© Springer International Publishing AG 2018
Philip T. Cagle, Timothy Craig Allen, Mary Beth Beasley, Lucian R. Chirieac, Sanja Dacic, Alain C. Borczuk, Keith M. Kerr, Lynette M. Sholl, Bryce Portier and Eric H. Bernicker (eds.)Precision Molecular Pathology of Lung CancerMolecular Pathology Libraryhttps://doi.org/10.1007/978-3-319-62941-4_2121. Liquid Biopsies
(1)
Cancer Center, Houston Methodist Hospital, Houston, TX, USA
Keywords
Circulating tumor cells (CTCs)Cell-free tumor DNA (ctDNA)ExosomesEducated plateletsLiquid biopsiesLung cancer is the leading cause of cancer mortality and an international public health issue [1]. After many years of very slow and disappointing clinical progress over the past decade, the advent of targeted therapies and immunotherapies has significantly expanded our available armamentarium against advanced lung cancer as well as other metastatic solid tumors. Similarly, the revolution in genomics has provided a much better understanding of what drives cancer initiation and growth and allowed improved drug development against oncogene-addicted tumors. However, in many cases of metastatic solid tumors, tissue biopsy offers scarce material and hence next-generation sequencing is impossible. Patients suffering with advanced lung cancer often pose a significant challenge for pathologists and treating clinicians as current treatments necessitate reliable genomic information. However, they frequently have disease that is only accessible through invasive biopsies and, in addition, often have significant comorbidities such as chronic obstructive pulmonary disease or coronary artery disease that increase their risk of complications from invasive biopsies. In the past when treating a patient required only a simple “yes” to the question of is it lung cancer, a small biopsy was adequate. However, with the veritable explosion of discovered driver mutations that now have available and well-tolerated therapies, coupled with the need to correlate tumor histology and the microenvironment as the field of immune-oncology advances, small biopsies are no longer acceptable to assess and plan state-of-the-art treatment for patients. Thus, the zeal that many oncologists are showing toward adopting the developing technologies of “liquid biopsies,” whether circulating tumor cells (CTCs), circulating tumor DNA fragments (ctDNA) , or exosomes and tumor-educated platelets, is understandable. This chapter will review the emerging science behind these tests and discuss the potential clinical benefit that is starting to emerge.
While the description “liquid biopsy” has been disputed by pathologists, the term has entered the vernacular and will undoubtedly stick. These “biopsies” are tests that are performed on the blood or other body fluids and do not require a needle put into a visceral organ or lymph node nor do they require a surgeon or pulmonologist to obtain it. There is no histologic or architectural information obtained. Obviously the target of interest—either whole cells in the case of CTCs or DNA fragments for ctDNA—is not liquid. Many potential roles can be envisioned should the technologies continue to develop and be shown to have meaningful clinical uses: diagnostic purposes when patients first present with a radiographic abnormality, monitoring of response to therapy, analysis of driver mutations for selection of targeted therapy, and further analysis when patients on targeted therapy develop acquired resistance. There will probably also eventually be interest in using it as a screening test in completely healthy patients (most likely even before evidence even exists to support that use). Clearly much work remains to be done in order to fully assess the strength and weaknesses of the various assays and how to best utilize them in the care of patients. Importantly, assessing the benefit will require multidisciplinary evaluation and consensus between oncologists, pathologists, and bench researchers.
Circulating Tumor Cells
The fact that cells could break off from the tumor colony and spread through the blood or lymphatics has been noticed for over a century, but it took a long time to develop techniques to reliably capture and analyze these cells. Even in many patients with advanced cancers, CTCs are rare compared to the normal blood cells that they travel among. Thus strategies were employed that tried to separate tumor cells out of the blood using cell capture techniques that relied on epithelial adhesion molecules such as EpCAM . The CellSearch system (Veridex) is approved by the FDA for monitoring some tumors, such as breast and colon cancer, however, not for lung cancer. It has been demonstrated in metastatic breast cancer that levels of CTCs correlate with progression-free survival as well as overall survival [2]. However, obviously not all pathogenic cells express EpCAM; cells that have undergone epithelial to mesenchymal transition will not be detected by this method, and this has led to the development of assays that do not depend on cell surface markers but rather on cell size. Krebs et al. compared two strategies for detecting CTCs in patients with advanced lung cancer: a cell surface marker-dependent method (CellSearch for EpCAM + cells) and a surface marker-independent method that relied on isolation by the size of the epithelial tumor cells (ISET) [3]. In a small study of 40 patients with advanced lung cancer, 23% had CTCs detected with the surface-marker approach while 83% of the ISET tests were positive. Still, there remains little consensus on how CTCs compare with cfDNA, the methods of collection are not standardized, and there is difficulty in controlling the pre-analytic phase [4]. Attempts have been made to try to enhance the enrichment phase in order to increase yield. Pallier and colleagues combined blood filtration and FISH assay optimized for CTC characterization to detect ALK-positive CTCs in the blood of patients known to harbor the mutation and found 18/18 had greater than four ALK-positive CTCs and negative controls had 1 or 0 CTCs [5]. Still, even with the ability to isolate and sequence single cells in patients with lung cancer, the primary information that has been derived from studying CTCs in patients with advanced lung cancer has been prognostic. Yields of CTCs have been higher in patients with small-cell lung cancer (SCLC) rather than NSCLC, and some studies have suggested that CTCs better predict survival than stage and that a reduction in the number of CTCs detected after the first cycle of chemotherapy has been associated with better outcomes [6]. While the data is intriguing, there is as of yet no routine clinical role for utilizing CTCs to monitor response in small-cell lung cancer, which also has much to do with the paucity of effective salvage therapies for patients who fail to respond to initial therapy or rapidly relapse [7]. The success of meaningful diagnostic information is always tethered to available therapeutic options.
CTCs have been studied in some of the other driver mutations that cause oncogene-addicted lung cancers. CTCs were looked at in four patients being treated with crizotinib for ROS-1-mutated lung cancers as well as in four ROS-1-negative patients [8]. All four ROS-1 patients with tissue-proven mutations also had CTCs harboring the mutation. Patients progressing on therapy had significant increase in ROS-1 copy number in their CTCs.
One of the appealing possible strategies of CTCs as opposed to ctDNA would be the development of technologies to culture patients’ CTCs ex vivo and allow assays of drug sensitivity [9]. Hodgkinson recently reported that CTCs from patients with small-cell lung cancer, either chemosensitive or chemo-refractory, are tumorigenic in immune-compromised mice [10]. Furthermore, the CTC-derived explants mirrored the donor patients’ response to cytotoxic chemotherapy. Similar results have been reported with CTCs from patients with colon cancer and prostate cancer [11, 12].
So while the techniques to isolate and study CTCs in lung cancer patients have improved upon prior results, the main explosion over the past few years in clinical application has not been utilizing CTCs but rather using ctDNA.
Circulating Tumor DNA
Circulating tumor DNA was noted 40 years ago to be detectable in the blood of patients, and even at that time, levels were noted to be higher in patients with metastatic disease and the levels dropped in patients who responded to therapy [13]. However it was not until the genomic revolution, ushered in by the Cancer Genome Project , that the ability to sequence and analyze circulating DNA fragments began to reliably provide meaningful, actionable data.
Healthy humans have free DNA fragments that circulate in plasma and can be detected using quantitative PCR techniques. In patients with cancer, a percentage of the circulating DNA is derived from the tumor cells, generally between 0.1% and 10% of the total circulating DNA [14]. ctDNA is thought to be released from tumor cells both from passive mechanisms , such as apoptosis and necrosis, and therapy-induced destruction such as from radiation and chemotherapy. Recent data looking at cfDNA from cultured cells suggest that the process is an active one of secretion and not release through apoptosis or necrosis [15]. cfDNA is enriched in fragments of 150–180 bp as one would expect from apoptotic fragmentation [16]. Initially cfDNA levels were noted to be quantitatively higher in cancer patients than normal controls; Yoon et al. found that the median plasma DNA concentration in lung cancer patients was 22.6 ng/ml compared to 10.4 ng/ml in healthy volunteers [17]. The blood specimen must be centrifuged within 2 h as cfDNA begins to degrade quickly.
The real breakthrough with the technology was when not just the circulating levels of DNA could be assayed but the somatic mutations present in the tumor could be detected. Coming at a time when multiple new targeted therapies for lung cancer were being developed and validated in trials, the technology seemed to open the door on following patients and detecting new acquired resistance mutations without having to subject the patients to repeated biopsies.
Bettegowda and colleagues reported their results using digital PCR in 640 patients with early stage and advanced malignancies [18]. In patients with metastatic pancreatic, ovarian, colorectal, bladder, gastroesophageal, breast, melanoma, hepatocellular, and head and neck cancers, ctDNA was detectable in over 75% of patients. In patients with advanced primary brain, renal, prostate, or thyroid cancers, the rate was under 50%. In patients with localized disease, ctDNA was detectable in 73% of patients with colorectal cancer, 57% of gastroesophageal cancer, 50% of breast cancers, and 48% of pancreatic cancers. Importantly, ctDNA was often detectable in patients when CTCs not found, indicating that these are different markers.
One of the questions that arises when discussing ctDNA is how well it correlates with the genomic landscape of the primary tumor . Of course, the issue of tumor heterogeneity and the resulting clinical implications remain a pressing and vexing one. Even when tissue is biopsied, there can be intra-tumoral variation in detected mutations, raising the question of the validity and actionable yield of single-site tumor biopsies [19]. Proponents of using ctDNA state that the value of blood-based assays is that it captures a better snapshot of the various mutations within the heterogeneous tumor colonies. Critics state that correlative studies between tissue and the primary site are still ongoing and that it is possible that the mutations detected in the blood come from necrotic cells and might not be driver mutations.
To try to answer this question, Zill et al. looked at somatic genomic profiling of 15,000 patients with advanced cancer utilizing a 70-gene panel run on ctDNA [20]. The researchers compared the results with the incidence of mutations found in tissue biopsy specimens from the TCGA. Over half of the patients had lung, colon, or breast cancer. The specific frequency of mutations by cancer type as well as the mutual exclusivity among driver mutations matched well with the results from tissue. The overall accuracy of ctDNA analysis was 336/386 (87%) with matched tissue and that increased to 98% when the tissue and blood were acquired within 6 months of each other. While many additional details need to be worked out, at least the current data would suggest that the mutations detected in cfDNA numerically correlate with what has been documented in the tumor.
It is important to point out that many of the early genomic-based basket trials , such as BATTLE 1 and BATTLE 2 performed at MD Anderson, utilized tissue biopsies, not ctDNA, and that current large-scale basket trials, such as NCI Match and the American Society of Clinical Oncology’s Targeted Agent and Profiling Utilization Registry (TAPUR) , also are looking at tissue-based sequencing [21, 22]. Much published data thus far, such as the SHIVA trial as reported by Le Tourneau, suggests that when trying to use tissue genomics to identify targets to exploit with targeted treatment, the response rates are low [23]. SHIVA was a genomic matching trial comparing molecularly targeted therapies with physician’s choice; 741 patients were screened to randomize 26%, and the progression-free survival was 2.3 months against 2.0 months, leading the authors to caution against treating patients outside of a clinical trial based on molecular profiling of the tumor. It is certainly possible that should plasma-based genomic profiling of patients with advanced cancers achieve further validation, then the number of patients who would potentially benefit would expand more easily in the community, especially in later-stage patients where repeat tissue biopsy could be problematic. Wheeler et al. recently reported on a single-institution study of 500 enrolled patients where the plan was to do comprehensive genomic profiling [24]. Three-hundred and thirty nine patients were successfully profiled, and patients with a higher score of genomic aberrations and medication matches had significantly better 6-month survival than patients with low scores. While this data needs to be confirmed, it does suggest that a significant minority of patients with advanced disease can potentially benefit from genomic profiling , and thus improving the ease and accessibility of acquiring such data would greatly expand the number of patients eligible for these treatments. To move the field forward, we will need not just refinements to show that we have the capacity to quickly obtain data; we will need to demonstrate that we can use the data to effect meaningful treatment that changes the course of the disease in patients.
The success of tyrosine kinase inhibitors (TKIs) for certain oncogene-addicted metastatic lung cancer has profoundly changed the management of patients and made biomarker testing imperative for formulating the most appropriate treatment plan for individual patients [25]. While blood-based diagnostics have not yet replaced tissue biopsy as part of the initial workup of patients, there might be situations when patients have undergone a biopsy and the tissue is insufficient to run specific mutation testing. While clinical characteristics are suggestive, there are no absolute historical features (smoking status, ethnicity) that fully predict for the presence or absence of targetable mutations. In that case, the treating clinician might elect to re-biopsy, or they might decide to run ctDNA studies to look for actionable mutations.
Reck and colleagues looked at the ability of ctDNA to discover EGFR mutations in advanced lung cancer patients [26]. In the ASSESS study, patients with newly diagnosed lung cancer had tissue and ctDNA assays run. In 1162 matched samples, the mutational concordance was 89% (sensitivity 46%, specificity 97%, positive predictive value 78%, and negative predictive value 90%). The EGFR mutation frequency was 16% on tissue and 9% on plasma. Patients with extra-thoracic metastases were more likely to have mutations detected in plasma (13%) versus intrathoracic metastases (7%). This study confirmed that detection of ctDNA for EGFR mutations was possible in real time across many centers in different countries.
Despite the initial success of most targeted therapies to achieve clinical response, the majority of patients develop acquired resistance and subsequent radiographic or clinical progression. Many centers began repeating biopsies in patients who progressed in order to look at the mutation spectrum and see what was driving therapeutic resistance [27]. While many patients agree to re-biopsy, which initially struck many oncologists as counterintuitive, the yield was not 100%. Drilon et al. at MSKCC presented data on patients with adenocarcinoma of the lung with initial negative NGS, and they underwent hybrid capture and almost a third had actionable mutations [28]. Interestingly, many of those patients had between two and four biopsies in order to get successful next-generation sequencing , a strategy that is helpful for generating hypotheses but not one that is practical across a large population.
The development of TKIs that were active in cases of acquired resistance made re-biopsy an urgent issue in patients with advanced lung cancer progressing on initial therapy. Recently, a number of studies have confirmed that ctDNA can play a major role in detecting resistance mutations and help guide selection of therapy. Oxnard et al. recently reported their results using plasma genotyping using BEAMing in patients on a trial of a third-generation TKI osimertinib in patients with EGFR-mutated lung cancer [29]. They compared the plasma results to tissue genotyping run at a central laboratory. The sensitivity of plasma genotyping was 70%.
Regardless of whether the patient was T790 positive in tissue or blood, osimertinib was effective and response rates were similar. Obviously since the sensitivity is only 70%, patients negative on blood would require a biopsy as clinicians do not want to deny patients the chance to remain on targeted therapy should the tissue biopsy be positive.
Sacher and collegaues utilized digital droplet PCR in looking at two groups of patients: advanced lung cancer patients at the time of initial diagnosis and patients who were found to be EGFR mutation positive and progressed on initial TKI therapy [30]. All patients underwent tissue biopsy to use as the reference sample to correlate with the blood specimens, and the blood was analyzed for the presence of EGFR exon 19 del, L858R, T790 M, and/or KRAS G12X. The median turnaround time for ddPCr at initial diagnosis was 3 days (range 1–7), and the tissue genotyping median TAT was 12 days (range 1–54) for newly diagnosed disease and 27 days (range 1–146) for acquired resistance. Plasma ddPCR had a positive predictive value of 100% for EGFR 19 deletion mutations, L858R, and KRAS and a 79% for T790. The sensitivity of the assay was 82% for EGFR 19, 74% for L858R, and 77% for T790. The sensitivity for EGFR and KRAS mutations was better in the setting of multiple metastatic sites and especially with extra-thoracic metastases to the liver or bone. The study shows that rapid TAT was possible for a defined molecular target that could significantly decrease the need in many patients to undergo a repeat biopsy.
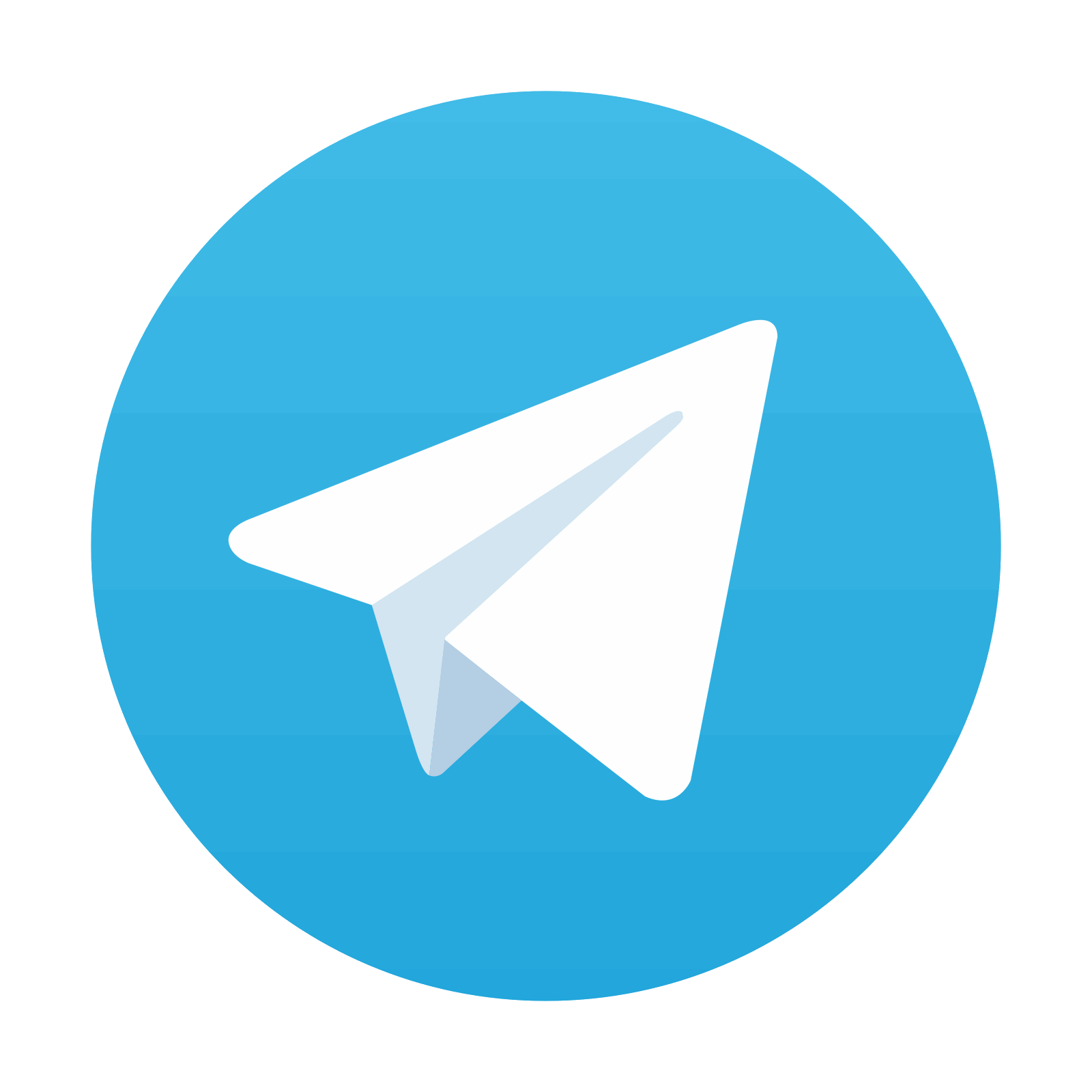
Stay updated, free articles. Join our Telegram channel

Full access? Get Clinical Tree
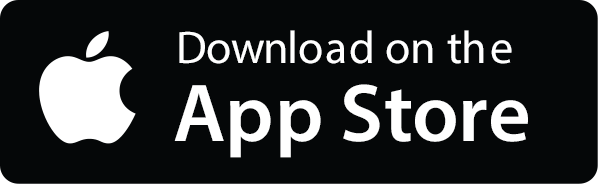
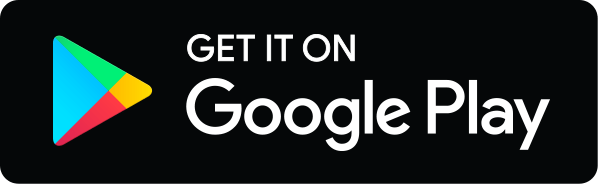