal cancer, and overexpression correlates with a poor prognosis.6–9 EGFR overexpression is typically due to increased engagement with ligands and decreased turnover. However, the mutation of a tyrosine residue in the cytoplasmic domain is rare. Increased expression of TGF-α and EGF has been detected in BE, EAC, and ESCC.10–14 EGFR overexpression may predict a poor response to chemoradiotherapy15,16 and is associated with decreased survival in patients with squamous cell carcinoma.15 Furthermore, EGFR overexpression was associated with recurrent disease and diminished overall survival in patients undergoing an esophagectomy for ESCC.16,17
Cyclin D1 and p16INK4a
Cyclins, cyclin-dependent kinases (CDK), and cyclin-dependent kinase inhibitors (CDKi [such as p15, p16, p21, and p27]) regulate the mammalian cell cycle. During the G1 phase, the cyclin D1 oncogene complexes with either CDK4 or CDK6 to phosphorylate the retinoblastoma (pRb) tumor suppressor protein and, in so doing, relieves the negative regulatory effect of pRb, allowing the E2F family of transcription factors to propel the cell cycle toward the G1/S phase transition.18 Toward the late G1 phase, cyclin E complexes with CDKs to phosphorylate p107, which is related to pRb, and liberates more E2F members to navigate the cell cycle into S phase. As with EGFR, cyclin D1 overexpression is found in premalignant lesions, such as esophageal squamous dysplasia or BE, and the majority of early-stage ESCC or EAC.19,20 Additionally, cyclin D1 overexpression correlates with poor outcomes and survival as well as poor response to chemotherapy.21,22
Although cyclin D1 overexpression accounts for cyclin D1 dysregulation, other mechanisms include mutations in cyclin D1 and mutations in Fbx4, which is the E3 ligase for cyclin D1, thereby preventing degradation of cyclin D1 in the cytoplasm and reimportation into the nucleus, where it exerts its oncogenic effects.23
In a similar vein, p16INK4a is an early genetic alteration, via promoter hypermethylation, point mutation, or allelic deletion, in BE and EAC, but interestingly, is a late event in ESCC. Loss of heterozygosity of 9p21, the locus for both p16 and p15, has been demonstrated with high frequency in both dysplastic Barrett epithelium and Barrett adenocarcinoma (90% and more than 80% of cases, respectively).24,25 Promoter hypermethylation, which prevents tumor suppressor function by blocking transcription, has been documented and correlates with the degree of dysplasia in BE. It is present in up to 75% of specimens with high-grade dysplasia and is found in almost 50% of patients with adenocarcinoma of the esophagus.24,26 Point mutations of p16 in ESCC have been found, and promoter hypermethylation has been noted in up to 50% of these tumors.27,28 An Rb gene mutation is not found in either type of esophageal neoplasm, but allelic loss of 13q, where the locus of the Rb gene resides, is found in up to 50% of patients with Barrett adenocarcinoma and squamous cell carcinoma.29 This can correlate with diminished or loss of pRb protein in BE with dysplasia, EAC, and ESCC.30
TP53 Tumor Suppressor Gene
TP53 is the most commonly known mutated gene in human cancer.31–33 TP53 is a tumor suppressor that interrupts the G1 phase to evaluate and permit the repair of damaged DNA, which may arise from environmental exposure (e.g., irradiation, ultraviolet light) or cellular stress.34 In the face of irreparable damage, p53 induces apoptosis. The p53 transcription factor binds DNA to activate or suppress a large repertoire of target genes.35 TP53 mutations induce the loss of cell-cycle checkpoints and promote genomic instability. The majority of TP53 mutations occur in the DNA-binding region, and more than 80% of them are missense mutations resulting in loss of wild-type p53 function.36 Wild-type TPp53 has a short half-life and is difficult to detect by immunohistochemistry; a mutation in p53 results in the stabilization of the protein and allows for easier detection by immunohistochemistry.
The detection of the mutated p53 protein by immunohistochemistry has been demonstrated with increasing frequency during histologic progression from BE (5%) through dysplasia (65% to 75%) to frank adenocarcinoma (up to 90%).37–39 Thus, the p53 mutation or loss of heterozygosity appears early in BE and EAC. Both mutant p53 protein detected by immunohistochemistry and specific p53 gene mutations detected by genomic sequencing have been identified in 40% to 75% of patients with ESCC.40–42 The presence of a p53 point mutation correlates with a response to induction chemoradiotherapy and predicted survival after esophagectomy in patients with either ESCC or EAC.43
Telomerase Activation
The maintenance of telomere length allows DNA replication to be sustained indefinitely. The aberrant expression of telomerase has been observed in most esophageal cancers examined to date.43 Morales et al.44 observed increased telomerase expression in 100% of adenocarcinoma and BE cases with high-grade dysplasia. Telomerase activation is important, but alternative mechanisms to maintain the length of telomeres may operate in these cancers as well.45
Tumor Invasion and Metastasis
The loss of cell–cell adhesion can lead to both invasion and metastases. Alterations in expression of E-cadherin, a cell–cell adhesion molecule, or its associated catenins (e.g., p120 catenin or p120ctn) disrupt cell–cell interactions, which results in the potential for tumor progression.46 Reduced expression of E-cadherin has been correlated with progression from BE, to dysplasia, and finally to adenocarcinoma, and is also observed in ESCC.47,48
Models of Esphageal Squamous Cell Cancer and Esophageal Adenocarcinoma
Advances in the diagnosis and therapy of esophageal neoplasms will ultimately be fostered through cell lines, xenotransplantation mouse models, surgically based rodent models, and genetically engineered mouse models. There is a vast array of cell lines established from primary and metastatic human esophageal cancers that allow the perturbation of gene expression to gauge effects on cellular behavior. Recently, organotypic (three-dimensional) cell culture models, which mimic human tissue, have revealed that the combination of EGFR and mutant p53 results in the transformation of human esophageal epithelial cells immortalized with human telomerase reverse transcriptase (hTERT).49
In transgenic mice in which cyclin D1 is targeted to the esophagus, esophagi reveal evidence of dysplasia that evolves into squamous cell cancer on crossbreeding the mice with p53 loss.50 More recently, the conditional knockout of p120ctn in the esophagi of mice results in invasive ESCC.51 Rodents have also been treated with nitrosamines to yield esophageal papillomas and ESCC.52
A classic rodent model involves a total gastrectomy followed by an esophagojejunostomy.53 This creates a milieu whereby the esophagus is exposed to high concentrations of bile (nonacid reflux) with the development of BE and EAC. Recently, two genetically engineered mouse models have changed our views of BE and EAC. The targeted expression of the interleukin-1β, a cytokine, to the mouse esophagi, results in esophageal and gastroesophageal inflammation, the development of BE, and long latency to EAC.54 However, the time for EAC development is hastened by adding bile acid to drinking water consumed by the mice or by crossbreeding these mice with mice null for the p16INK4a allele.54 Another model involves the global knockout of p63, which is important in squamous stem cells and progenitor cells, revealing Barrettlike cells in the postnatal period when the mice die from other causes.55 In each of these two models, the cells that give rise to the Barrett cells or Barrettlike cells migrate from the gastric–squamous forestomach junction to the junction–distal esophagus.54,55
Functional Genomics
The underlying fate switch between ESCC and EAC may also be influenced by the expression and function of lineage-specific transcriptional factors as demonstrated through functional genomics. To that end, SOX2, found to be part of an amplicon on chromosome 3q26.33 in human ESCC, fosters growth of these cancers. This may have implications in the therapy of human ESCC.56 Similarly, GATA6, a known transcriptional factor, has been reported to be overexpressed in EAC.57 Exome and whole-genome sequencing of EAC has revealed >20 genes that are mutated significantly, some of which include newly identified chromatin-modifying factors.58
MOLECULAR BIOLOGY OF GASTRIC CANCER
The most common type of gastric cancer is adenocarcinoma, of which there are two subtypes: intestinal and diffuse. They are distinguished by different anatomic locations within the stomach, variable clinical outcomes, and different pathogenesis. The intestinal type of sporadic gastric adenocarcinoma has a hallmark progression from normal gastric epithelium, to chronic atrophic gastritis (typically due to Helicobacter pylori infection), to intestinal metaplasia (which has some overlapping but also different features than intestinal metaplasia of BE), to dysplasia, to cancer (Fig. 44.2). Diffuse-type gastric adenocarcinoma is even more invasive and aggressive in its behavior, has overlap with lobular-type breast cancer, and may be highlighted by E-cadherin loss.

Inherited Susceptibility
Case-control studies have observed consistent—up to threefold—increases in risk for gastric cancer among relatives of patients with gastric cancer.59,60 Studies of monozygotic twins have even shown a slight trend toward increased concordance of gastric cancers compared with dizygotic twins.61,62 Large families with an autosomal dominant, highly penetrant inherited predisposition for the development of gastric cancer are rare. However, early-onset diffuse gastric cancers have been described and linked to the E-cadherin/CDH1 locus on chromosome 16q and associated with mutations in this gene.63 This seminal finding has been confirmed in other studies with gastric cancers at a relatively high (67% to 83%) penetrant rate.64–67 Thus, E-cadherin mutation testing should be considered in the appropriate clinical setting. In fact, prophylactic gastrectomy should be strongly considered in families with germ-line E-cadherin mutation even without gross mucosal abnormalities by endoscopic examination of the stomach.68 Recently, germ-line alpha-catenin mutations have been described as well in these families.69
Lynch syndrome, or hereditary nonpolyposis colon cancer, involves germ-line mutations of DNA mismatch repair genes.70 Gastric adenocarcinoma may be observed in some families with Lynch syndrome. Gastric cancers have also been noted to occur in patients with familial adenomatous polyposis and Peutz-Jeghers syndrome.70
Role of Helicobacter pylori
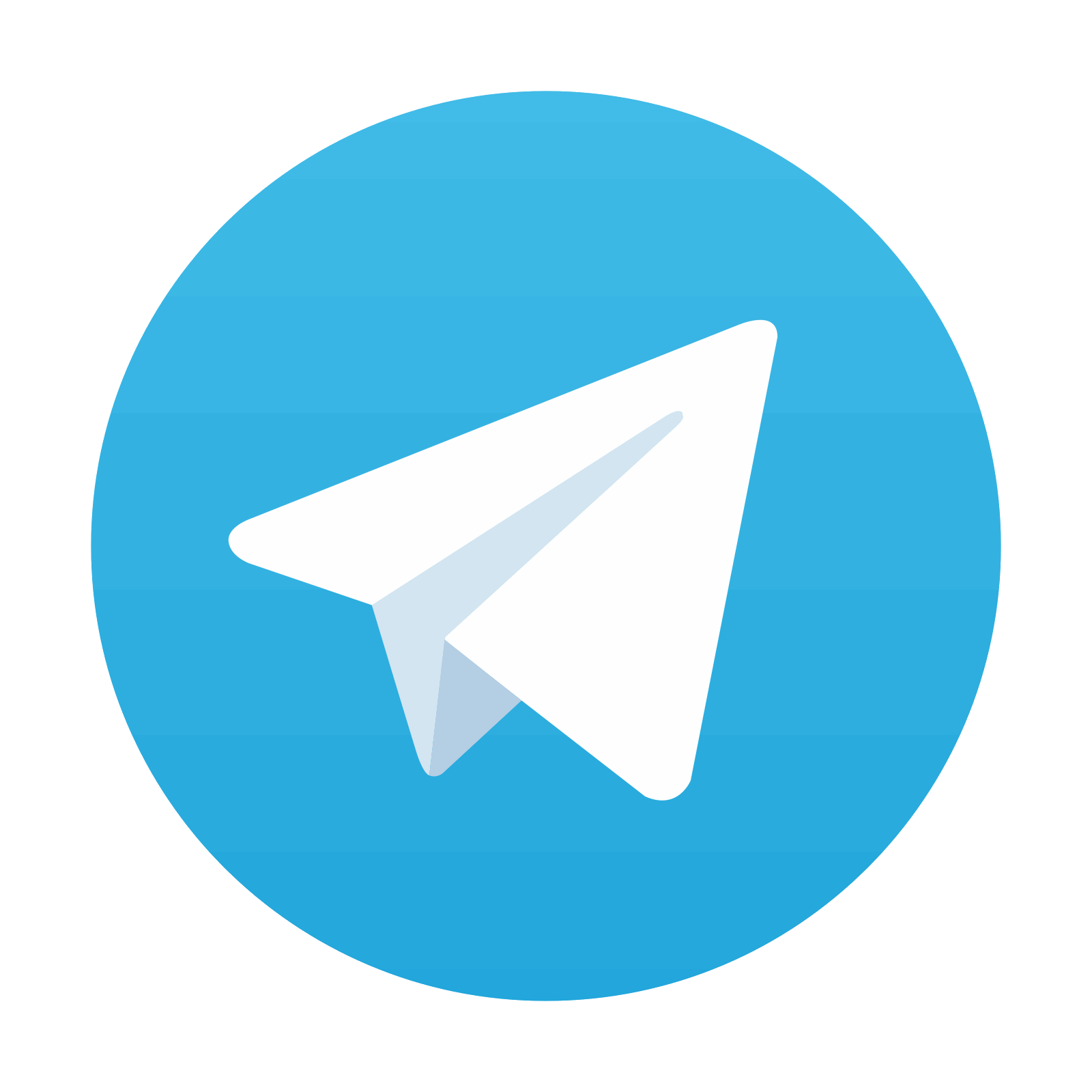
Stay updated, free articles. Join our Telegram channel

Full access? Get Clinical Tree
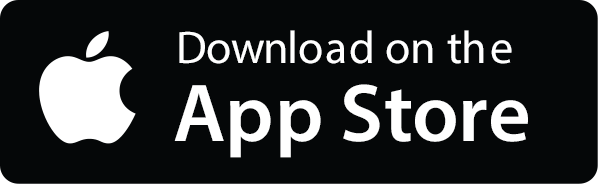
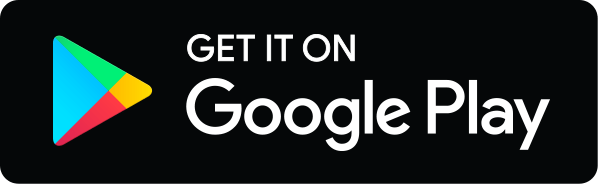