Infections in Skeletal Prosthesis and Allografts
Ilker Uçkay
Leonardo Pagani
Axel Gamulin
Daniel P. Lew
INTRODUCTION
Millions of orthopedic implant procedures are performed worldwide each year. The safety and biocompatibility of these devices are good and only 10% of patients experience complications during their lifetime. After loosening of the prosthesis (Figure 40.1), infection is the most common cause of complications. At present, the lifetime infection rate is thought to be 0.5% to 1% for primary total hip arthroplasty, 0.5% to 2% for primary knee replacement, and <1% for shoulder replacement (1,2). Even though the incidence has fallen steadily, the absolute number is rising because of the increase in the number of the procedures performed. For the United States alone, it is projected that ˜4 million artificial joints will be implanted each year by 2030 (3). Thus, the number of prosthetic joint infections is expected to increase. The economic burden of healthcare-associated infection (HAI) with septic prosthetic joints is very high. The cost to treat patients with hip or knee prosthetic osteomyelitis has been calculated to be 5.3- and 7.2-fold higher, respectively, than the primary operations (4).
MUSCULOSKELETAL ALLOGRAFTS
Allografts in orthopedics are gaining momentum. In the United States, ˜1 million musculoskeletal allografts are currently distributed by tissue banks (5) compared to 350,000 in 1990 (6). Processed tissue allografts are not necessarily sterile and may result in viral or bacterial infections. Among patients who had surgery for bone tumor and revision hip arthroplasty, Tomford et al. reported a 5% and 4% incidence, respectively, of infection related to the use of allografts (7). In a series of 945 patients who received cadaveric allografts, Mankin et al. reported 7.9% primary infections (8).
PATHOGENESIS
The usual mechanism involved is the introduction of microorganisms during the operative procedure as the freshly implanted biomaterial is highly susceptible to contamination. It is usually admitted that most joint infections are acquired in the operation theater. Arguments to support this are the efficacy of perioperative antibiotic prophylaxis, laminar flow in the operating room, and the similarity between skin flora and the pathogens that cause prostheses infections (2). Moreover, during the early postimplantation period when superficial infections can develop, the fascial layers have not healed and the deep, periprosthetic tissue is not protected by the usual physical barriers. Any factor or event that delays wound healing increases the risk of infection: ischemic necrosis; hematomas; and, more directly, wound sepsis or suture abscesses. Late infections may emerge after 2 years of pain-free mobility (≥15% of infections) and are mostly attributed to hematogenous seeding with selective persistence of the microorganisms in the joint (1,9).
MICROORGANISMS
Virtually any microorganism can cause prosthetic joint infection. Data from several series indicate that a single pathogen can be identified in only about two-thirds of cases (10). The predominant microorganisms are Staphylococcus sp. (˜50% in several series), evenly divided between S. epidermidis and S. aureus. Aerobic streptococci are responsible for a significant group of infections (between 10% and 20% in different series), followed by gram-positive organisms ordinarily considered as “contaminants” of cultures, such as Corynebacterium spp., Propionibacterium spp., and Bacillus spp. Gram-negative aerobic bacilli have been identified in some series in ≤25% of cases, but anaerobes usually do not account for >10% of all pathogens. Among arthroplasty patients with solid organ transplantations, more atypical pathogens such as mycobacteria may occasionally be observed (11). In up to 10% of cases, no organisms can be detected.
Among allografts, gram-positive bacteria are equally the most frequent organism associated with infection (12). Microorganisms, such as Clostridium spp., have become a concern (13). In 2003, Malinin et al. showed that 8.1% of donor blood, marrow, and musculoskeletal samples grew clostridia, mainly C. sordellii, among 795 donors in the United States (10).
ROLE OF THE FOREIGN BODY AND BIOFILMS
Attachment of bacteria to a prosthetic joint is a critical first step in the pathogenesis of virtually all foreign body-associated infections. A subcutaneous foreign body reduces the inoculum of S. aureus required to induce infection by more than 100,000-fold to as few as 100 colony-forming units (14). In addition, the interaction of neutrophils with the foreign body can induce a neutrophil defect that may enhance the susceptibility to infection (15). Bacteria embedded deeply within
the biofilm are metabolically inactive or in various stages of dormancy, thus protected from host defenses, such as phagocytes, and highly resistant to antimicrobial agents (16). The microenvironment within a biofilm may also adversely affect diffusion of antimicrobial agents. Soon after a biofilm is established, the susceptibility of bacteria to antimicrobial agents often shows a logarithmic decline. With an infection of >1-month duration, it has been postulated that the biofilm has progressed to such a degree that cure with prosthetic retention is less achievable than with resection. In addition, ultrahigh molecular weight polyethylene particles emitted by prosthesis material seem to add to the inhibition of the neutrophil antibacterial activity (17).
the biofilm are metabolically inactive or in various stages of dormancy, thus protected from host defenses, such as phagocytes, and highly resistant to antimicrobial agents (16). The microenvironment within a biofilm may also adversely affect diffusion of antimicrobial agents. Soon after a biofilm is established, the susceptibility of bacteria to antimicrobial agents often shows a logarithmic decline. With an infection of >1-month duration, it has been postulated that the biofilm has progressed to such a degree that cure with prosthetic retention is less achievable than with resection. In addition, ultrahigh molecular weight polyethylene particles emitted by prosthesis material seem to add to the inhibition of the neutrophil antibacterial activity (17).
DIAGNOSIS
Diagnosis of prosthetic infection can be difficult. If there is no sinus tract communicating with the artificial joint or the presence of the same pathogen in at least two separate tissue or fluid samples from the affected joint, diagnosis of infection becomes difficult and relies on a combination of clinical, anamnestic, laboratory, and histologic parameters (18).
CLINICAL PRESENTATION
There are no uniform clinical criteria for the diagnosis of prosthetic joint infections. Most patients experience a long, indolent course of infection characterized by steadily increasing joint pain and the occasional formation of cutaneous draining sinuses. A minority of patients have an acute fulminant illness associated with high fever, severe joint pain, local swelling, sinus tract, and erythema. Patients with late-onset infections due to hematogenous seeding can present with this acute onset of symptoms in one or several previously well-functioning joints. The pattern of clinical presentation is determined largely by the nature of the infecting microorganism (i.e., the symptoms are more prominent in S. aureus infections compared with S. epidermidis). Infection must be differentiated from aseptic mechanical problems. Constant joint pain suggests infection, whereas mechanical loosening commonly causes pain only with motion and weight-bearing. Nevertheless, it is often difficult to differentiate delayed-onset infection from aseptic joint loosening of hip or knee prostheses.
ERYTHROCYTE SEDIMENTATION RATE AND C-REACTIVE PROTEIN LEVELS
Persistent elevation of the erythrocyte sedimentation rate (ESR) suggests infection, but is neither very sensitive nor very specific since it may be due to many other causes. The same is true for leukocytosis or C-reactive protein (CRP). The combined measurement of CRP and the ESR seems to have an acceptable negative predictive value, although it does not completely rule out a prosthetic joint infection. These two parameters are also more appropriate to measure as follow-up during therapy than for diagnosis. The role of procalcitonin in nonbacteremic prosthetic infections has to be further investigated. A study performed at our center did not prove a diagnostic or follow-up value of procalcitonin in localized prosthetic joint infections (19). In the future, the use of other inflammatory markers in the diagnosis of infection will certainly emerge of which the serum leukocyte esterase warrants further investigation, for example (20).
RADIOLOGY
A plain radiograph can display abnormal lucencies (>2 mm in width) at the bone-cement interface, changes in the position of prosthetic components, cement fractures, periosteal reaction, or the motion of components. Periosteal new bone formation is suggestive of infection, but infrequently present as is the presence of fistula between the joint and soft tissue. When both the distal and proximal components of a prosthetic joint demonstrate radiographic abnormalities, infection is more likely than simple mechanical loosening. The sensitivity and the specificity of radiographic anomalies have been reported as 73% and 76%, respectively (21). Magnetic resonance or computed tomography techniques for evaluating prosthetic joints for infection are of little help because the metal present in prostheses interferes with these images. Radioisotopic scans demonstrate increased uptake in areas of bone with enhanced blood supply or increased metabolic activity, but this does not help to diagnose true infection because increased uptake is routinely observed around normal prostheses for several months after arthroplasty. Smith et al. investigated the usefulness of bone scintigraphy after a mean period of 3 years between knee replacement surgery and the onset of knee pain. However, the pattern of isotope uptake in the abnormal studies was not specific enough to differentiate aseptic from septic loosening (22). This result was confirmed in a report of 144 patients who underwent revision hip arthroplasty; bone-gallium imaging offered no additional diagnostic advantage over hip aspiration (23). According to Bernard, the sensitivity and specificity of bone scintigraphy is at best 76% (21). Only limited data are available for positron emission tomography (PET). In a report of 35 patients with painful hip replacements, PET scan performed similarly to scintigraphy and was less sensitive and more specific than
conventional bone radiography (24). In conclusion, a normal bone scan is generally useful to exclude the need for surgical intervention aimed at correcting loosening or infection of the joint (25).
conventional bone radiography (24). In conclusion, a normal bone scan is generally useful to exclude the need for surgical intervention aimed at correcting loosening or infection of the joint (25).
MICROBIOLOGIC CULTURES AND HISTOLOGY
Several identical positive microbiologic cultures identifying the same microorganism(s) are currently the gold standard to confirm the clinical suspicion of infection. As a result, the diagnosis of prosthetic joint infection always requires obtaining samples of joint fluid or tissue (26). The most important step is to isolate the offending organisms so that the appropriate antimicrobial therapy can be chosen. The importance of the identification of the pathogen cannot be overemphasized. Alternatively, isolation can be achieved by blood cultures, sonication of explanted prostheses (27), or by direct biopsy from the involved bone, since joint fluid aspirates may be falsely negative (28). Whenever biopsies or aspirations are done, the samples should be processed for aerobic and anaerobic cultures. Samples for mycobacterial and fungal cultures should be taken and processed if commonly cultured microorganisms are not present and if the clinical features are compatible. Often, culture growth has to be extended beyond the standard incubation period of 5 days. Cultures may be negative because of prior antimicrobial exposure, a low number of organisms, an inappropriate culture medium, fastidious organisms, or prolonged transport time to the microbiology laboratory. Some microorganisms, such as Abiotrophia spp. or staphylococcal small-colony variants (SCV), are inherently difficult to detect (29). In contrast to the normal staphylococcal phenotype, SCVs are characterized by an enhanced ability to persist intracellularly, fastidious growth characteristics, nonpigmented and nonhemolytic pinpoint colonies after 24 to 72 hours of incubation on blood agar, and the absence or reduction of biochemical reactions (e.g., mannitol salt agar negative) (29). SCVs are rapidly overgrown and are easily missed when the normal S. aureus is present because they divide about nine times slower than normal-phenotype S. aureus. Isolates suspicious for S. aureus SCVs, which may give a false-negative coagulase test, should be confirmed as S. aureus by testing the species-specific genes or by diagnostic sequencing of 16S rDNA sequences (29).
Eubacterial polymerase chain reaction (PCR) has usually only little sensitivity and is still relatively expensive, which excludes its routine application. In polymicrobial colonization or infection, its interpretation can be difficult. Moreover, it does not provide information about antibiotic resistance, except for genes coding for methicillin resistance (30). However, specific or multiplex PCR is beneficial in special circumstances when very slow- or difficult-growing bacteria are suspected, such as Kingella kingae, Brucella spp., Coxiella burnetii, Bartonella henselae, Mycobacterium tuberculosis, or M. ulcerans.
Tissue specimens should also be submitted for histopathologic study, as special staining techniques may reflect unusual or slow-growing microorganisms. A histopathologic examination showing acute inflammation has a >80% sensitivity and >90% specificity for the diagnosis of infection (31). However, the results can be dependent on appropriate sampling of the tissue harboring the infection and the expertise of the pathologist. Not all centers will have pathologists who are experienced in this type of histopathologic analysis.
TREATMENT
Treatment of prosthetic joint infections is not standardized due to the variable clinical presentations and the lack of data from randomized, controlled trials. The treatment usually involves both medical and surgical measures (1), depending upon the cause and timing of the infection, and the condition of the host.
SURGICAL THERAPY
When the diagnosis of infection is established, the treatment options are debridement with prosthesis retention, resection arthroplasty, one-or two-stage reimplantation, or arthrodesis.
SURGERY WITHOUT PROSTHESIS REMOVAL
Antibiotic treatment alone, without the removal of the prosthesis, is not considered standard therapy for prosthetic joint infection and has been associated with a >90% failure rate. However, in selected patients, it may be an option under the condition that at least one early concomitant surgical debridement is performed. It is limited to a small subset of patients, that is, patients with an early (<4 weeks postoperatively) acute onset of symptoms and a well-fixed prosthesis without signs of loosening or sinus tract. For some authors, infection with S. aureus is a relative contraindication to debridement and component retention (29), whereas others consider only the methicillin-resistant strains as a contraindication for prosthesis retention (32).
PROSTHESIS REMOVAL
Upon removal of the prosthesis, there are several options. These include resection arthroplasty without reimplantation, arthrodesis, amputation, and one- or two-stage reimplantation of a new prosthesis.
In the absence of a prospective randomized study with prolonged follow-up comparing these two different surgical approaches, it is difficult at present to recommend one- over two-stage arthroplasty (1,33). With one-stage exchange arthroplasty, the infected components are excised, surgical debridement is performed, and a new prosthesis is immediately put in place under antibiotic coverage. It may be suitable in highly selected hip prosthesis patients who have satisfactory soft tissue, no severe coexisting illnesses, no fistula, no need for bone graft, and infection with organisms that are highly susceptible to antimicrobial drugs (33). Some authors have reported 85% to 90% success rates with this approach (29,33). These series are often characterized by susceptible organisms, the use of antibiotic-impregnated cement, and prolonged postoperative antibiotic therapy. For hip prosthesis, an analysis of published series does not show any advantage of one-stage exchange arthroplasty with or without antibiotic cement compared to a two-stage approach. Rather, a two-stage approach seems to yield better results, but at higher costs.
The two-stage approach requires careful surgical removal of all foreign body material and infected tissue followed by prolonged parenteral and oral therapy (1,34). Reported success rates of more than 90% are achieved with two-stage replacement arthroplasty for infected hip prostheses with the interim use of antibiotic-loaded cement (35). The advantages of two-stage
hip reimplantation arthroplasty are that it allows for additional debridement and optimization of the choice of antibiotic and duration of therapy for more virulent pathogens. The disadvantage is that the second intervention is more difficult to perform as a result of scarring and can signify a second perioperative morbidity and mortality risk for patients of advanced age and serious comorbidities. In patients who have experienced extensive bone loss, a third stage consisting of bone grafting is required between resection and reimplantation. Most centers in the United States use two-stage arthroplasty, whereas the one-stage approach is more commonly performed in Europe with a variable intermediate period between excision and reimplantation (1). Of note, the precise duration of antibiotic therapy after debridement and implant retention is somewhat arbitrary, with suggestions ranging from 3 to 6 months (for knee arthroplasties) (1,36). Joint aspiration before reimplantation is advocated by some experts to rule out dormant infection/presence of pathogens. This approach is not evidence-based for routine application and should be used in selected cases when the clinician is concerned about persistent infection. Indeed, in many studies, this preimplantational joint aspiration may reveal false-negative or -positive results (28).
hip reimplantation arthroplasty are that it allows for additional debridement and optimization of the choice of antibiotic and duration of therapy for more virulent pathogens. The disadvantage is that the second intervention is more difficult to perform as a result of scarring and can signify a second perioperative morbidity and mortality risk for patients of advanced age and serious comorbidities. In patients who have experienced extensive bone loss, a third stage consisting of bone grafting is required between resection and reimplantation. Most centers in the United States use two-stage arthroplasty, whereas the one-stage approach is more commonly performed in Europe with a variable intermediate period between excision and reimplantation (1). Of note, the precise duration of antibiotic therapy after debridement and implant retention is somewhat arbitrary, with suggestions ranging from 3 to 6 months (for knee arthroplasties) (1,36). Joint aspiration before reimplantation is advocated by some experts to rule out dormant infection/presence of pathogens. This approach is not evidence-based for routine application and should be used in selected cases when the clinician is concerned about persistent infection. Indeed, in many studies, this preimplantational joint aspiration may reveal false-negative or -positive results (28).
Antibiotic-impregnated polymethylmethacrylate (PMMA) spacers are used by many surgeons to maintain soft-tissue tension around the joint during the interval between debridement and reimplantation in two-stage procedures. Some authors suggested benefits of this technique, including high levels of local antibiotic delivery, improved exposure at the time of re-implantation, and the ability to maintain weight-bearing during the interval period (29). However, concerning the outcome of recurrence of infection, no trial so far has ever demonstrated a superiority of local antibiotic therapy compared to combined local and systemic antibiotic therapy or to systemic antibiotic treatment alone (37).
SUPPRESSIVE ANTIBIOTIC TREATMENT
Exchange surgery is fastidious, time-consuming, and can be debilitating for patients with multiple comorbidities. As an alternative, lifelong suppressive oral antimicrobial treatment without surgical intervention is an option. Even if suppressive treatment is a palliative treatment, most experts recommend a lavage of the infected joint in order to reduce the inoculum.
ARTHRODESIS OR AMPUTATION
Arthrodesis as treatment of an infected arthroplasty can provide a stable, generally painless, limb with some expected shortening. The last option for the treatment of the infected arthroplasty is amputation. It essentially concerns infected knee arthroplasty. Amputation is indicated only for life-threatening infection or persistent local infection with massive bone loss not amenable to arthrodesis.
ANTIMICROBIAL THERAPY
Antibiotic therapy is very often concomitant to surgery and has some particularities to consider for osteoarticular infections. One important issue is antimicrobial penetration into bone or biofilm. A review by Landersdorfer et al. (38) on the penetration of antibacterials into bone highlighted how the application of different methods for the determination of drug concentrations may affect the results. The equilibrium in bone in relation to the antimicrobial plasma concentration after systemic administration may be influenced by several factors, including the physicochemical properties of the drug, its degree of protein binding and compartmental clearance, and by the particular structure of the bone tissue itself. For example, macrolides, linezolid, and quinolones show mean bone-to-serum concentration ratios between 0.3 and 1.2, whereas the average ratio ranges between 0.15 and 0.3 for cephalosporins, penicillins, and glycopeptides (38).
PARENTERAL ANTIBIOTIC THERAPY
Previously, experts usually recommended intravenous (IV) antibiotic therapy for 4 to 6 weeks (1,29) followed by an oral course of additional weeks or months. The rationale for a prolonged IV course was elevated serum concentrations. Today, opinion has switched to IV treatment during the initial 2 weeks (29), although this is based on expert opinion rather than on clinical trials. Without doubt, bone penetration of antibiotic agents in parenteral administration is acceptable with a bioavailability per definition of 100% (39). At the same time, IV medication should be limited as far as possible to avoid unnecessary costs, prevent catheter-related complications, and to increase patient and nursing comfort. The estimated proportion of complications attributed to a prolonged IV course is ˜15% (40). Recent retrospective data suggest that regimens with an early switch to oral antibiotics are as effective as prolonged parenteral regimens, at least for osteomyelitis (29). As a clinical example, Cordero-Ampuero et al. cured 36 arthroplasty infections with oral antibiotics administered from the start (41).
CHOICE OF ANTIBIOTIC AGENTS IN RELATION TO BONE, IMPLANT, AND BIOFILM
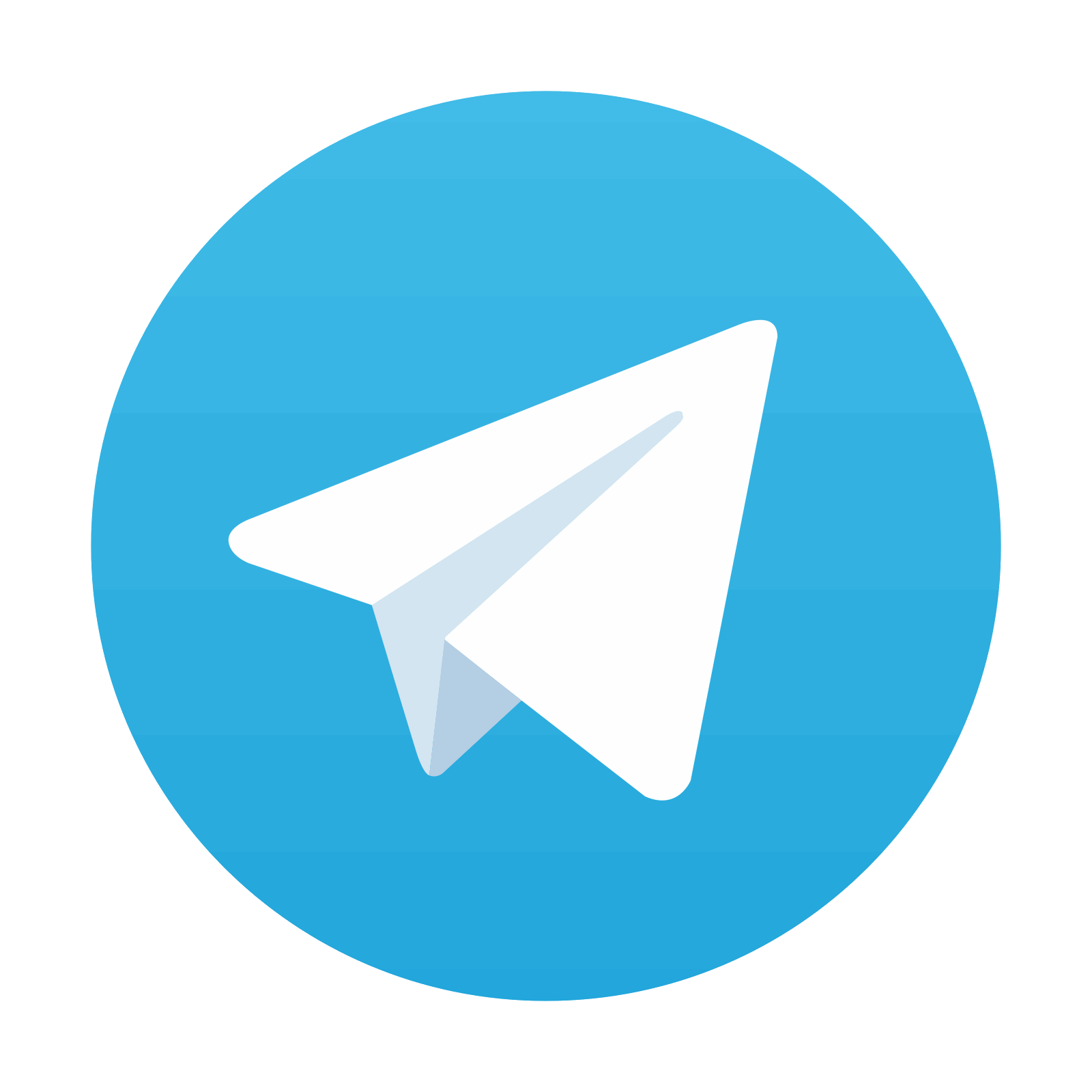
Stay updated, free articles. Join our Telegram channel

Full access? Get Clinical Tree
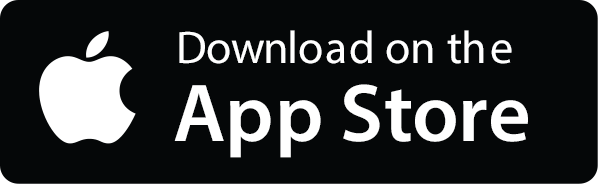
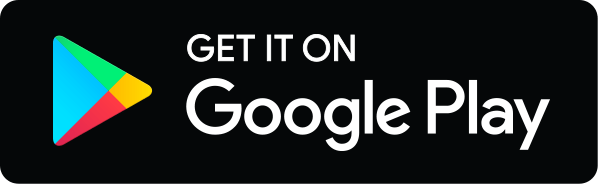