© Springer Science+Business Media New York 2017
William B. Coleman and Gregory J. Tsongalis (eds.)The Molecular Basis of Human Cancer10.1007/978-1-59745-458-2_2929. Molecular Basis of Skin Carcinogenesis
(1)
Phototherapy Unit, S. Gallicano Dermatological Institute, IRCCS, Rome, Italy
(2)
Immunology Department, The University of Texas MD Anderson Cancer Center, Houston, TX, USA
Keywords
ApoptosisDNA damagePhotocarcinogenesisSkin cancerTumor suppressor genesUV29.1 Introduction
The incidence of skin cancer exceeds the incidence of all other human cancers combined [1]. Epidemiologic, clinical, and biologic studies indicate that solar ultraviolet (UV) radiation is the major etiologic agent in skin cancer development. Wavelengths in the UVB range of the solar spectrum (290–320 nm) are absorbed by the skin producing erythema, burns, immunosuppression, DNA mutations, and non-melanoma skin cancers (NMSC) [2].
Multiple genetic alterations have been shown to have a direct correlation with cancer development. The majority of these mutations can be found within three categories of genes: proto-oncogenes, tumor suppressor genes, or DNA repair genes. A mutation in one of these groups or any combination can cooperate to induce a neoplastic condition. The proto-oncogenes act as crucial growth regulators in normal cell division, while the tumor suppressor genes act as negative growth regulators [3].
The p53 tumor suppressor gene is involved in the cell cycle arrest and activation of programmed cell death. Mutations in the p53 gene have been detected in 50 % of all human cancers and in almost all skin carcinomas [4]. The majority of carcinomas have missense mutations that produce a full-length protein with altered function. Often the other allele is lost resulting in loss of heterozygosity (LOH) which is particularly high (40–80 %) in carcinomas of the colon, lung, and bladder [5]. Several studies have shown that the p53 tumor suppressor gene is susceptible to UV-induced mutations and plays a critical role in the induction of NMSC.
In squamous (SCC) and basal (BCC) cell carcinomas of the skin the frequency of LOH is much lower with a higher proportion of both p53 alleles being independently mutated [6]. Mouse skin models have shown that standard chemical initiation/promotion protocols results in LOH, whereas repeated carcinogen experiments (like UV exposure) results in independent mutations on both p53 alleles [7]. Upon repeated exposure to UV radiation, DNA lesions in the p53 gene are transformed into mutations, mainly C → T or CC → TT transitions at dipyrimidine sites (UV signature mutations), thereby initiating the molecular process of skin carcinogenesis [8]. Thousands of p53 mutant cell clones are found in sun-exposed skin that appears quite normal. The frequency of UV signature mutations in the p53 gene is high in precancerous lesions and reaches 50–90 % in human BCCs and SCCs and 100 % in murine UV induced skin tumors [9, 10].
In addition to the disrupted p53-dependent mechanism, development of NMSC also may involve dysregulation of the Rb-controlled pathway of the cell cycle progression including inactivation of the CDKN2A tumor suppressor gene in the 9p21 region [11].
29.2 Acute Effects of UV Radiation
29.2.1 UV-Induced DNA Damage
Irradiation of DNA or cells with ultraviolet light induces the formation of several types of mutagenic DNA lesions. The most frequent lesions induced by UVB radiation are the cis-syn cyclobutane pyrimidine dimers (CPDs) and the pyrimidine pyrimidone photoproducts (PPs) [12, 13]. Several minor photoproducts such as purine dimers and pyrimidine mono-adducts are also formed [14].
CPDs are formed between the 5,6 bonds of any two adjacent pyrimidine bases. PPs are characterized by a stable bond between position 6 and 4 of the neighboring pyrimidines and appear to form preferentially at 5′-TC and 5′-CC sequences. They are formed at levels considerably lower than those of CPDs [15, 16]. These unique lesions in the DNA give rise to unique mutations in the p53 tumor suppressor gene. Consequently UVB induces mostly C → T and CC → TT transitions at the dipyrimidine sequence which are therefore called UV signature mutations [17].
The genotoxicity of UVA has most commonly been linked to endogenous photosynthesizers causing reactive oxygen species mediated induction of DNA damage [18, 19]. More recently it has been reported that UVA can induce CPDs in rodent cells. The poor absorbance of UVA by DNA itself favors the idea that type I (direct reaction) or type II (indirect through reaction of the excited photosensitizer molecule with oxygen) photosensitization reactions occurring within cells are primarily responsible for UVA induced DNA damage [20]. In addition, UVA may produce H2O2 through activation of flavin containing oxidases or through release of free iron in the irradiated cells from the iron storage protein ferritin [21, 22]. UVA has been shown to induce 8-oxo-7,8-dihydro-2′-deoxyguanosine (8-oxodG), a typical oxidative DNA lesion, as well as generate DNA strand breaks in a variety of experimental systems [ 23, 24].
29.2.2 Induction of p53 Tumor Suppressor Protein
p53 codes for a 53 kDa phosphoprotein involved in gene transcription and control of the cell cycle by coordinating transcriptional control of regulatory genes. Human p53 is a highly conserved 11 exon gene that is located on the short arm of chromosome 17 that is about 20 kb in size. p53 protein forms tetramers through interactions between recognize specific binding sites on target genes and stimulate their activation. Mutant forms of p53 rarely exhibit mutations in the DNA binding domain [25, 26].
Normally there is little p53 protein in the cell, but numerous studies have shown that in response to UV damage, high levels of p53 protein are induced. This has been demonstrated in cell culture, mouse epidermis, and human tissue [27]. With high levels of p53 protein, there is a G1 arrest, allowing the cellular repair pathway to remove DNA lesions before DNA synthesis and mitosis and an increase in apoptosis. Therefore p53 aids in the DNA repair or the elimination of cells that have excessive DNA damage [3, 25]. p53 is stabilized as early as 6 h after UV exposure reaching a peak at 12 h. Nelson and Katzan found the DNA lesions induced by UV radiation were pyrimidine dimers and that p53 was induced when these lesions were accompanied by excision repair-associated DNA strand breaks [28].
UV radiation induces the activating phosphorylation of the p53 protein at multiple serine residues, including Ser15, Ser20, Ser33, Ser37, Ser46, and Ser392. It has been also shown the involvement of the ATM, ATR, p38, ERK1/2, and JNK-1 mitogen activated protein (MAP) kinases in the phosphorylation of various p53 serine residues in response to UV radiation [29–31].
29.2.3 Cell Cycle Arrest and DNA Repair
Accumulation of activated p53 protein induces a cell cycle arrest at G1 phase which allows the repair of DNA damage before is replication in the S phase.
The p21Waf1/CIP1 gene encodes an inhibitor of cyclin dependent kinase (CDK) whose induction is associated with the expression of wild-type p53. p21 inactivates the CDK cyclin complex by forming a complex of CDK2, cyclin A, PCNA, and p21 leading the cell into G1 arrest. Formation of these complexes leads to the accumulation of hypophosphorylated retinoblastoma protein (Rb) causing the release of the E2F transcription factor which is necessary for DNA synthesis [32, 33].
The tumor suppressor activity of p53 is in turn inhibited by the Mdm2 protein, which targets p53 for rapid degradation. The gene encoding Mdm2 is itself activated by p53 thus providing a negative autoregulatory loop. If normal DNA repair is not achieved, cells proliferate abnormally which can lead to carcinogenesis [34].
The induction of DNA damage appears to play a central role in photocarcinogenesis and efficient removal of DNA lesions by cellular repair processes appears to be a critical step in the prevention of tumor formation. Interaction of UV with DNA can give rise to different forms of DNA damage which is normally removed by nucleotide excision repair (NER). The most relevant DNA damage generated after UV irradiation is the formation of CPDs. These lesions are primarily repaired by NER which removes bulky DNA damage in two distinct sub-pathways [35]. Damage existing in actively transcribed genes is removed by a quick mechanism called transcription-coupled repair (TCR) [36]. Damage prevailing in other parts of the genome is removed with a slower mechanism by the global genome repair (GGR) [37]. Defects in both sub-pathways of NER can lead to three distinct diseases: Xeroderma pigmentosum (XP), Cockayne syndrome (CS), and trichothiodystrophy (TTD). These diseases provide important model systems for investigating aspects of DNA repair since they are all deficient in NER but in different sub-pathways.
p53 directly affects NER by inducing the transcriptional activation of several downstream genes. Cells lacking functional p53 are defective in removing DNA photoproducts which are normally repaired by NER complexes [38].
29.2.4 Apoptosis
Keraratinocytes respond to UV-induced DNA damages by growth arrest or induction of the programmed cell death—apoptosis. p53 plays a major role in the control of both of these processes. In fact these two p53-dependent mechanisms are believed to maintain the integrity of the genome and to protect cells from the accumulation of mutations. Consequently, induction of apoptosis of keratinocytes by UV radiation is a protective phenomenon relevant in limiting the survival of cells with irreparable DNA damage [41].
p53-induced apoptosis involves several mechanisms and among them the bcl-2 gene family seems to play a crucial role. The bcl-2 gene family is composed of the pro-apoptotic gene group (Bax, Bad, Bak, Bcl-x s , Bik, Bim, and Mtd/Bok) and the anti-apoptotic gene group (Bcl-2, Bcl-w, Bcl-x L , and Mcl-1). Bax and Bcl-2 proteins are homologous but they have opposing effects on apoptosis and they may form heterodimers in cells [42]. Thus their interaction is critical to the ability of Bcl-2 to block cell death. p53 induces apoptosis by disrupting the balance between these proteins, upregulating the Bax gene, and downregulating the Bcl-2 gene [43].
Another p53 induced apoptotic mechanism is through the expression of Fas (APO-1/CD95) and its ligand. Fas receptor is a target for transcriptional activation by p53 and a target for p53-mediated cytoplasmic redistribution to the cell surface [44]. The Fas-Fas ligand interaction results in the cleavage of pro-caspase 8 and release of cytochrome c from mitochondria, followed by activation of the Apaf-1-caspase 9-caspase 3 pathway which results in apoptosis [45]. Hill et al. in 1999 demonstrated that Fas-Fas ligand interactions are essential for the induction of sunburn cells in UV-irradiated mouse skin and the elimination of aberrant cells following skin exposure to UV radiation. UVB irradiation induced the rapid overexpression of Fas and Fas ligand in murine skin. The formation of sunburn cells was found to depend on Fas ligand expression since their formation was significantly reduced in Fas ligand-deficient mice [46].
29.3 Chronic Effects of UV Radiation
29.3.1 Mutations in p53 Tumor Suppressor Gene
Following chronic UV exposure, mistakes associated with DNA repair and replication can result into mutations in the p53 gene, especially C → T and CC → TT transitions at dipyrimidine sites, considered as UV molecular signature. The p53 mutation in keratinocytes is probably an initiating event in UV skin carcinogenesis [8].
As UV signature mutations in the p53 gene are already present in benign precursor lesions of squamous cell carcinomas (AK), they appear to be an early step in the UV carcinogenesis. In the experiments with hairless mice, microscopic clusters of epidermal cells overexpressing mutant p53 occur long before skin carcinomas become visible [47]. Such clusters are also found in sun-exposed human skin [48]. Most of human NMSC found to bear mutations in the p53 gene. Brash et al. in 1991 showed p53 mutations in 58 % of human SCCs analyzed (3/24 showed CC → TT transition and 5/24 had C → T base change) [49]. In a recent study, Bolshakov et al. analyzed 342 human NMSC and found p53 mutations in 28/80 aggressive SCCs and in 28/56 non aggressive SCCs. About 71 % of the detected p53 mutations were UV signature mutations [50].
Experiments to determine the timing of p53 mutation in relation to skin cancer development have been performed in the mouse model of photocarcinogenesis because this model is easily referable to UV irradiation and sample collection at various time points during the carcinogenesis protocol. Ananthaswamy et al. have shown that p53 mutations in mouse skin arise very early during UV carcinogenesis by using a very sensitive mutation-specific PCR technique for the early detection of p53 mutations in UVB irradiated C3H mice. They were able to detect p53 mutations at 4th week of UV irradiation and the frequency of p53 mutations increased progressively and reached 50 % at 12th week of chronic UV exposure. The real p53 mutations frequency observed in this study is probably higher than the one described because only tandem CC → TT mutations were analyzed [51]. In addition to C3H mice, Ouhtit et al. used SKH-hr1 mice to determine the timing of p53 mutations during UV carcinogenesis. Interestingly, p53 mutations were detected as early as 1st week of chronic UV irradiation and the mutation frequency reached 80–90 % by 4–8 weeks [52]. Both the early appearance and the high frequency of p53 mutations in UV irradiated SKH-hr1 mice skin can be explained with the fact that either C → T or CC → TT mutations were analyzed in this study.
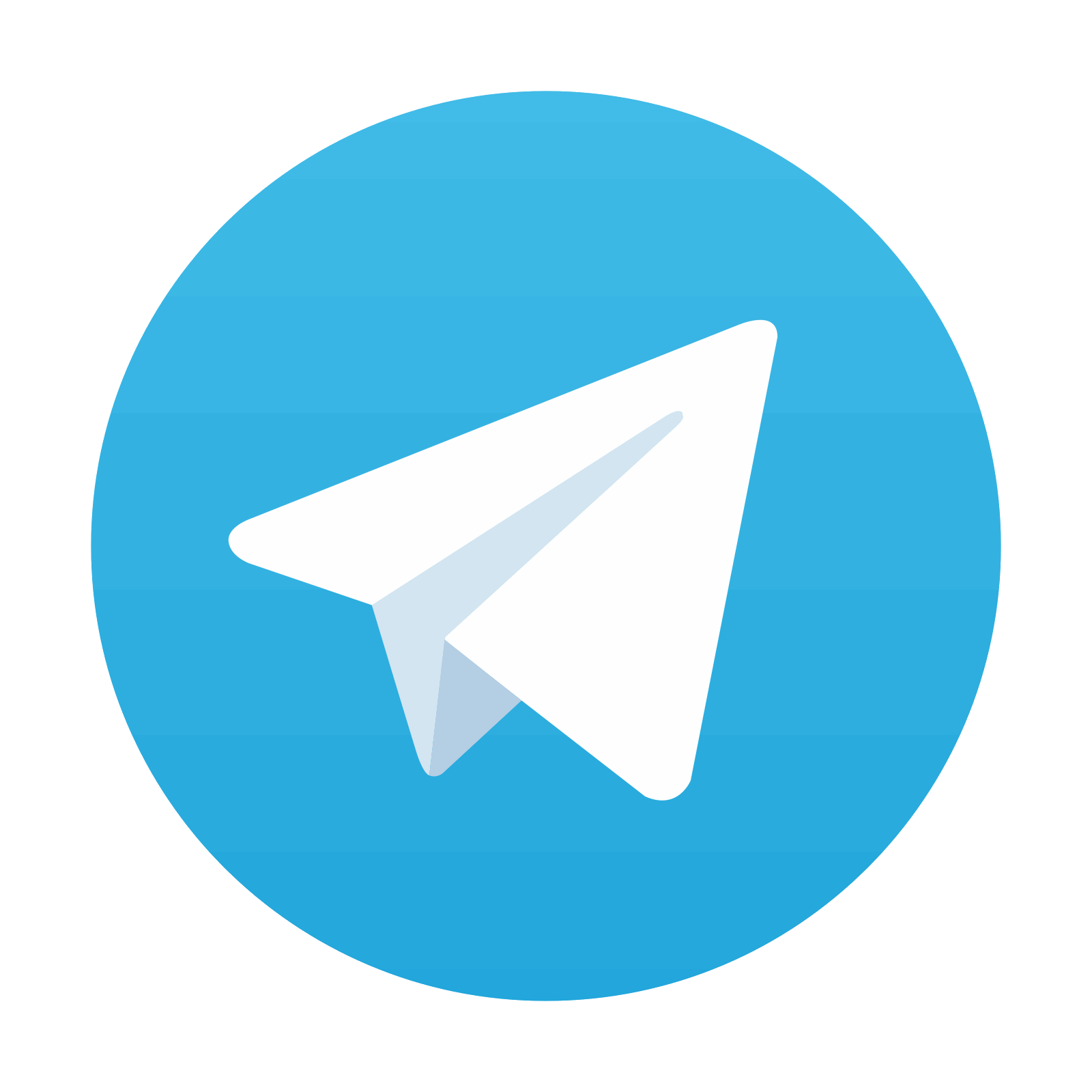
Stay updated, free articles. Join our Telegram channel

Full access? Get Clinical Tree
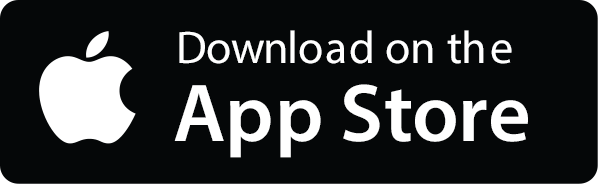
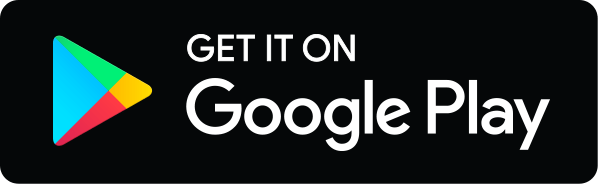