Chapter Outline
KEY COMPONENTS OF THE IMMUNE SYSTEM AND THEIR RELEVANCE IN CANCER IMMUNOTHERAPY
TARGET ANTIGENS IN CANCER IMMUNOTHERAPY
Antigens Arising from Genetic Alterations
Clonally Rearranged Immunoglobulin Genes
Altered Glycolipid and Glycoprotein Antigens
Products of Genes That Are Selectively Expressed in Immune-Privileged Sites
Molecules That Activate Recognition of the Innate Immune System
Normal Proteins Overexpressed in Tumor Tissue
ADOPTIVE CELLULAR IMMUNOTHERAPY
GVL Effect in HSCT and Donor Lymphocyte Infusions
Adoptive Therapies Exploiting NK-Cell Alloreactivity
Adoptive Therapy Using Cytokine-Induced Killer Cells
Allogeneic Adoptive T-Cell Therapy for the Control of Viral Infections After HSCT
The role of the immune system in controlling cancer was subject to debate for decades, reflecting the limited understanding of the immune system at the time and the complexity of tumor immunology. However, over time a wealth of evidence from murine and human studies has demonstrated that the immune system can control cancer. Numerous animal models have demonstrated the ability of T cells to reject tumors, revealed the importance of cytokines for antitumor immunity, and showed the susceptibility of immunodeficient mice to tumor formation. Compelling evidence of tumor immunosurveillance in humans is provided by reports of rare spontaneous tumor regressions in persons with melanoma and renal cell carcinoma, particularly after infection, paraneoplastic diseases (which are autoimmune neurologic manifestations of an antitumor response), and the higher incidence of melanoma in patients undergoing chronic pharmacologic immunosuppression after organ transplantation. Pediatric patients with immunodeficiencies are at risk for lymphoma, and posttransplant lymphoproliferative disease (PTLD) is a well-known entity in iatrogenically immunosuppressed patients. The immune system can prevent virus-induced malignancies, eliminate tumor cells via recognition of tumor antigens that distinguish them from “self,” and promptly terminate inflammation to avoid tumorigenesis. However, when the latter process is in disarray, such as in chronic inflammatory conditions and autoimmunity, the immune system is implicated in tumorigenesis. The control of tumor growth by the immune system also applies selection pressure, which may ultimately promote tumor progression. In this process, termed “cancer immunoediting,” cancer cells develop immune escape mechanisms and establish a tumor microenvironment that interferes with antigen presentation, T-cell activation, and differentiation, thereby leading to a gradual loss of control by T cells ( Fig. 47-1 ). An expanding body of preclinical and clinical experiences has established that the immune system can be manipulated in increasingly sophisticated ways to prevent, control, or aid in the control of established cancers. Careful selection of the appropriate target antigen(s) and consideration of preexisting immunologic memory are key features in the pursuit of successful immunotherapy. Antigens that are unique to the tumor and absent on healthy tissues often require priming of the immune system but possess the advantage that the induced antitumor response is unlikely to have autoimmune adverse effects. In the case of antigens that are present or overexpressed on tumor cells but are also detectable in some healthy tissues, depletion of T-cell clones with high avidity to the antigen may have polarized existing memory T cells toward tolerance. In this scenario, effective immunotherapy has to break tolerance and is more likely to induce undesired autoimmunity. The clinical use of immunotherapeutic modalities such as monoclonal antibodies (mABs), vaccines against oncogenic viruses, cytokines, and hematopoietic stem cell transplantation (HSCT) has been the standard of care in the prevention, treatment, and supportive care of cancer for many years. More recently, an exciting renaissance in cancer immunotherapy occurred as a result of the success of proof-of-concept clinical trials for a variety of novel immune-modulating strategies. As will be discussed further, these strategies include cellular therapies such as chimeric antigen receptor (CAR) T-cells (CARTs) and the first Food and Drug Administration (FDA)–approved cancer vaccine (sipuleucel-T); blockade of regulatory pathways with anti–cytotoxic T-lymphocyte antigen 4 (anti-CTLA-4) mABs; and antibody-targeted delivery of drugs via immunoconjugates such as brentuximab vedotin and trastuzumab emtansine. Many early-phase immunotherapy trials are being conducted for adult malignancies first and will be discussed when they are applicable. While promising results and toxicity data emerge from adult trials, the benefits of immunotherapy will also increasingly become available to pediatric patients with cancer.

Key Components of the Immune System and Their Relevance in Cancer Immunotherapy
The immune response can be divided into innate and adaptive immunity to differentiate between an early, generalized response against evolutionarily conserved molecular patterns and a highly specific response that forms fully as a result of exposure to specific antigens. One can also distinguishe a humoral from a cellular arm of the immune system. The humoral arm consists of soluble factors that are secreted by immune cells and includes antibodies and cytokines. The cellular arm encompasses antigen-presenting cells (APCs), phagocytes, lymphocytes, and innate immune cells.
Humoral Arm of the Immune System
Antibodies
B cells have the ability to differentiate into plasma cells and secrete antibodies. B-cell receptors, which represent surface-expressed antibodies against a vast variety of potential antigens, are created by somatic V(D)J-recombination. Subsequent antigen exposure leads to clonal expansion of B cells with the cognate B-cell receptor and facilitates large-scale antibody production. Antibodies consist of two heavy chains (γ, δ, α, µ, or ε) and two light chains (λ or κ). The composition of the heavy chains determines the immunoglobulin (Ig) subclass, which can be IgM, IgG, IgA, IgE, or IgD. These immunoglobulin subclasses have different binding valencies and functions; for example, IgM is produced early in the immune response followed by a class switch to IgG, whereas IgA is predominantly involved in mucosal immunity. Antibodies function by binding to the antigen of their specificity via the antigen-binding fragment (Fab; consisting of one constant and one variable domain from each of the heavy and light chains), thereby marking their target for destruction in a process termed “opsonization.” Cellular destruction is mediated by the crystallizable fragment (Fc) component, which is composed of two heavy chains ( Fig. 47-2 ). Depending on the effector cell and type of Fc receptor (FcR), the immune response can be modulated in multiple ways. Activating FcRs contain a cytoplasmic immunoreceptor tyrosine activation motif, whereas inhibitory FcRs signal via an immunoreceptor tyrosine inhibition motif. FcR binding can lead to antibody-dependent phagocytosis (ADP) by macrophages or APCs or cell destruction by natural killer (NK) cells via antibody-dependent cellular cytotoxicity (ADCC). The Fc component can also fix complement, leading to complement-dependent cytotoxicity (CDC). Antibodies can further kill tumor cells by direct induction of apoptosis and blockade of growth factors or other stimulatory signals ( Fig. 47-3 ).


Cytokines
Cytokines are soluble proteins that are predominantly produced by leukocytes and carry out intercellular signaling function. Based on their varied functions, they can be classified into interleukins (ILs), interferons (IFNs), chemokines, and hematopoietic growth factors. Several cytokines have been used in cancer immunotherapy either as a single agent or to enhance the effect of other therapeutic agents. Growth factors such as granulocyte-colony stimulating factor (G-CSF) also play an important role in facilitating the neutrophil recovery necessary for compressed chemotherapy regimens, which have led to improved survival for children with multiple types of pediatric solid tumors. Lastly, analysis of the cytokine response triggered by immune therapies is informative in elucidating the mechanism of action and associated toxicities and can guide lifesaving therapeutic interventions, as demonstrated by the successful use of an IL-6 receptor (IL-6R) antagonist in the treatment of cytokine release syndrome associated with cluster of differentiation (CD)19-CAR therapy.
Cellular Arm of the Immune System
T Cells
T cells are a major component of the adaptive immune system ( Fig. 47-4 ). They are derived from bone marrow, but in contrast to B cells, they travel to the thymus to undergo V(D)J-recombination of their T-cell receptors (TCRs), editing, and maturation. Somatic recombination of the α- and β-chains of the TCR results in the incredibly diverse T-cell repertoire from which clonal expansion can occur upon encounter of a specific antigen. To prevent autoimmunity, TCRs specific for “self” undergo negative selection in the thymus but also are subject to regulatory mechanisms in the periphery. Generation of an adaptive antitumor response begins with capture of tumor antigens by dendritic cells (DCs) in the peripheral tissues. DCs then travel to the draining lymph nodes, where they interact with T cells. Successful activation of a T-cell response (T-cell priming) requires presentation of the antigen on major histocompatibility complex class I (MHC-I) or class II (MHC-II) molecules for TCR-mediated recognition (signal 1), as well as a costimulatory signal (signal 2). Classically, the costimulatory signal is delivered to CD28 on T cells via the B7 family (CD80 and CD86) on APCs, but many other costimulatory molecules have been identified. The absence of costimulation leads to anergy. T cells may also receive inhibitory signals via CTLA-4, programmed death 1 (PD-1), and various other receptors, which play a physiologic role in terminating an immune response. The tumor microenvironment exploits some of these mechanisms to escape immune surveillance by MHC downregulation, as well as expression of inhibitory ligands such as PD-1 ligand (PD-L1). The discovery and characterization of other modulatory signaling pathways is ongoing and will continue to be a platform for therapeutic intervention.

T cells express CD3 and are divided in CD8+ cytotoxic T lymphocytes (CTLs) and CD4+ helper T cells. DCs can process and present antigens via MHC-I and MHC-II pathways. CD8+ CTLs are generally activated via MHC-I. This pathway predominantly processes antigens derived from the cell itself (such as viral antigens), but it can also present antigens taken up by the cell through a mechanism referred to as cross-presentation. MHC-I is present on all nucleated cells, and upon recognition of the MHC-restricted cognate antigen on a tumor cell, primed CD8+ CTLs mediate tumor cell death by virtue of releasing perforin/granzyme B or by inducing apoptosis through interactions of death receptors such as Fas/Fas ligand. MHC-II expression is limited to APCs such as DCs, macrophages, and B cells, which process phagocytosed antigens and activate CD4+ T cells via the MHC-II pathway. CD4+ T cells do not generally directly mediate tumor cell lysis, but they critically support and influence the immune response by producing cytokines and providing “help” to CD8+ T cells and B cells via CD40 signaling. Depending on the polarization of their cytokine profile and function, CD4+ helper T cells are divided into Th1, Th2, Th17, and follicular helper T-cell subtypes and regulatory T cells (Tregs). Th1 responses are characterized by proinflammatory cytokines such as IFN-γ and tumor necrosis factor (TNF)-α, which enhance macrophage and CTL function and promote secretion of immunostimulatory cytokines, such as IL-12 by APCs. Th2 responses are essential for antibody production and class switching of B cells, are involved in allergic responses, and produce an array of cytokines such as IL-4, IL-9, IL-10, and IL-13 that contribute to eosinophil and mast cell activation. The Th17 subset was only described relatively recently. Although it is involved in the pathogenesis of autoimmune and inflammatory diseases, it may also play an important role in tumor immunity. Lastly, Tregs express CD25 and the forkhead box (FOX)P3 transcription factor in addition to CD4+. They serve to inhibit immune activation, induce self-tolerance under physiologic conditions, and notably expand in response to IL-2. Treg blockade or elimination is an important factor in facilitating an effective antitumor immune response, and therapeutic expansion of Tregs may play a role in controlling graft-versus-host disease (GVHD).
γδ T Cells
γδ T cells represent a small subgroup of circulating T cells that function at the interface of innate and adaptive immunity. Their TCR also undergoes somatic recombination and editing but consists of a γδ heterodimer. This subset may recognize specific antigenic determinants via the TCR or by a TCR independent pathway similar to that of NK cells.
Natural Killer T Cells
Natural killer T cells (NKTs) are a unique subset of T cells with a highly restricted TCR repertoire, allowing recognition of certain glycolipid antigens, such as GD2, in conjunction with the nonclassical MHC-I molecule CD1d. They contribute to antitumor immunity as an early source of cytokine production, resulting in activation of other effector cells. Higher numbers of tumor-infiltrating NKTs have been associated with improved long-term disease-free survival (DFS) in children with stage IV neuroblastoma. NKTs are thought to inhibit tumor growth by targeting tumor-associated macrophages (TAMs) that have been shown to have clinical significance in neuroblastoma, Hodgkin lymphoma (HL), and other malignancies. NKTs are attracted by the chemokine (C-C motif) ligand 2 (CCL2). MYCN , the hallmark of aggressive neuroblastoma, mediates CCL2 repression and therefore reduces NKT infiltration. Via another escape mechanism, TAMs in the neuroblastoma microenvironment produce a different chemokine, CCL20, in response to hypoxia, thereby attracting NKTs into hypoxic traps. IL-15 was shown to protect NKTs from the inhibitory effects of hypoxia and enhances antimetastatic activity of NKTs.
NK Cells
NK cells play a key role in the innate immune response, in an early defense against infection or malignant transformation. NK cells can be phenotypically distinguished from other peripheral blood mononuclear cells because they are CD56+ and lack B-cell (CD19), T-cell (CD3), and monocytic (CD14) markers. Subsets of NK cells also express the FcRs CD16 and CD11b that mediate ADCC and participate in CDC. Contrary to T and B cells, NK-cell receptors do not undergo somatic recombination and do not require recognition of a cognate antigen to mediate cytotoxicity. Traditionally it has been thought that they do not develop immunologic memory, although this paradigm may be changing. Their function is tightly regulated by a complex system of activating and inhibitory receptors, which often recognize MHC-I or MHC-I–related molecules. These interactions provide the basis for the therapeutic exploitation of NK-cell alloreactivity. The key regulators of NK cells are activating and inhibitory killer cell immunoglobulin-like receptors (KIRs), which activate natural cytotoxicity receptors including NKp30, NKp46, and NKp44 and the activation receptor NKG2D. NK cells are capable of rapid killing via degranulation and release of toxic granules, primarily through a perforin and granzyme B–dependent pathway. They can also mediate apoptosis by utilizing Fas ligand or TNF-related apoptosis-inducing ligand (TRAIL) and execute ADCC via their FcRIII (CD16) receptor. NK cells further secrete chemokines such as CCL2 through CCL5, XCL1, and CXCL8. Their clinical significance in pediatrics is underscored by the susceptibility of infants born with NK-cell deficiencies to fulminant viral infections and the NK-cell defects underlying primary hemophagocytic lymphohistiocytosis. NK cells facilitate stem cell engraftment, fight infection, and control cancer, yet they do not cause GVHD. These characteristics make them attractive therapeutic candidates for adoptive NK-cell therapies.
Dendritic Cells
Dendritic cells (DCs) represent a small subset of peripheral blood mononuclear cells (1% to 2%). They are mobile sentinels that collect antigen from peripheral tissues and carry it to the secondary lymphoid organs to activate T cells. DCs are termed “professional APCs” based on their ability to process and present antigenic peptides on MHC-I or MHC-II molecules. In steady state, they reside in the periphery in an immature state and play an important role in maintaining tolerance by taking up self-antigens. Successful T-cell activation requires DC activation. Upon activation, DCs upregulate MHC molecules, costimulatory molecules (which provide the second signal for T-cell activation), and chemokine receptors (which enhance homing to lymphoid organs) and secrete inflammatory cytokines such as IL-12. DC activation can occur in response to a variety of signals, such as the receipt of exogenous and endogenous danger signals via Toll-like receptors (TLRs), which recognize evolutionarily conserved molecular patterns from products of damaged or dying cells and from pathogens. DCs are also matured by proinflammatory cytokines secreted by other cells (e.g., innate effectors) or by CD40 ligation. In addition, DCs are important in launching humoral immunity, partly because of their capacity to directly activate B cells.
Myeloid DCs and plasmacytoid DCs are the two main subsets among various other DCs. They express high levels of human leukocyte antigen (HLA)-DR (MHC-II), but they differ in their expression of CD11c (myeloid DCs), CD123 (plasmacytoid DCs), and TLRs, among other markers. DCs are critical regulators of adaptive T- and B-cell responses and are central to the development of successful tumor vaccines. Because of their low abundance in the peripheral blood, sufficient numbers of DCs can be generated in vitro either by expansion of isolated DCs or by generation from monocytes using cytokine cocktails. DCs further play a role in the pathogenesis of GVHD and are required for full graft-versus-leukemia (GVL) effects, and thus their therapeutic manipulation is of relevance in HSCT.
Neutrophils
Neutrophils are crucial to the rapid destruction of pathogens, particularly bacterial, fungal, and certain parasitic organisms, as evidenced by the high risk of sepsis during periods of neutropenia in the oncology population. However, neutrophils also contribute to antitumor activities of the immune system. Through their FcRs, they can be involved in ADCC-mediated tumor destruction. In addition, neutrophil activation at sites of the tumor via administration of cytokines such as GM-CSF can play a role in tumor cell degradation and enhance antigen presentation.
Monocytes/Macrophages
Monocytes are myeloid-derived phagocytic cells that differentiate into resident macrophages upon recruitment into tissues. Macrophages are able to ingest apoptotic cells and opsonized cells via their FcRs. Aside from their role in clearing cellular debris, they are able to present antigenic peptides of the digested cells in an immunogenic way. Activated macrophages prominently secrete cytokines such as IL-12, which may be critical in activating local immune responses by NK cells and T cells. Macrophages were originally classified into M1 (proinflammatory) and M2 (antiinflammatory) macrophages according to their polarization status. Although this classification remains conceptually helpful, it is now clear that tissue macrophages display a high degree of functional and phenotypic plasticity in response to changing microenvironmental stimuli and that M1 and M2 polarization represent extremes of a continuum of activation states. Increasing evidence indicates that TAMs are implicated in protumor effects and can play a role in tumor initiation, progression, and distant metastasis. Limited evidence suggests that myeloid-derived suppressor cells develop from a common myeloid progenitor without a monocytic intermediate and have predefined immunosuppressive properties, whereas monocytes lack suppressive activity in steady state and acquire this function in response to certain stimuli. In persons with cancer, the majority of studies support an association between high TAM density and poor clinical outcome, as has been demonstrated in several malignancies, including neuroblastoma. However, TAM function in tumor immunity appears to be dynamic and heterogeneous depending on the tumor type, tumor progression, location within the tumor, and perhaps most importantly, polarization of the TAM. In this regard, increased density of M2-like TAMs is a marker of poor prognosis, whereas the presence of M1-like subsets is advantageous. Based on these findings, subpopulations of TAMs are now being explored as new therapeutic targets.
Target Antigens in Cancer Immunotherapy
Tumor antigens (TAs) are molecules that enable the recognition of tumors by components of the immune system. A wide array of target antigens have been identified and may be classified into various subgroups according to their origins. Tumor-specific antigens are epitopes that are found exclusively on malignant cells. This group includes proteins arising from mutated oncogenes, clonally rearranged immunoglobulin genes, and oncoviral gene products. Tumor-associated antigens are expressed on some healthy and malignant tissues alike. Tumor-associated antigens may arise from overexpressed normal proteins, oncofetal proteins, lineage-restricted markers, histocompatibility molecules, and proteins that are normally expressed in immune-privileged sites.
Antigens Arising from Genetic Alterations
A number of malignancies are associated with specific genetic mutations or translocations, resulting in the synthesis of novel proteins that are foreign to the host’s immune system, thereby offering the potential of immune recognition while sparing healthy tissues. Commonly they are drivers of or contribute to tumorigenesis, as is the case for oncogenes or mutated tumor suppressor genes, but they may also arise from mutations not directly involved in the neoplastic process. A variety of malignancies express gene products derived from mutated tumor suppressor genes, such as p53 or ras . Examples of antigens derived from mutated oncogenes include the EWS-FLI fusion protein resulting from the t(11 : 22) translocation characteristic of Ewing sarcoma and the p210 and p190 products of the bcr/abl translocation in chronic myelogenous leukemia (CML) and Philadelphia chromosome–positive acute lymphoblastic leukemia (ALL), respectively.
Oncoviral Proteins
Viral infections play a causal role in several malignancies and give rise to immunogenic viral proteins that can be recognized and eliminated by the immune system. This process is underscored by the fact that immunosuppressed persons, particularly those with impairment of T-cell immunity, have a higher propensity to develop Epstein-Barr virus (EBV)–associated lymphomas and human papillomavirus (HPV)–associated skin and cervical cancers. Examples of oncoviral proteins are the expression of the EBV antigens EBNA-1, EBNA-3, LMP-1, and LMP-2 in PTLD and some lymphomas and the papillomavirus E6 and E7 proteins in cervical carcinomas.
Clonally Rearranged Immunoglobulin Genes
Surface immunoglobulin is a marker of B-cell maturity and is present in mature B-cell non-Hodgkin lymphomas (NHLs) and multiple myeloma. Because surface immunoglobulin expression results from separate somatic recombination events in each B cell, all malignant cells in a monoclonal B-cell malignancy express the clonally unique immunoglobulin variable region, or idiotype. Immunoglobulins are proteins and can therefore be immunogenic and lead to the development of idiotype vaccines. Another approach to targeting this group of TAs includes the generation of anti-idiotype mABs in mice, which in turn are immunogenic and amplify the immune response upon administration to the patient as a type of vaccine.
Altered Glycolipid and Glycoprotein Antigens
Most human tumors express higher levels or abnormal forms of surface glycoproteins and glycolipids, which serve as targets for immunotherapy. Some aspects of the malignant phenotype of tumors, including tissue invasion and metastatic behavior, may reflect altered cell surface properties as a result of the abnormal glycolipid and glycoprotein synthesis. Most of these epitopes are not exclusively expressed on tumors but are present at higher levels on cancer cells compared with healthy tissues. Examples of such glycolipids are GD2, which is expressed in neuroblastoma and some osteosarcomas, as well as GM2, GD2, and GD3 in melanomas. These molecules are actively being explored in vaccination strategies, and mABs against the carbohydrate groups or peptide cores of such molecules have been generated and clinically used with success.
Oncofetal Antigens
Oncofetal antigens are proteins that are expressed at high levels in cancer cells and during normal fetal development, but not in adult tissues. Alpha-fetoprotein expression in hepatoblastomas and germ cell tumors and carcinoembryonic antigen in colorectal cancer are examples of aberrant expression of fetal antigens. Although they are largely utilized as biomarkers, they are also being explored as targets of CART therapy.
Products of Genes That Are Selectively Expressed in Immune-Privileged Sites
An interesting group of antigens are the so-called “cancer-testes antigens.” These nonmutated proteins arise from germline-associated genes that are preferentially expressed in immune-privileged sites such as the testes and placenta. These antigens include NY-ESO, MAGE, BAGE, and RAGE and are expressed in melanomas and many carcinomas. They are promising targets given their relatively tumor-specific distribution and have been explored in the context of tumor vaccination.
Histocompatibility Antigens
Histocompatibility antigens are of importance in the allogeneic HSCT setting. Because HLAs are widely expressed in normal tissues and are immunologic targets for T-cell responses, donor selection intends to maximize major histocompatibility matches between the donor and recipient to minimize GVHD. However, minor histocompatibility antigens (mHAs) may generate a GVL response both in matched sibling and in unrelated donor (URD) transplantation. mHAs may have tissue restricted expression, such as HA-1 and HA-2, which are expressed only on hematopoietic cells. Such mHAs are particularly appealing immunologic targets because the induced immunologic response will not cause GVHD in nonexpressing tissues.
Molecules That Activate Recognition of the Innate Immune System
Cells of the innate immune system do not require presentation of antigens via MHC molecules but instead have the ability to respond to MHC-I–related ligands, which tissues may express inducibly in association with cell stress, infection, or malignant transformation, thus allowing for a system in which the body alerts the immune system by creating internal danger signals. Such molecules may not be recognized by endogenous T and B cells but can induce potent responses by innate effector cells. The advantage of such danger signals is that they are absent or expressed at very low levels in steady state. In healthy humans, MICA and MICB, which are ligands for the NKG2D receptor on NK cells and CD8+ T cells, are expressed only by intestinal epithelial cells at low levels, likely as a consequence of stimulation by the local bacterial flora. However, these molecules are significantly upregulated in a variety of tumor types and are thus promising targets.
Normal Proteins Overexpressed in Tumor Tissue
Increased gene transcription or amplification of normal genes in tumor cells may result in aberrantly high expression of proteins. Examples include MYCN amplification in high-risk neuroblastoma, overexpression of human epidermal growth factor receptor 2 (Her2/neu) in a subset of breast and ovarian cancers, as well as some sarcomas, and gp100 and MART in melanomas. Because these proteins are also found at lower levels in some healthy tissues, immune tolerance to such antigens may have developed and needs to be overcome by effective immunotherapy.
Lineage-Specific Tumor Antigens
Many tumors express differentiation antigens that are not restricted to the malignant cell but rather to their lineage of origin. Examples include CD19 and CD22 in pre–B-cell ALL (pre–B-ALL), CD33 in acute myeloid leukemia (AML), and prostate-specific antigen (PSA) in prostate cancer. Similar to overexpressed proteins, the utility of these antigens as targets for tumor immunotherapy is highly dependent on the expression pattern on healthy tissues. As such, the B-cell aplasia accompanying CD19-targeted therapies may be transient and can be managed with exogenous administration of immunoglobulin. Similarly, targeting of PSA may be associated with immune destruction of normal prostate cells, yet this destruction is pathophysiologically tolerable. In contrast, lineage-specific antigen expression on vital organs would be prohibitive to this approach.
Tumor Vaccines
One of medicine’s great successes was the introduction of vaccines for the prevention of infectious diseases. Vaccines that provide protection against oncogenic viruses effectively prevent certain cancers as well. Based on Center for Disease Control and Prevention estimates, approximately 26,000 new cervical cancers each year are attributable to HPV. Therefore the quadrivalent HPV vaccine, which was implemented in 2007 and is recommended for female and male adolescents, has tremendous potential to prevent cervical cancer. Another example is the prevention of hepatocellular carcinoma by way of hepatitis B virus vaccination. The generation of therapeutic cancer vaccines is much more challenging, given that such vaccines must overcome preestablished tumor tolerance. However, the discovery that patients can harbor CD8+ and CD4+ T cells specific for antigens expressed in their tumors gave rise to the idea that therapeutic cancer vaccines could activate and amplify this preexisting reaction and possibly induce new immune responses. Adjuvants such as keyhole limpet hemocyanin (KLH) or Montanide are important components of cancer vaccines because they act to enhance the immunogenicity of the antigen, activate DCs, and help overcome preexisting tolerance.
Dendritic-Cell Vaccines
Because of the central role of DCs in the orchestration of adaptive immune responses, DCs have been a special focus of tumor vaccination strategies. Immature DCs take up antigen and rely on appropriate maturation signals to effectively prime T cells, whereas antigen presentation by immature DCs leads to immune tolerance. It is essential to consider the complexity of DC biology in the context of DC vaccine design. Delivery of the TA to DCs and provision of maturation signals can be provided ex vivo or in vivo. Because of the low frequency of DCs in the peripheral blood, approaches have been developed to generate inflammatory DCs from monocytes under culture conditions with cytokine combinations such as GM-CSF and IL-4 and then pulse them with the desired antigen ex vivo. Such vaccines have been clinically tested for more than a decade, and it has been concluded that they are safe and can induce expansion of circulating tumor-specific CD4+ and CD8+ T cells, with objective and potentially long-lasting responses in some patients. Sipuleucel-T, a vaccine based on enriched blood APCs that are briefly cultured with a fusion protein of prostatic acid phosphatase and GM-CSF, was the first tumor vaccine to be approved by the FDA after it demonstrated a prolonged median survival of patients with metastatic prostate cancer in a randomized phase I trial. In pediatrics, the experience has been limited to a few early nonrandomized trials. Administration of tumor lysate/KLH pulsed autologous DCs to children with relapsed solid tumors was well tolerated and demonstrated induction of specific T-cell responses. Significant regression of metastatic sites was observed in one patient, with several other patients having sustained stable disease. In a related approach, patients with metastatic or relapsed sarcomas or neuroblastomas are receiving Treg-depleted autologous lymphocytes in combination with tumor lysate/KLH pulsed DCs ± IL-7 (trial NCT00923351). An initial study demonstrated that consolidative immunotherapy consisting of adoptive T-cell transfer with peptide-pulsed DCs can improve survival compared with historic control subjects when given in a minimal residual disease (MRD) setting of high-risk solid tumors. An ongoing trial of decitabine followed by vaccination with autologous DCs pulsed with overlapping peptides derived from full-length MAGE-A1, MAGE-A3, and NY-ESO-1 antigens may hold promise in the induction of remission of relapsed neuroblastoma or sarcoma (trial NCT01241162).
DCs can also be targeted in vivo by fusing the selected antigen to an antibody directed against a DC surface receptor. This technique has been shown to elicit potent antigen-specific CD4+ and CD8+ T-cell responses. The choice of a DC receptor that is targeted is an important consideration because distinct DC subsets induce different types of T-cell polarization, which determine in part the elicited immune response. The engagement of DC receptors by such targeting antibodies also provides activation signals, which are crucial to avoid the induction of T-cell anergy. In fact, it is now accepted that the adjuvant components of vaccines (even non–DC-based vaccines) primarily act by triggering DC maturation.
The delivery of antigens and adjuvants into the same intracellular DC compartment has been shown to be crucial. Nanovaccines are a promising preclinical technology to achieve synchronized delivery of antigen and adjuvant cargo to DCs. Nanoscale complexes such as polymer-based or lipid-based nanoparticles provide protection against unwanted antigen degradation or systemic immune activation by soluble adjuvants. Upon injection into the skin, nanovaccines are transported to the lymph nodes by cell-based drainage or free interstitial lymphatic flow (depending on particle size), where they are internalized by lymph node resident DCs and generally directed to the MHC-II pathway. Another bioengineering-based approach is now entering clinical trials and involves implantation of absorbable polymer scaffolds, pulsed with tumor lysate and adjuvants, to facilitate DC-based T-cell priming in situ.
Peptide-Based Vaccines
The immunogenicity of peptide epitopes depends on their associations with specific polymorphic variants of MHC molecules, such that a given peptide might only elicit a response in persons with a certain HLA allele. Studies with HLA-restricted peptides thus must be carried out in patients with unique HLA alleles. Given that the population of pediatric patients is small a priori, the exploration of peptide vaccines has been much more feasible in adults who have common malignancies. Largely as a result of a poor understanding of the mechanisms of immunization and the role of DCs, in many early trials patients were unsuccessfully treated with short peptide-based vaccines in the absence of effective adjuvants. Short peptides have pharmacokinetic properties that make them susceptible to rapid clearance in the absence of an adjuvant that can effectively trigger DC maturation and may promote tolerance rather than immunity. A notable exception is the administration of a short-peptide vaccine derived from gp100 in conjunction with IL-2, which augmented tumor responses and prolonged progression-free survival (PFS) compared with IL-2 alone in persons with advanced melanoma. Longer peptides (~20-mer) require further processing by DCs but have the potential to prime against a greater variety of antigens and capture CD8+ and CD4+ lymphocyte responses more efficiently compared with peptides (10- to 12-mer) that fit the MHC-I antigen-binding groove unedited. A long-peptide vaccine against HPV-16 oncoproteins administered in incomplete Freund adjuvant to patients with vulvar intraepithelial neoplasia proved to have good efficacy, including complete responses in a phase II trial. These favorable results may be based on the selection of viral gene products for vaccination that are more readily recognized as foreign. In contrast, a long-peptide vaccine derived from p53 (a tumor suppressor protein mutated in many cancers), which was delivered in the emulsion-adjuvant Montanide, induced no tumor regressions in patients with advanced ovarian cancer, emphasizing preexisting tolerance and the need to optimize these formulations.
Full-length protein vaccines harbor a wider profile of epitopes, and a diverse set of targets—including two cancer testes antigens, MAGE-A3 and NY-ESO-1—have been explored. Phase I and II trials have been conducted with adjuvant-mixed, recombinant MAGE-A3 vaccines in melanoma and non–small cell lung cancer, demonstrating potent antitumor B- and T-cell responses, tumor-specific cell persistence for years after vaccination, and encouraging clinical effects. “MAGRIT”—an ongoing large, randomized phase III trial accruing more than 2500 HLA-A2–positive patients with non–small cell lung cancer—is exploring a recombinant MAGE-A3 fusion protein in combination with an adjuvant consisting of a saponin/lipid-A emulsion and TLR4 and TLR9 agonists.
Whole-Cell Vaccines
Whole-cell vaccines contain the broadest range of TAs, including mutated proteins. A meta-analysis of 173 immunotherapy trials involving a variety of solid tumors revealed that patients vaccinated with whole-cell vaccines had low objective response rates that were nonetheless significantly higher than those of patients treated with molecularly defined antigen vaccines. GVAX, a vaccine strategy in which autologous or allogeneic whole tumor cells are engineered to secrete GM-CSF (to activate APCs at the site of vaccination) and are irradiated prior to injection, reached the furthest in clinical development. Phase I trials of GVAX were conducted in adults who had a variety of malignancies. These early-stage trials were not powered to assess clinical endpoints, yet they consistently demonstrated immune cell infiltration at the sites of vaccination, as well as extensive tumor necrosis mediated by dense infiltrates of intratumoral CD4+ and CD8+ lymphocytes and plasma cells. Although some patients had promising results after receiving GVAX in preceding trials, a phase III trial in persons with prostate cancer that used allogeneic tumor cell lines did not demonstrate clinical efficacy. This results indicate that this strategy may be most effective when autologous tumor cells are used or when it is combined with other immune-modulating therapies. Administration of anti-CTLA4-mAb to patients previously immunized with GVAX evoked objective responses of metastatic melanoma with minimal toxicities. Furthermore, administration of GVAX early after allogeneic HSCT for adult AML or myelodysplastic syndrome was shown to be safe and immunogenic, with encouraging responses that have led to further exploration in a randomized phase II study (trial NCT01773395). In a similar approach, a pediatric phase I trial with IL-2-transduced autologous neuroblastoma cells in 10 patients resulted in the generation of antitumor antibodies and increased antitumor cytotoxic activity, which correlated well with clinical responses. This study observed one complete response, one partial response, and three patients with stable disease, of whom one patient entered complete remission (CR) after oral etoposide therapy. In a subsequent study of 21 patients treated with an allogeneic neuroblastoma vaccine engineered to secrete lymphotactin and IL-2, two complete responses and one partial response were induced.
Idiotype Vaccines
The clonal immunoglobulin idiotype, which is displayed on the surface of most malignant B cells, is a patient- and tumor-specific antigen that can be used for therapeutic vaccination. An idiotype vaccine conjugated with KLH and administered with GM-CSF indicated prolonged PFS in one phase III trial, whereas several other trials failed to demonstrate a therapeutic benefit.
DNA Vaccines
Deoxyribonucleic acid (DNA) vaccines consist of viral vectors that encode TAs and are based on the idea that strong immune responses against the viral vaccine components enhance the reactivity to the TA. A phase II trial, PROSTVAC, utilized a recombinant vaccinia virus that encoded PSA along with costimulatory and adhesion molecules with the intention of rendering infected cells into surrogate APCs, followed by administration of a similarly configured fowlpox vector in a prime-boost strategy. For additional immune stimulation, GM-CSF was administered with the vectors. This trial demonstrated a median overall survival (OS) benefit of 25.1 months in the treatment group versus 16.6 in the control group and prompted initiation of a phase III trial.
Cytokines
Interferons
IFNs are a class of cytokines that were initially investigated for their ability to interfere with viral infections, and they play an important role in the propagation of an immune response. Clinically meaningful activity of IFN-α has been shown against CML, although its clinical use in CML has largely been abandoned with the advent of tyrosine kinase inhibitors. IFN-α remains a very relevant agent in the adjuvant therapy of high-risk operable melanoma, and high-dose IFN-α has demonstrated a consistent and significant improvement in DFS and OS.
Interleukin-2
IL-2 plays a crucial role in T-cell growth and proliferation. It is primarily produced by CD4+ T cells after antigen stimulation, and to a lesser extent by CD8+ cells, NKT cells, activated DCs, and mast cells. IL-2 transcription is mediated by multiple transcription factors, including the nuclear factor of activated T cells (NF-AT) family proteins, AP-1 and NF-κB. The immunosuppressive drugs cyclosporine and tacrolimus act by blocking NF-AT translocation to the nucleus. The IL-2 receptor (IL-2R) is composed of an α, β, and γ chain. The IL-2R β chain is shared with IL-15, and the common γ chain receptor (γcR) is shared with six other cytokines. The crucial role of IL-2 in T-cell growth is evidenced by the severe combined immunodeficiency phenotype of patients with γcR defects. IL-2 preferentially promotes differentiation of Th1 and Th2 cells while inhibiting Th17 and follicular helper T-cell development, yet it can promote Th17 expansion once polarization has occurred. Importantly, IL-2 also drives the development of Tregs, which promote tolerance, and is essential for activation-induced cell death, an important regulator of T-cell homeostasis. Besides its potent effect on T cells, IL-2 induces proliferation and cytolytic activity of NK cells and promotes B-cell proliferation and antibody production.
Clinical administration of IL-2 in an effort to activate immune cells has been in use for many years, with FDA approval in 1992 for metastatic renal cell carcinoma and in 1998 for metastatic melanoma. In a small subset of 5% to 10% of patients with these malignancies, IL-2 treatment has resulted in long-term CRs, and the addition of adoptive therapy approaches have improved cure rates for metastatic melanoma to a range of 20% to 40%. A limitation of IL-2 is its toxicity, including the propensity for severe capillary leak syndrome.
In pediatrics, a randomized children’s oncology group trial of 289 children with AML in CR1 who received either no further therapy or two short courses of IL-2 after intensive chemotherapy did not show improvement in DFS or OS. However, IL-2 administration plays a role in the treatment of high-risk neuroblastoma, where it is used in conjunction with anti-GD2 mAb therapy, GM-CSF, and isotretinoin, an approach that has led to significant improvements in outcome. The rationale underlying coadministration of IL-2 is the promotion of ADCC via FcRs of IL-2–activated NK cells and macrophages, which demonstrated an enhanced antitumor effect in murine models compared with IL-2 or mAb alone.
As the effects of IL-2 on Tregs have become more clearly delineated, exogenous administration of IL-2 and therapeutic interventions that may prompt high levels of endogenous IL-2 production are being considered carefully. In fact, the tolerance-inducing aspect of IL-2 administration is now being explored for the treatment of GVHD. It has been shown that daily low-dose IL-2 can be safely administered in patients who have chronic GVHD, leading to a preferential and sustained Treg expansion in vivo and ameliorating chronic GVHD symptoms in a substantial portion of patients. Furthermore, IL-2 remains an invaluable tool for the in vitro expansion and preparation of adoptive T-cell therapeutics.
Interleukin-7
IL-7 is produced by nonhematopoietic stromal cells and in small amounts by DCs but is not produced by T cells, B cells, or NK cells. It signals through the IL-7 receptor (IL-7R), a heterodimer consisting of IL-7Rα and the γcR, and mediates antiapoptotic and costimulatory proliferative signals through activation of phosphatidylinositol 3-kinase (P13K) and Janus kinase (JAK) signal transducers and activators of transcription (STAT), as well as antiapoptotic pathways such as B-cell lymphoma–2 (B-CL2). IL-7 is required for normal human T-cell development as demonstrated by the absence of T cells in patients with severe combined immunodeficiency as a result of mutations in the IL-7Rα or γcR, and it may play a role in normal B-cell development. Further, IL-7–mediated signaling in DCs has been implicated as a regulator of peripheral CD4+ T-cell homeostasis. Contrary to typical activation cytokines that do not act on resting T cells, IL-7 primarily affects naive T cells, which then downregulate IL-7R upon T-cell activation. An exception to this rule occurs during primary immune responses when IL-7R expression is maintained on a small subset of T cells destined to enter the central memory pool. Whereas IL-7 is continuously available in secondary lymphoid organs, plasma IL-7 is a limited resource and increases in lymphopenic states as a result of decreased use, thereby enhancing T-cell proliferation. In preclinical models, IL-7 therapy augments antigen-specific T-cell responses after vaccination and adoptive cell therapy. It also facilitates immune reconstitution in lymphopenic settings, largely via thymus-independent homeostatic expansion of peripheral T-cell populations, and has been implicated in antagonizing inhibitory networks.
In light of the preclinical promise, several clinical trials are using recombinant human IL-7 (rhIL-7) to treat patients with malignancies, chronic viral infections, and idiopathic CD4+ lymphopenia, as well as after HSCT. In the first adult human trial of patients with refractory malignancies, rhIL-7 was well tolerated apart from mild toxicities. After an initial decline in the numbers of circulating lymphocytes, IL-7 showed consistent biologic activity with substantial increases of CD8+ and CD4+ cells in the peripheral blood, as well as in spleen and lymph nodes, whereas proliferation rates of Tregs inversely correlated with IL-7 treatment. Notably, rhIL-7 preferentially induced the nonclonal proliferation of naive T cells, resulting in broadening of the TCR repertoire diversity without increasing thymopoiesis. However, in this subset of highly refractory patients, no significant antitumor effects were observed. The first trial that used rhIL-7 after allogeneic HSCT was performed in recipients of T-cell–depleted grafts, given that IL-7 had boosted alloreactive T cells and GVHD in murine models. IL-7 was well tolerated, with only one case of acute GVHD of the skin. In contrast to other clinical scenarios, IL-7 in the posttransplant setting preferentially expanded effector memory T cells rather than naive T cells but increased TCR diversity and functional viral-specific T cells. Further studies are needed to determine whether these effects translate clinically into reduced morbidity and mortality and protection from infection. In pediatric sarcomas, IL-7 therapy is currently being explored in the context of DC vaccination (trial NCT00923351).
Interleukin-15
IL-15 was discovered in 1994 and has pleiotropic immune-enhancing properties. It is thought to be critical for the stimulation and differentiation of NK cells, NKTs, and memory CD8+ T cells. It also promotes proliferation and differentiation of CD4+ T cells, nonmemory CD8+ T cells, and B cells; it further enhances cytolytic activity of CD8+ T cells and induces DC maturation and immunoglobulin synthesis by B cells. Although IL-15 messenger ribonucleic acid (mRNA) is expressed by a large number of tissues, the detection of IL-15 protein is largely limited to DCs and monocytes/macrophages. Free soluble IL-15 is limited, indicating tight regulation of this proinflammatory cytokine. The composition of the heterotrimeric IL-15 receptor (IL-15R) and the main signaling mechanism of IL-15 via presentation in trans are unique. IL-15 shares the β chain (IL-2Rβ/IL-15Rβ) and γcR chain with the IL-2R and signals through these domains via the JAK1/JAK3/STAT5 signaling pathway. Contrary to IL-2 signaling, where the IL-2Rα domain assembles with the IL-2Rβ and γc subunits on the recipient cell, the IL-15Rα domain is located on APCs, binds IL-15 with high affinity, and then presents IL-15Rα–bound IL-15 to the IL-15Rβ/γcR heterodimer on NK or T cells in trans. IL-15 is also thought to have minor signaling pathways that involve direct binding to the IL-15Rβ/γcR as well as binding to IL-15Rα on the target cell and presentation in cis to the IL-15Rβ/γcR on the same target cell. Unlike IL-2, IL-15 does not stimulate immunosuppressive Tregs and has an antiapoptotic effect on T cells. Preclinical and clinical data suggest a dual role for IL-15. Through the link between inflammation and cancer, it has been suggested that IL-15 plays a proleukemic role in hematologic malignancies. In adults with pre–B-ALL, IL-15 expression was associated with an inferior 5-year relapse-free survival (RFS), as well as mediastinal and lymph node involvement. In children without central nervous system involvement at diagnosis, high IL-15 expression levels were associated with a higher risk of central nervous system relapse. A phase I clinical trial blocking IL-15 presentation in trans for patients with T-cell large granular leukemia was well tolerated but showed no responses. On the other hand, evidence for a protective role of IL-15 through enhanced tumor immunosurveillance is provided with the antitumor effects of IL-15 in several preclinical tumor models. Ongoing phase I trials for adults with advanced melanoma are investigating the administration of rhIL-15, either alone or after transfer of tumor-infiltrating lymphocytes (TILs; trials NCT01021059 and NCT01369888). IL-15 is also used for ex vivo preparation of cellular immunotherapies such as in a pediatric trial of haploidentical HSCT and IL-15–stimulated NK cells for refractory solid tumors (trial NCT01337544).
Antibody-Based Immunotherapy
The specific recognition of surface antigens on pathogens or tumor cells was first conceptualized by Paul Ehrlich more than a century ago. In contrast to cellular immunotherapy, which often requires costly and individualized preparation, antibody-based immunotherapy is feasible for off-the-shelf use and thus is more cost effective. Antibody-based therapy may rely on recognition of TAs or interfere with tumor cell growth by disrupting negative regulatory and survival pathways as well as ligand receptor–dependent cell growth. Unconjugated antibodies predominantly function by recruiting other immune effector cells and initiating pathways such as ADCC, CDC, ADP, and direct apoptosis. Via the ADP pathway, antibodies entice APCs to take up not only the targeted antigen but also a variety of tumor contents that are processed to induce T-cell responses in MHC-restricted fashion. This effect is termed the “vaccinal effect” of antibody therapy because it promotes not only immediate tumor cell death, but also vaccinelike antitumor effects with ensuing humoral and cellular immunologic memory. Clinically this effect has been shown to be relevant in solid tumors and lymphomas. Although conventional antibodies activate innate effector mechanisms, they do not trigger T-cell immunity directly. The development of bispecific antibodies represents a promising approach to recruit and activate T cells directly at the site of the tumor cell by providing specificity not only to the TA but also to CD3 ( Fig. 47-5 ).

Unconjugated Antibodies
Rituximab, a genetically engineered chimeric mouse antihuman CD20 mAb, was the first mAb to be approved by the FDA for the treatment of cancer in 1997. The murine Fab domain of rituximab binds CD20 on mature B-cell malignancies and healthy B cells, and the humanized Fc domain mediates effector functions. The mechanism of cytotoxicity is primarily via ADCC and CDC, although rituximab has also been shown to sensitize resistant lymphoma cells to chemotherapy and Fas ligand–induced apoptosis, which led to its synergistic use with chemotherapy regimens in clinical practice. It may also have a vaccinal effect, leading to T-cell priming and lasting antilymphoma immunity. Rituximab is now a well-established component of therapeutic regimens for adult CD20+ NHL and chronic lymphocytic leukemia (CLL) in the United States and Europe. CD20 is expressed by almost all cases of pediatric B-cell NHL, yet clinical experience with rituximab in pediatric lymphomas is still limited and in evolution. Pediatric randomized controlled trials of antibody therapy for B-cell lymphoma have not been published, but a limited number of phase I and II studies suggest a low incidence of adverse effects and encouraging response rates. In a study of 38 children with stage III/IV B-cell NHL who were treated with a combination of FAB/LMB96 chemotherapy and rituximab, the 3-year event-free survival (EFS) and OS was 95%. As rituximab is studied further in the pediatric population, it may improve outcomes both for newly diagnosed patients and patients who have had a relapse or have refractory disease, and facilitate chemotherapy regimens with reduced toxicity. Rituximab further finds application in the treatment of PTLD, either as a single agent or in combination with low-dose chemotherapy. Isolated reports also indicate its utility in the treatment of pediatric paraneoplastic syndromes. It should be emphasized that rituximab temporarily depletes healthy B cells, and thus patients may require immunoglobulin replacement.
Specific to the treatment of pediatric high-risk neuroblastoma is a highly effective unconjugated mAb against the disialoganglioside GD2. When immunotherapy (consisting of ch14.18 anti-GD2 mAb in conjunction with IL-2 and GM-CSF) was administered with isotretinoin after standard intensive multimodal therapy in a phase III trial, 2-year EFS increased dramatically to 66% versus 46% and OS increased to 86% versus 75%.
Also noteworthy is trastuzumab, an antibody against HER2, which is approved by the FDA for the treatment of HER2-overexpressing breast cancer and gastric adenocarcinoma.
Bispecific Antibodies
Staerz and colleagues first introduced the concept of bispecific antibodies to engage CTLs for cancer cell lysis. Such antibodies have dual specificity for both CD3 and the TA of interest, thereby bringing CTLs in close proximity to the tumor cell and facilitating the killing of tumor cells, regardless of the T-cell’s TCR specificity. Increasingly sophisticated constructs have been developed with time. Bispecific T-cell engagers (BiTEs) can be distinguished from other bispecific constructs by their high potency at picomolar range and support of serial lysis at low effector-to-target ratios. BiTEs against multiple different target antigens including CD19, GD2, epithelial cell adhesion molecule (EpCAM), Her2/neu, epidermal growth factor receptor (EGFR), carcinoembryonic antigen (CEA), CD33, ephrin type A receptor 2 (EphA2), and melanoma-associated chondroitin sulfate proteoglycan (MCSP) have been constructed. Conventional EGFR antibodies such as cetuximab can mediate an ADCC effect via FcRs but appear to act predominantly by blocking EGFR, based on analyses showing that patients with downstream mutations in KRAS and BRAF genes do not have an overall survival advantage. EGFR-BiTE antibodies, however, can activate T cells via CD3 when engaging a tumor cell and have been shown to lyse even KRAS-mutated colorectal cancer cell lines. EpCAM is frequently expressed on human adenocarcinomas and some squamous cell carcinomas, but also on cancer stem cells. MT110, an EpCAM BiTE, is being tested in a phase I trial for lung, gastrointestinal (GI), breast, ovarian, and prostate cancers (trial NCT00635596). Blinatumomab, a CD19 BiTE that targets B-cell malignancies, first demonstrated efficacy in persons with advanced NHL and has shown promising phase II results as a single agent in adult patients with ALL who have persistence or recurrence of MRD, leading to a 80% response rate with elimination of MRD and a 61% hematologic RFS at a median follow-up of 33 months. Of note, some but not all of the patients who experienced a relapse had CD19-negative relapses. As expected, blinatumomab treatment resulted in B-cell depletion, but interestingly, it also resulted in a transient decline followed by a significant expansion of T cells bearing the early activation marker CD69. CD4+ T cells expanded more profoundly than did CD8+ T cells, but Treg numbers generally remained low. A single-arm phase II multicenter international trial is currently evaluating blinatumomab in pediatric and adolescent patients with relapsed or refractory ALL (trial NCT01471782). In the first reported pediatric experience of compassionate blinatumomab use in patients with refractory ALL who had experienced multiple relapses after HSCT, all three patients were able to reach MRD-negative CRs, although only one patient who was able to undergo subsequent HSCT had a lasting remission. From a practical standpoint, blinatumomab is administered as a continuous 24-hour infusion for 4 weeks per 6-week cycle via a portable mini pump. The most prominent adverse effects of blinatumomab include lymphopenia, pyrexia, hypogammaglobulinemia, and hypokalemia, but reversible neurologic toxicities such as seizures and ataxia have been reported. Other BiTEs such as a CD33/CD3 BiTE for AML and BiTEs engaging NK cells via CD16 are still in preclinical development.
In yet another iteration, Triomab antibodies are specific for the TA and engage T cells via CD3, but they also recruit NK cells and macrophages via their FcR. Catumaxomab, a Triomab antibody against EpCAM, was approved in Europe in 2009 for the treatment of malignant ascites in a variety of EpCAM+ abdominal tumors, following its success in a phase II/III clinical trial. Other Triomab antibodies are in phase I/II adult clinical trials, such as a CD20 Triomab antibody in the therapy of B-cell lymphoma in conjunction with donor lymphocyte infusion (DLI) after allogeneic HSCT (trial NCT01138579) and ertumaxomab, a Triomab antibody against HER2/neu+ advanced solid tumors (trial NCT01569412). An anti-GD2 Triomab antibody has thus far been tested in animal models of neuroblastoma.
Immunoconjugates
Various immunoconjugates have emerged from the idea that mABs against TAs could be exploited to deliver cytotoxic agents directly to the tumor cell site and minimize systemic adverse effects. In this approach, mABs are linked to radionuclides (radioimmunotherapies), drugs (antibody-drug conjugates, or ADCs), toxins (immunotoxins), enzymes (antibody-directed enzyme prodrug therapy), and cytokines (immunocytokines) to boost the cytotoxic mAb effects on target cells. Two radioimmunotherapies targeting CD20 have been approved by the FDA for adults. Yttrium-90 ibritumomab tiuxetan, approved in 2002, is used in the therapy of follicular NHL or relapsed/refractory low-grade NHL. Iodine-131 tositumomab was approved by the FDA in 2003 and is now indicated in the treatment of CD20+ relapsed or refractory follicular or transformed NHL.
A very promising recent ADC, brentuximab vedotin, carries the antimitotic drug monomethyl auristatin E and targets CD30, leading to tumor cell apoptosis. It has shown remarkable efficacy in adults with relapsed or refractory HL or anaplastic large-cell lymphoma (ALCL). These results led to accelerated FDA approval for brentuximab in 2011 for the treatment of HL and ALCL; it was the first new drug to be approved for HL in more than 30 years. Brentuximab has also been used successfully prior to allogeneic HSCT, as well as for relapse after allogeneic HSCT. Its efficacy in other CD30+ adult malignancies is being tested in the phase II trials NCT01461538 and NCT01421667, and several clinical trials are being conducted to evaluate its safety and efficacy in conjunction with combination chemotherapy as a frontline agent (e.g., trial NCT01060904). In the pediatric population, brentuximab is currently being explored in a phase I/II trial for relapsed or refractory ALCL and HL (trial NCT01492088), but successful single-agent remission induction in a pediatric patient with ALCL who has experienced multiple relapses has been reported. The Children’s Oncology Group is planning to evaluate inclusion of brentuximab in the upfront therapy of newly diagnosed patients with classic HL across all risk strata in an effort to improve initial response and minimize radiotherapy, as well as to evaluate administration of brentuximab in conjunction with gemcitabine for relapsed/refractory HL. Cooperative groups are also pursuing evaluation of brentuximab vedotin in combination with standard chemotherapy in children with ALCL. Based on CD30 expression in patients with GVHD, brentuximab is also being investigated as an agent to treat and prevent GVHD (trials NCT01596218 and NCT 01700751).
Trastuzumab emtansine most recently gained FDA approval in 2013 for the treatment of Her2+ metastatic breast cancer. This ADC combines the Her2-targeting antitumor properties of the mAb trastuzumab with the cytotoxicity of the microtubule inhibitory agent DM1, a derivative of maytansine. In a randomized international phase III trial it showed significantly prolonged PFS with less toxicity compared with a standard chemotherapeutic regimen and further compared favorably to trastuzumab and docetaxel in a phase II trial of patients with metastatic breast cancer who had progressed after receiving Her2-targeted therapy with trastuzumab. This drug has not been evaluated in children but may hold promise, given reports of Her2 expression in subgroups of patients with osteosarcoma, Ewing family tumors, neuroblastic tumors, and some Wilms tumors.
Gemtuzumab ozogamicin (GO) is a humanized anti-CD33 mAb conjugated with the anthracycline calicheamicin. It targets CD33, which is expressed in more than 80% of patients with AML. In phase II results of adults older than 60 years who had their first relapse, GO as a single agent was associated with a 30% overall response rate, leading to accelerated FDA approval in 2000. Other randomized phase II trials comparing GO with induction chemotherapy and chemotherapy alone confirmed these results. However, a large phase III study evaluating the effects of GO in combination with chemotherapy versus standard induction therapy in adults younger than 60 years with newly diagnosed AML failed to show superiority of the GO arm with respect to overall efficacy, RFS, and OS and demonstrated increased induction mortality in the GO arm. Based on results from this study and concerns about high frequencies of hematologic and liver toxicity, approval for GO was voluntarily withdrawn in 2010. Since then, resurrection of GO has been widely discussed, citing the exceptionally low induction mortality of 1% in the control arm of the aforementioned study and typical induction mortality of 5% in the GO arm, as well as a lower dose of daunorubicin in the GO arm, complicating direct comparison of both groups. Several other large randomized trials in adults with newly diagnosed AML have shown no difference in remission rates but a significantly higher EFS and OS in patients receiving GO in conjunction with chemotherapy, with particular benefit for younger adults who have favorable cytogenetics. Furthermore, trials using lower dose GO either at single or fractionated dosing no longer showed an increase in hepatotoxicity or veno-occlusive disease. In pediatric patients, an increased incidence of veno-occlusive disease was also limited to high-dose GO, and a phase I study of GO in combination with busulfan and cyclophosphamide conditioning for 12 children with CD33+ AML showed no GO-associated dose-limiting toxicities. CD33 expression in childhood AML is heterogeneous, but high CD33 expression is associated with adverse disease characteristics such as fms-related tyrosine kinase 3 internal tandem duplication (FLT3-ITD) and independently predicts poor outcome. Initial phase I and compassionate use data suggested efficacy of GO monotherapy in some patients, and subsequent studies established that combination of GO with chemotherapy was well tolerated in the pediatric population. In a study that administered chemotherapy in combination with GO to patients with poor end-induction MRD responses, 27 of 29 patients had a reduction in MRD and 13 of 29 patients became MRD negative, including 4 of 8 patients who had MRD levels greater than 25%. However, no published pediatric trials have compared GO with chemotherapy versus chemotherapy alone in a randomized fashion.
Inotuzumab ozogamicin is a calicheamicin-linked mAb directed against CD22, a marker that is highly expressed in ALL. It has been evaluated in a phase II trial for relapsed or refractory adult ALL with promising responses and in a phase I/II trial for adults with relapsed NHL, where it showed high response rates and PFS in combination with rituximab. It is currently undergoing further evaluation in several adult trials. No pediatric clinical trials have been conducted thus far, although preclinical studies suggest sensitivity of pediatric B-ALL blasts to inotuzumab ozogamicin in vitro.
Within the group of immunotoxins, BL22 is an antibody that is bound to a modified Pseudomonas exotoxin and directed against CD22 . A phase I trial of BL22 in pediatric patients with relapsed or refractory CD22+ ALL or NHL had an acceptable safety profile but did not show any responses, leading to an ongoing phase I trial of a second-generation antibody, CAT-8015, with improved binding affinity and in vitro cytotoxicity (trial NCT00659425). BL22 has shown promise in phase II trials for the treatment of hairy cell leukemia in adults, and CAT-8015 is also being investigated in a phase I trial. Neutralizing antibody responses to BL22 have been documented in some patients and present an obstacle to retreatment.
Antibodies in the Modulation of Regulatory Pathways
During a physiologic T-cell response, the balance between costimulatory and inhibitory signals (so-called immune checkpoints) is critical to allow for efficient T-cell activation while limiting the duration and amplitude of T-cell responses, thereby protecting against chronic inflammation and autoimmunity. CTLA-4 and PD-1 are examples of inhibitory receptors that are upregulated by T cells during the course of an immune response and inhibit T cells upon binding to their respective ligands. In the process of immunoediting, tumor cells or nontransformed cells in the tumor microenvironment express inhibitory ligands to escape immune surveillance. However, given that many of these immune checkpoints are mediated by ligand-receptor interactions, they lend themselves to therapeutic blockade with mABs. In contrast to TA-specific mABs, such mABs do not target the tumor but rather target lymphocyte receptors or their ligands to unleash intrinsic antitumor activity.
CTLA-4 was the first inhibitory receptor to be clinically targeted. CTLA-4 is upregulated on activated T cells, where it dampens T-cell activation upon engagement with the B7 (CD80/CD86) receptors on DCs. Although expressed by CD8+ T cells, its major impact is the inhibition of CD4+ helper T cells and enhancement of the activity of Tregs, which express CTLA-4 constitutively. Although the exact mechanism by which CTLA-4 enhances Treg function is not known, numerous studies have documented that the ratio of effector T cells to Tregs in the tumor microenvironment plays a crucial role in the control of tumors and correlates with outcome. After demonstration of significant tumor regressions by single-agent anti-CTLA-4 mAb in conjunction with GVAX by Leach et al. and van Elsas et al., two fully humanized anti-CTLA-4 mABs, ipilimumab and tremelimumab, entered phase I clinical trials in 2000. These mABs demonstrated ~10% objective response rates in patients with refractory metastatic melanoma who received either GVAX or gp100 peptide vaccines. The initial phase III trial with tremelimumab plus dacarbazine compared with dacarbazine alone did not show benefit at the dose used, but a later trial comparing ipilimumab plus dacarbazine versus dacarbazine plus placebo showed improved outcomes with ipilimumab. After a phase III trial with ipilimumab alone or combined with a gp100 peptide vaccine versus gp100 alone, which demonstrated a survival benefit in both ipilimumab groups, ipilimumab was approved in 2011 by the FDA for use in persons with advanced melanoma. Up to 30% of patients may experience severe immune-related adverse effects (the most common being colitis) with ipilimumab, which modestly correlate with response. Steroids and TNF blockade have been used in clinical management; some adverse effects such as hypophysitis may be long lasting or irreversible, although they are readily managed with hormone replacement therapy. Trials in several adult malignancies are assessing Ipilimumab alone or in combination regimens such as with chemotherapy (trials NCT01331525 and NCT01473940), in combination with GM-CSF (trial NCT 01530984), or with androgen ablation in persons with prostate cancer (trial NCT 01377389). The necessity for rigorous evaluation of novel combination regimens is highlighted by significant hepatotoxicity observed during concurrent administration of ipilimumab and the BRAF inhibitor vemurafenib. In the pediatric population, a first phase I trial is underway to evaluate ipilimumab in the treatment of refractory solid tumors and lymphoma (trial NCT01445379).
The PD-1/PD-L1 axis has been another focus of clinical investigation. PD-1 is expressed by activated T cells, B cells, and NK cells. PD-1 binding to its ligands PD-L1 or PD-L2 (expressed on DCs and in peripheral tissues) inhibits kinases that are involved in T-cell activation. Furthermore, PD-1 is expressed on Tregs, where it enhances proliferation upon PD-1 engagement with its ligand. Whereas CTLA-4 regulates the immune response at the time of T-cell activation in the lymphoid organs, PD-1 primarily limits the activity of T cells in peripheral tissues. PD-1 ligands are upregulated on the tumor cell surface and on myeloid cells in the tumor microenvironment of a variety of human malignancies, where they mediate immune resistance. Studies in mouse models demonstrate enhanced antitumor immunity through blockade of PD-1 or its ligands, while suggesting a favorable safety profile compared with CTLA-4 based on a comparatively mild phenotype of PD-1, PD-L1, and PD-L2 knockout mice. The first phase I trial with a single-dose fully human anti–PD-1 mAb yielded promising results, including one complete response. A subsequent phase I trial evaluating sequential treatment with an anti–PD-1 mAb in adults with a variety of malignancies demonstrated quite durable objective response rates of 36% in patients with PD-L1+ tumors and a safety profile comparable with or better than that of ipilimumab. Investigators of a concurrent phase I trial exploring blockade of PD-L1 rather than PD-1 reported an objective response rate of 6% to 17% depending on the underlying malignancy but did not consider PD-L1 expression in the tumor. Ongoing phase I/II trials are investigating blockade of PD-1/PD-L1 in various malignancies either alone (trial NCT01354431); with ipilimumab (trial NCT01024231); with a gp100, MART-1, and NY-ESO-1 peptide vaccine (trial NCT01176461); or in conjunction with chemotherapeutic agents (trial NCT01313416). Several other immune-checkpoint molecules are emerging as candidates for therapeutic blockade, such as lymphocyte-activation gene 3 ( LAG3 ), 2B4, B- and T-lymphocyte attenuator (BTLA), T-cell immunoglobulin and mucin domain 3 (TIM3), A2aR, and KIR. Anti-KIR mABs aim to interfere with the interaction of inhibitory KIR receptors on NK cells and their ligands to create a missing-ligand situation and induce NK-cell–mediated tumor killing. Results from two phase I trials in adults with multiple myeloma and AML have determined safe and tolerable doses at which inhibitory KIR saturation can be achieved, and RFS in patients with AML compared favorably with that in historic control subjects.
The stimulation of activating costimulatory pathways such as 4-1BB and inducible costimulator (ICOS) by way of therapeutic mAb administration is another strategy undergoing evaluation in early trials.
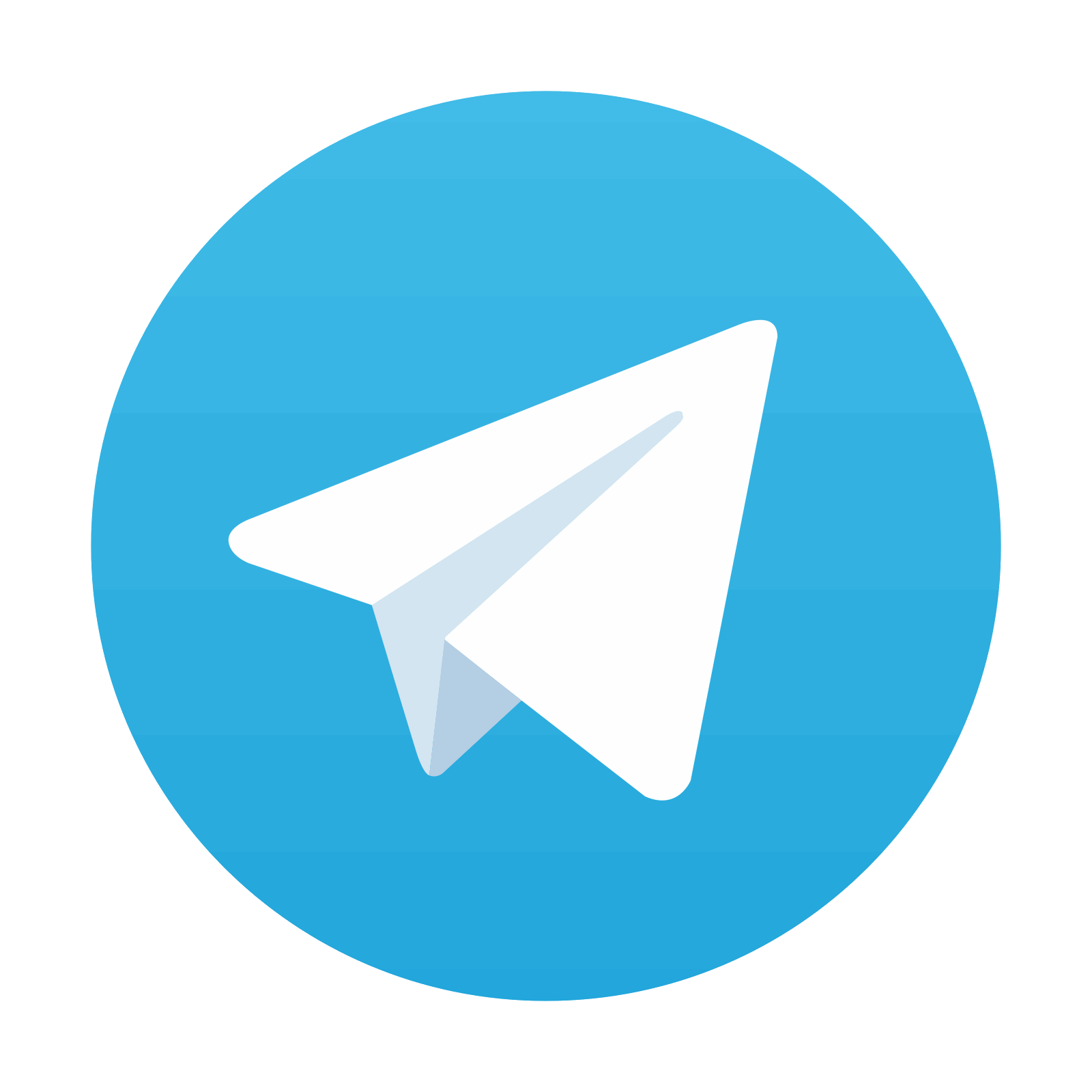
Stay updated, free articles. Join our Telegram channel

Full access? Get Clinical Tree
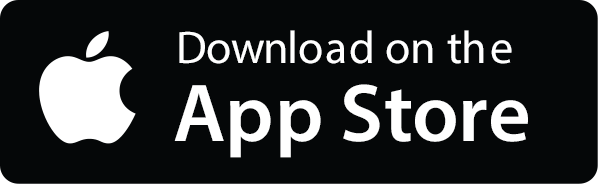
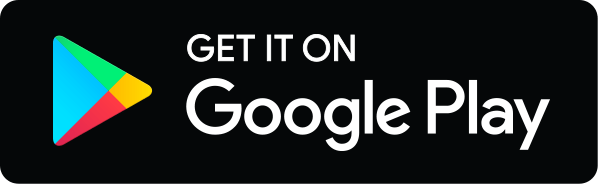