Symptoms
Circumoral and acral tingling paresthesias
Increased neuromuscular irritability
Tetany
Muscle cramps and twitching
Abdominal cramps
Laryngospasm
Bronchospasm
Altered CNS function
Seizures of all types
Papilledema or pseudotumor cerebri
Choreoathetoid movements
Depression
Coma
Congestive heart failure
Generalized fatigue
Signs
Chvostek’s sign
Trousseau’s sign
Prolongation of QTc interval
Cataracts
Basal ganglia and other intracerebral calcifications
More severe hypocalcemia may cause bronchospasm, laryngospasm, seizures, cardiac dysrhythmias associated with QT interval prolongation, coma, or sudden death. Head CT or X-ray imaging may demonstrate calcification of the basal ganglia and other intracerebral structures. Patients may develop cataracts related to long-standing treatment-related increases in the calcium x phosphate product, or pseudotumor cerebri. Prolonged hypocalcemia may cause congestive heart failure due to cardiomyopathy, which may reverse with appropriate management of hypocalcemia. Patients often report feeling weak, fatigued, or depressed until hypocalcemia is corrected.
Differential Diagnosis
Causes of hypocalcemia may be broadly divided into PTH-related and non-PTH-related causes (Table 21.2). PTH-related hypocalcemia is most often due to PTH deficiency or resistance. A large portion of non-PTH-related causes are due to vitamin D deficiency or resistance, with a wide variety of other less common causes. This section briefly reviews the multiple causes of hypocalcemia, and gives several case illustrations of different causes.
Table 21.2
Causes of hypocalcemia
PTH related |
Acquired hypoparathyroidism |
Postsurgical |
Infiltration with iron |
Hemochromatosis |
Thalassemia with repeated transfusions |
Infiltration with copper: Wilson’s disease |
Parathyroid metastases |
Neck radiation therapy |
Hypomagnesemia or hypermagnesemia |
Autoimmunity: Parathyroid gland antibodies |
Congenital or inherited hypoparathyroidism |
APECED syndrome |
Autosomal dominant hypocalcemia |
Isolated hypoparathyroidism: Familial or X-linked |
Parathyroid agenesis |
Mutations in PTH gene |
Syndromes associated with hypoparathyroidism: DiGeorge syndrome, mitochondrial disorders with hypoparathyroidism |
PTH resistance |
Pseudohypoparathyroidism types 1a, 1b, 1c, and 2 |
Hypomagnesemia |
Vitamin D related |
Vitamin D deficiency: |
Nutritional deficiency |
Malabsorption |
Lack of adequate sunlight exposure |
Hyperpigmentation |
Anticonvulsant therapy |
Pseudovitamin D deficiency rickets (vitamin D-dependent rickets type 1) |
Chronic renal disease |
Severe liver disease |
Vitamin D resistance |
Hereditary vitamin D-resistant rickets (vitamin D-dependent rickets type 2) |
Others |
Hyperphosphatemia |
Chronic renal failure |
Tumor lysis syndrome |
Rhabdomyolysis |
Acute pancreatitis |
Burns |
Hungry bone syndrome |
Osteoblastic bone metastasis |
Transfusion with citrated blood products |
Critical illness |
Pseudohypocalcemia: Gadolinium-based contrast agents: gadodiamide and gadoversetamide B |
Medications |
Hypocalcemia with decreased PTH levels |
Drug-induced hypomagnesemia: Cisplatin, diuretics, aminoglycosides, amphotericin |
Drug-induced hypermagnesemia: Magnesium-containing antacids or laxatives, tocolytic therapy |
Cinacalcet |
Alcohol abuse |
Hypocalcemia with increased PTH levels |
Calcium-chelating agents: EDTA, citrate, foscarnet, hydrofluoric acid |
Vitamin D deficiency or resistance: Phenytoin, phenobarbital, carbamazepine, valproate, isoniazid, theophylline, glutethimide, rifampicin |
Skeletal antiresorptive agents: Bisphosphonates, denosumab, estrogens, raloxifene, calcitonin, plicamycin, colchicine overdose |
Loop diuretics |
PPIs and H2-blockers |
Glucocorticoid therapy |
Others: |
Propylthiouracil (PTU) |
Dobutamine |
Calcium channel blockers |
Strontium-89 |
Deferasirox |
Bicarbonate therapy |
Electroconvulsive therapy |
PTH-Mediated Hypocalcemia
Hypoparathyroidism
By far the most common cause of hypoparathyroidism in adults is postsurgical hypoparathyroidism [7]. Postsurgical hypoparathyroidism occurs after neck surgery, but not only due to surgery targeting the parathyroid glands. The parathyroid glands may be adversely affected by compromised blood supply after manipulation during surgery on other neck structures, or by inadvertent removal. Postsurgical hypoparathyroidism usually results in hypocalcemia within 24–48 h after surgery, and is usually temporary. Different centers have reported different rates of symptomatic hypoparathyroidism after thyroid cancer surgery, ranging from 1 to 46 % of cases [8], whereas long-term postsurgical hypoparathyroidism is usually limited to less than 1–2 % of cases, depending on surgical expertise. The rate of post-thyroidectomy hypoparathyroidism increases with the stage of thyroid cancer, and is dependent on the extent of surgery, with about half of stage IV patients suffering from postsurgical hypoparathyroidism.
Illustration: Case 1
A 29-year-old female was referred for post-thyroidectomy tetany. She had undergone surgery for a benign multinodular goiter, without evidence of malignancy at pathology review. The morning after surgery, her serum total calcium was decreased at 5.8 mg/dL (normal, 8.9–10.1), serum phosphorus mildly increased at 4.8 mg/dL (normal, 2.5–4.5), and serum creatinine normal at 0.9 mg/dL (normal, 0.8–1.3). Her serum 25-hydroxyvitamin D was normal at 38 ng/mL (optimal, 20–50 ng/mL). Her serum parathyroid hormone was undetectable at <6 pg/mL (normal, 15–65). Her serum magnesium was normal at 1.9 mg/dL (normal, 1.7–2.3 mg/dL). These findings indicate that postsurgical hypoparathyroidism was the cause of her hypocalcemia and hyperphosphatemia. Unfortunately about 75 % of adults diagnosed with acquired hypoparathyroidism are postsurgical, with surgery sometimes being done for benign causes such as goiter or primary hyperparathyroidism, but more often for thyroid or other head or neck cancers.
Most patients with postsurgical hypoparathyroidism have transient hypoparathyroidism. Experience of the surgeon performing surgery generally predicts the incidence of postsurgical hypoparathyroidism. Immediate postoperative PTH levels may be useful in predicting which patients will develop permanent hypocalcemia due to postsurgical hypoparathyroidism [9].
Nonsurgical hypoparathyroidism may be due to deficiency or excess of serum magnesium [10, 11]. Hypomagnesemia usually causes hypoparathyroidism when serum magnesium is less than 1.0 mg/dL. Up to 11 % of hospitalized patients may have hypomagnesemia, while up to 9 % may have hypermagnesemia [12]. Hypomagnesemia may be due to gastrointestinal losses associated with vomiting related to excessive alcohol intake, chronic diarrhea, steatorrhea, malabsorption, or intestinal resection; renal tubular losses due to medications such as furosemide, aminoglycosides, cisplatin, cyclosporin, amphotericin B, pentamidine, tacrolimus, or proton pump inhibitors [13]; or rare genetic disorders such as Gitelman syndrome. Hypomagnesemia occurs frequently in critically ill patients, which contributes to the hypocalcemia frequently seen in intensive care unit patients. Hypermagnesemia may occur in the setting of late-stage chronic kidney disease in patients treated with magnesium antacids, enemas, or infusions, or acute renal failure associated with rhabdomyolysis or tumor lysis syndrome [14].
Illustration: Case 2
A 48-year-old male was referred for possible hypoparathyroidism. His serum calcium was decreased at 6.7 mg/dL (normal, 8.9–10.1 mg/dL), with serum phosphorus increased at 5.5 mg/dL (normal, 2.5–4.5 mg/dL), and serum creatinine normal at 1.3 mg/dL (normal, 0.8–1.3 mg/dL). His serum 25-hydroxyvitamin D was normal at 45 ng/mL (optimal, 20–50 ng/mL). His serum PTH was decreased at 8 pg/mL (normal, 15–65 pg/mL). His serum magnesium was very low at 0.8 mg/dL (normal, 1.7–2.3 mg/dL). These findings indicate that significant magnesium deficiency was the primary cause of his hypoparathyroidism leading to hypocalcemia and hyperphosphatemia. Further evaluation demonstrated renal tubular magnesium wasting due to previous use of outdated aminoglycoside antibiotics.
Primary intestinal hypomagnesemia results from a rare inherited disorder causing magnesium malabsorption leading to hypomagnesemia in early infancy. This condition is thought to primarily occur due to deficient intestinal magnesium absorption, but there may also be defects in renal magnesium reabsorption. Patients usually present with neurological symptoms, including tetany, muscle spasms, and seizures due to both hypomagnesemia and hypocalcemia associated with hypoparathyroidism. Lifelong high oral intake of magnesium supplements decreases symptoms and restores serum calcium levels to normal. Mutations in the TRMP6 gene on chromosome 9 have been identified to cause this disorder [15, 16]. The TRMP6 protein is a member of the transient receptor membrane potential channel family that complexes to TRPM7, a calcium- and magnesium-permeable cation channel.
Other acquired causes of hypoparathyroidism are much rarer. Infiltration and destruction of the parathyroid glands by iron overload may occur in hemochromatosis, or thalassemia requiring multiple blood transfusions [17]. Copper overload occurring due to Wilson’s disease may also result in hypoparathyroidism [18].
Hypoparathyroidism may result from metastases to the parathyroid glands in extremely rare circumstances [19]. External beam radiation therapy to the neck for treatment of malignant disease in this region, or radioactive iodine therapy for Graves’ disease, may also rarely result in destruction of the parathyroid glands [20].
Inherited causes of hypoparathyroidism include autosomal dominant hypocalcemia (ADH), a condition in which there is a gain-of-function mutation in the CaSR [21]. This type of mutation changes the threshold of PTH secretion by parathyroid cells in response to circulating ionized calcium, leading to low or inappropriately normal PTH secretion despite hypocalcemia. Most of the mutations reported to date affect the extracellular amino-terminal or transmembrane domains of the receptor. The mutant receptors may show both increased receptor sensitivity to calcium and increased maximal signal transduction capacity.
Since this activating mutation is also expressed in the CaSR on proximal renal tubular cells in the thick ascending limb of Henle, absolute or relatively increased 24-h urinary calcium excretion is a hallmark of the disorder. Most patients with ADH are asymptomatic and have mild hypocalcemia with significant hypercalciuria, but occasional patients may present with moderate or severe hypocalcemia. This form of CaSR-mediated hypoparathyroidism may cause increased risk of nephrocalcinosis compared to other forms of hypoparathyroidism. In one series, almost half of the patients evaluated had nephrocalcinosis associated with hypercalciuria [22]. Calcium supplementation must therefore be monitored carefully in this condition.
Occasional reports have described patients with CaSR gain-of-function mutations associated with a Bartter-like syndrome, suggesting that the CaSR may also play a role in sodium chloride regulation [23]. These patients present with hypocalcemia, hypercalciuria, and nephrocalcinosis, associated with hypokalemic alkalosis, renal salt wasting that may cause hypotension, hyperreninemic hyperaldosteronism, and increased urinary prostaglandin excretion. Extensive burns may lead to upregulation of the CaSR, with lower than normal serum calcium suppressing PTH secretion, resulting in hypocalcemia and hypoparathyroidism.
Autoimmune hypoparathyroidism is thought to be the second most common form of acquired hypoparathyroidism. Isolated autoimmune destruction of the parathyroid glands may occur, resulting in idiopathic hypoparathyroidism, or autoimmune destruction may occur in association with other autoimmune conditions as part of autosomal recessive autoimmune polyglandular endocrinopathy candidiasis ectodermal dystrophy (APECED) syndrome [24]. This syndrome is caused by mutations in the autoimmune regulator gene AIRE, which results in abnormal thymic expression of tissue antigens, generation of autoreactive T cells, ultimate loss of central tolerance to specific self-antigens, and the development of multiple autoimmune disorders [25]. Antibodies against the CaSR have been identified in some individuals with both idiopathic hypoparathyroidism or APECED syndrome [26, 27], but it is not yet clear if these antibodies are causative or simply markers of disease [28]. Idiopathic autoimmune hypoparathyroidism most often occurs in the teens or the young adulthood, but may occur at any age. APECED usually presents in childhood, and is characterized by chronic mucocutaneous candidiasis in addition to variable expression of endocrine and other autoimmune diseases. Variation in the clinical phenotype of individuals with identical mutations in the AIRE gene is incompletely understood, but this suggests that other genetic loci or environmental factors are important in the development of the phenotype.
Hypoparathyroidism may be diagnosed at birth or during childhood due to a variety of genetic mutations causing congenital syndromes, the most widely known being the DiGeorge (velocardiofacial) syndrome [29]. This disorder is caused by abnormal development of neural crest cells in the third and fourth branchial pouches. In 90 % of cases the syndrome is caused by heterozygous chromosomal deletion of the TBX1 gene in the region of chromosome 22q11. Thirty-five genes have been identified in this region, so deletion of other genes, alone or in combination, could also cause this syndrome, but the TBX1 gene is a major determinant of cardiac, thymus, and parathyroid cell phenotypes. A region on chromosome 10p (DiGeorge critical region II) has also been linked to the syndrome. DiGeorge syndrome is associated with distinctive facial abnormalities, cleft lip and/or palate, conotruncal cardiac anomalies, and mild-to-moderate immune deficiency. Hypocalcemia due to hypoparathyroidism has been reported in 17–60 % of affected children [30]. DiGeorge syndrome is estimated to occur in as many as 1:2,000–1:3,000 births, with the incidence rate of new mutations estimated at 1:4,000–1:6,000. Because the clinical phenotype varies, findings may be subtle and therefore overlooked, and mild hypocalcemia may be easily missed. In one study of adults with chromosome 22q11.2 deletion, about half were hypocalcemic, with a median age of presentation of 25 years, and a maximum age of diagnosis of up to 48 years [31]. This disorder may rarely be diagnosed for the first time as late as the mid-60s, with late onset of mild hypocalcemia, and is not infrequently diagnosed in an affected parent in the 20s or 30s after birth of an affected child.
Finally, a variety of other rare genetic or inherited disorders may cause hypocalcemia that is recognized in infancy or childhood. Familial isolated hypoparathyroidism due to autosomal recessive or dominant mutations in the pre–proPTH gene on chromosome 11p15 [32, 33], or parathyroid gland dysgenesis due to mutations in various transcription factors regulating parathyroid gland development such as GCMB (glial cells missing B) [34] or GCM2 (glial cells missing 2) [35], GATA3 [36, 37], or Sry-box 3 (SOX3) [38], is thought to be very rare. Autosomal dominant hypoparathyroidism associated with deafness and renal anomalies has been linked to mutations in the GATA3 gene on chromosome 10p14-10-pter [36, 37]. Hypoparathyroidism has been very rarely associated with X-linked recessive mutations on Xq26-27, leading to disruption of SOX3 transcription [38]. The syndrome of autosomal recessive hypoparathyroidism, growth and mental retardation, and dysmorphism due to mutations in the TBCE gene on chromosome 1q42-q43 is another very rare cause of hypoparathyroidism [39]. Hypoparathyroidism with metabolic disturbances and congenital anomalies has been associated with rare maternal mitochondrial gene defects [40, 41].
PTH Resistance
Pseudohypoparathyroidism (PHP) is a complex disorder with several recognized subtypes, characterized biochemically by hypocalcemia, hyperphosphatemia, and hyperparathyroidism due to tissue unresponsiveness to PTH [42] (Table 21.3). Most often hypocalcemia is not present at birth, and typically develops during childhood. The previous gold standard for diagnosis of PHP was the Ellsworth–Howard test, in which bovine PTH was infused to determine if urinary cyclic AMP increased normally. In most forms of PHP, urinary cyclic AMP does not increase as expected. This test is rarely performed today due to lack of bovine PTH, and the diagnosis is often based on the constellation of biochemical findings, family history when present, and genetic analysis.
Table 21.3
Characteristics of pseudohypoparathyroidism subtypes
Type | Gsα activity | AHO | PTH resistance | Urinary cAMP response | Multiple hormone resistance | Molecular defect |
---|---|---|---|---|---|---|
1a | Reduced | Yes | Yes | Reduced | Yes | Heterozygous mutations in GNAS |
Pseudo-PHP | Reduced | Yes | No | Normal | No | Heterozygous mutation in GNAS |
1b | Normal | No | Kidney | Reduced | No | Imprinting defect in GNAS |
1c | Normal | No | Yes | Reduced | Yes | Unknown |
2 | Normal | No | Kidney | Normal | No | Unknown |
PHP is further classified as types 1a, pseudo-PHP, 1b, 1c, or 2. In PHP type 1a, the most common subtype, loss-of-function mutations in the coding region of the maternally inherited GNAS gene encoding the Gsα subunit of G proteins, causes the disorder, with resultant 50 % loss of Gsα protein expression [43]. PHP type 1a patients have PTH resistance at the renal tubule, resulting in a blunted phosphaturic and cAMP response to PTH. This blunted response is due to lack of normal signaling by the PTH receptor due to reduced stimulatory G protein expression.
PHP type 1a is characterized by Albright’s hereditary osteodystrophy (AHO), which includes obesity, round facies, mild mental retardation, and a skeletal phenotype involving short stature, brachydactyly of hands and/or feet, and heterotopic ossifications in subcutaneous tissues. This disorder is frequently associated with multiple hormone resistance involving thyroid-stimulating hormone (TSH), luteinizing hormone (LH), follicle-stimulating hormone (FSH), calcitonin, and/or growth hormone-releasing hormone (GHRH).
Patients with PHP type 1a have renal tubule PTH resistance because they inherit a mutated maternally imprinted GNAS allele. GNAS alleles undergo differential imprinting in mothers and fathers, with tissue-specific expression of alleles in offspring. Only the maternal allele is expressed in the kidney and in the relevant endocrine organs associated with hormone resistance in this form of the disorder, but the rest of the body expresses both maternal and paternal alleles. An affected allele in other body tissues leads to haploinsufficiency and AHO expression, but PTH resistance occurs only in the tissues expressing the maternal allele.
Patients with paternally inherited GNAS mutations who have AHO without renal or endocrine gland resistance are designated as having pseudo-PHP. Patients with pseudo-PHP have a normal urinary cAMP response to PTH, unlike PHP type 1a patients. Patients with both PHP type 1a or pseudo-PHP may occur in the same kindred. In some cases both forms are found in the same generation, but more often the two forms are found in different generations.
PHP type 1b patients lack typical features of AHO, but may have mild brachydactyly [44]. Levels of Gsα in accessible tissues are normal, but patients have renal tubular PTH resistance without resistance to other hormones, although some may have mildly increased TSH levels. Skeletal manifestations are similar to those seen in patients with hyperparathyroidism, with bone loss or changes of osteitis fibrosa cystica. Most cases are due to switching of the maternal GNAS allele to a paternal pattern of methylation, caused by microdeletions in the STX16 gene located 220 kB centromeric from GNAS exon 1A, or deletions removing the differentially methylated region involving exon NESP55 or exons 3 and 4 of the antisense transcript [45]. In both situations, inheritance of a mutation from a female, or spontaneous mutation of a maternally derived allele, removes the maternal GNAS epigenotype, leading to transcriptional silencing of the Gsα promoter in imprinted tissues, with little or no expression of either GNAS allele in these tissues.
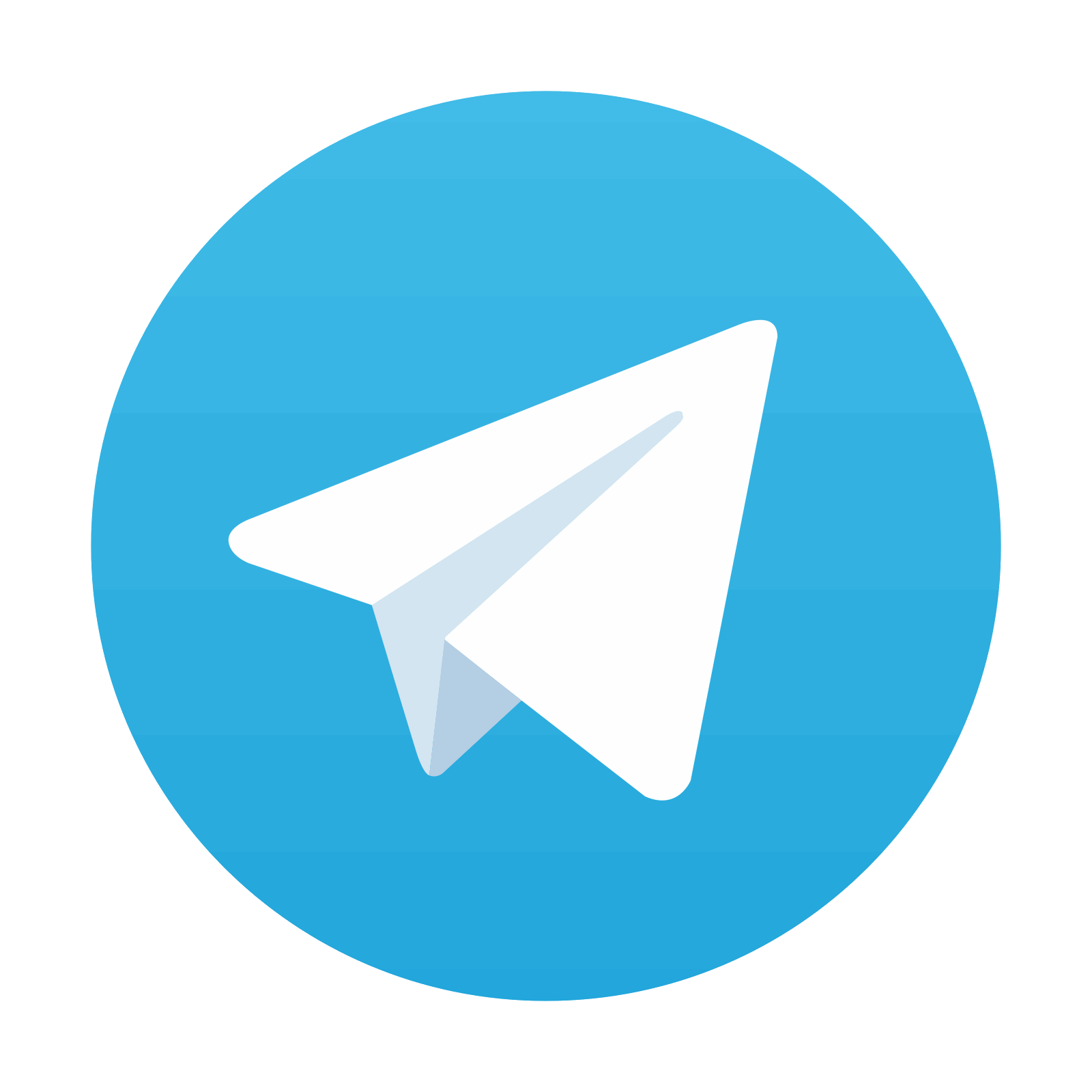
Stay updated, free articles. Join our Telegram channel

Full access? Get Clinical Tree
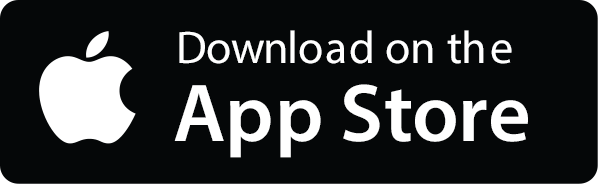
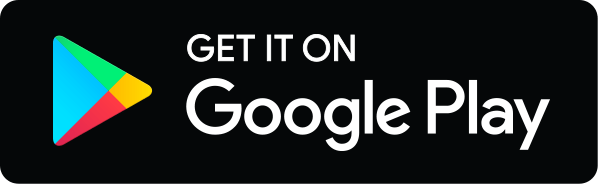