© Springer-Verlag London 2015
Hannah Cohen and Patrick O’Brien (eds.)Disorders of Thrombosis and Hemostasis in Pregnancy10.1007/978-3-319-15120-5_11. Hemostatic Changes in Normal Pregnancy
(1)
Centre for Haematology, Faculty of Medicine, Hammersmith Campus, Imperial College, London, W12 0NN, UK
Abstract
Hemostasis represents a balance between pro- and anti-coagulant processes, with variations in this balance determining the net outcome. Significant physiological changes during pregnancy result in a hypercoagulable and hypofibrinolytic state that serves to protect the mother from bleeding complications at the time of placental separation. This chapter describes the effects of pregnancy on parameters of primary hemostasis, coagulation factors, anticoagulant pathways and the fibrinolytic system.
Keywords
HemostasisPhysiologicalPregnancyHypercoagulabilityHypofibrinolysis1.1 Introduction
Under normal conditions, blood flows within the vascular system, transporting oxygen, nutrients, and hormonal information around the body and removing metabolic waste. The confinement of circulating blood to the vascular bed and maintenance of blood fluidity are dependent upon a complex hemostatic system that involves interaction between the vasculature, platelets, coagulation factors, and the fibrinolytic system. Such interaction enables the stimulation of coagulation following injury, limits the extent of the response to the area of injury, and initiates the eventual breakdown of the clot as part of the process of healing. Thus, hemostasis may be viewed as a delicate balance between the pro- and anticoagulant processes, with variations in this balance determining the net outcome.
As well as a significant expansion in plasma volume, normal pregnancy is accompanied by major changes in the maternal hemostatic system, most likely mediated by hormonal influences, the net effect of which is to create a state of hypercoagulability and hypofibrinolysis. Together with the stemming of placental blood flow by myometrial contraction, these changes protect the mother from bleeding complications at the time of delivery. However, potentiation of the coagulation system also confers an increased risk of venous thromboembolism (VTE) and disseminated intravascular coagulation (DIC), leading causes of morbidity and mortality in pregnancy and the postpartum period.
In order to understand the physiological effects of pregnancy on the hemostatic system, an appreciation of hemostasis in the nonpregnant individual is required. Thus, while this chapter will focus on the hemostatic changes during pregnancy, each will be discussed in the context of normal hemostatic processes. It should be borne in mind that although these will be covered individually, they should not be considered to act in isolation. Furthermore, caution needs to be exercised when interpreting data obtained from studies in pregnancy. Data reported from these studies are often conflicting, and the study design and methods by which data are analyzed and reported require careful consideration. For example, it is generally preferable to obtain serial measurements from women throughout pregnancy rather than a set of cross-sectional data from different groups. This will allow the detection of subgroups and effects of starting (pre- or early pregnancy) values although it may be possible to derive reference ranges from cross-sectional studies providing the sample size is sufficiently large. Nonetheless, the reader should be aware that individual cases may deviate from the pooled data.
1.2 Primary Hemostasis
1.2.1 Platelets
Platelets are produced in the bone marrow by megakaryocytes and have a circulating life span of approximately 10 days. Following vascular injury, platelets adhere to the subendothelium, either directly to collagen via glycoprotein (GP) IaIIa and GPVI or indirectly via von Willebrand factor (VWF) and GPIb. Adhesion initiates platelet activation, with release of granular contents, notably ADP, and the synthesis of thromboxane A2. These factors have a positive feedback effect and activate more platelets, which are captured by VWF also released from platelet granules. Platelet activation is completed by a platelet shape change and a conformational change in the GPIIbIIIa receptor resulting in platelet aggregates held together by VWF and fibrinogen. These effects are normally counterbalanced by the active flow of blood and the endothelial production of prostacyclin, nitric oxide, and ADPase, which suppress platelet activation and prevent inappropriate platelet aggregation.
During pregnancy, there is a physiological decrease in the maternal platelet count of approximately 10 % [1–4]. While the resultant platelet count at term is usually maintained within the normal range, the prevalence of gestational thrombocytopenia, as defined by a platelet count of less than 150 × 109 L−1, has been shown to range between 5 and 12 % in population-based studies [4–6]. Subsequently, the platelet count has been shown to rise on days 2–5 postpartum [7]. The degree of thrombocytopenia is mild in most cases of gestational thrombocytopenia, with platelet counts of greater than 115 × 109 L−1 reported in more than 80 % of cases, but a level down to 80 × 109 L−1 is regarded as normal [4]. Factors such as hemodilution and accelerated platelet clearance that may contribute to the fall in platelet count have not been well defined. Earlier studies do not consistently show reduced platelet survival in pregnancy [8, 9], and means of directly assessing platelet lifespan are limited in pregnant women. It is possible that the increase in platelet volume reported in conjunction with falling platelet counts in the third trimester indicates a state of increased platelet destruction [10, 11]. Moreover, while enhanced platelet activation has been demonstrated in pregnancies complicated by hypertension or preeclampsia, it is less clear whether this occurs in normal pregnancy. Spontaneous platelet aggregation, increased platelet reactivity to arachidonic acid, and, more recently, increased platelet activation and adhesion have been variously demonstrated in normotensive pregnant women [12–14]. Increased numbers of circulating platelet aggregates and a rise in levels of β-thromboglobulin, platelet factor 4, and thromboxane B2 (the stable product of thromboxane A2) have also been reported, all suggestive of enhanced platelet reactivity [15–17]. Thus, there is some evidence of increased platelet activation in uncomplicated pregnancies, but it is not clear to what extent this contributes to the fall in platelet count. Some increased activation would be consistent with other evidence for coagulation activation that is exaggerated in preeclampsia. Platelet aggregation has been shown to return to normal by 6 weeks postpartum [12, 13].
1.2.2 Von Willebrand Factor
Von Willebrand factor is a multimeric glycoprotein synthesized by endothelial cells and megakaryocytes that mediates the adhesion of platelet to sites of injury and promotes platelet-platelet aggregation. In addition to its adhesive properties, VWF is a specific carrier for factor VIII (FVIII), and the VWF/FVIII complex usually circulates with a molar ratio of FVIII to VWF monomer of 1:50 (although this is defined as a ratio of 1 in units/dL). Levels of maternal VWF rise progressively with advancing gestational age, increasing early in the first trimester of pregnancy and continuing to increase thereafter [1, 18, 19]. By the time of delivery, levels of VWF may have reached double or more those of the nonpregnant state, falling rapidly following delivery and returning to baseline non-pregnant values within a few weeks [2, 18–21]. Factor VIII levels also increase progressively throughout pregnancy [2, 18, 19, 22], rising early in the first trimester and returning to near-normal values by around 8 weeks postpartum [22]. The increase in FVIII has been shown in some studies to parallel the rise in VWF levels, thus maintaining the VWF:Ag/FVIII ratio close to normal [18, 19, 23]. Other studies have demonstrated an increase in this ratio, in particular during the third trimester of pregnancy [2, 20, 21, 24, 25], reflecting a relatively greater rise in VWF levels with advancing gestation. This discrepancy may reflect variation in the degree of endothelial perturbation, as it is recognized that the rise in VWF levels is greater in conditions associated with endothelial cell damage such as preeclampsia [24, 25]. The functional activity of VWF is determined by its multimeric size, which is regulated by the protease ADAMTS13. Studies of ADAMTS13 during pregnancy have shown a mild progressive decrease in its activity from early in the second trimester until the first or second postpartum day [26, 27], and discrepancies between ADAMTS13 antigen and activity have been reported in pregnancy in older mothers [28]. A reduction in ADAMTS13 activity would be consistent with a reported disproportionate increase in large VWF multimers in the third trimester [24] and an increase in the specific functional activity of VWF, although this finding is not consistently reported [19, 21].
1.2.3 Hematocrit
Hematocrit also plays a role in primary hemostasis by influencing blood viscosity and platelet adhesion. However, as with the decreased platelet count, any decrease in platelet adhesion that may result from a reduction in hematocrit appears to be outweighed by the increase in VWF levels. This is discussed further when we consider global measures of primary hemostatic function.
1.3 Coagulation System
Blood coagulation pathways center on the generation of thrombin [29], which cleaves fibrinogen to generate fibrin, the structural scaffold that stabilizes platelet aggregates at sites of vascular injury [30].
1.3.1 Initiation of Coagulation
The extrinsic or tissue factor (TF)-dependent pathway of coagulation represents the major route by which thrombin generation is initiated in response to vessel damage. TF is an integral membrane protein and is the only procoagulant factor that does not require proteolytic activation. TF is primarily located at extravascular sites that are not usually exposed to the blood under normal physiological conditions [31]. As a result, the blood only encounters TF-presenting cells thus activating the extrinsic pathway, at sites of vascular injury. This dogma has been challenged by the identification of microparticle-associated TF and an alternatively transcribed, soluble TF molecule in blood, both of which have been implicated in the development of thrombosis [32, 33].
As well as being the principal initiator of coagulation, TF is essential for other cellular processes including implantation, embryogenesis, and angiogenesis [34]. The decidua and placenta are both rich sources of TF, with high levels of expression on syncytiotrophoblasts, while tissue factor pathway inhibitor (TFPI) is expressed in human umbilical vein endothelial cells [35]. Progesterone has been shown to upregulate TF gene expression by decidualized endometrial stromal cells, principally mediated by the promoter-specific transcription factor Sp1 [36]. This may be relevant in reducing postimplantation hemorrhage, although few studies have addressed the role of TF and TFPI in the pathogenesis of gestational complications [37].
1.3.2 Overview of Coagulation and Its Regulation
Following the exposure of TF-presenting cells to the blood, TF comes into contact with factor VII (FVII), a fraction (~1 %) of which circulates in its active form, FVIIa. TF binds both FVII and FVIIa with high affinity. The trace amount of TF-FVIIa that forms is enough to activate factor X (FX) both directly (extrinsic pathway) and indirectly via activation of factor IX (FIX) [38, 39]. However, FXa production by this route is rapidly terminated by the action of TFPI which binds FXa and VIIa forming an inactive TF-VIIa-Xa-TFPI complex. The limited quantities of FXa that are generated via this route facilitate the inefficient conversion of trace quantities of prothrombin to thrombin [40, 41]. The low concentrations of thrombin that arise mediate the feedback activation of the cofactors FVIII and factor V (FV). Binding of FIXa to FVIIIa forming the intrinsic tenase activates further FX thus bypassing the reliance upon TF-FVIIa as a source of FXa generation. The subsequent assembly of FXa and FVa on activated platelets (prothrombinase) leads to a rapid burst in thrombin generation at the site of vessel damage. Binding of the intrinsic tenase and prothrombinase complexes to the phospholipid surfaces expressed by platelets is mediated by calcium ions bound to the terminal gamma-carboxy residues on FXa and FIXa. Gamma-carboxylation of glutamic acid residues on FIX and FX as well as prothrombin and FVII requires vitamin K.
Fibrin is produced by proteolytic cleavage of fibrinogen by thrombin with the release of fibrinopeptides A and B and precipitation of insoluble fibrin monomer followed by polymerization and cross-linkage by factor XIII (FXIII). Thrombin has many functions that influence both coagulation and the vascular system; in addition to cleaving fibrinogen to produce fibrin and activating FVIII and FV as described above, other procoagulant functions of thrombin include the activation of platelets, factor XI (FXI), FXIII, and thrombin-activatable fibrinolysis inhibitor (TAFI).
1.3.3 Effect of Pregnancy on Coagulation Factors
As already discussed, normal pregnancy is accompanied by a rise in the plasma concentration of FVIII, which is likely to be secondary, at least in part, to increased VWF levels. Pregnancy also results in substantial progressive increases in the plasma concentrations of FVII [2, 42, 43] and fibrinogen [2, 3, 22, 42]. The increase in FVII levels appears to be greatest in the second trimester of pregnancy, with rises of around 50 % observed by the early third trimester [2, 42]. FVII levels fall sharply after delivery [2]. By term, levels of fibrinogen may reach double their prepregnancy values, and fall more slowly following delivery, returning to basal levels within 5 weeks post-partum [2, 3, 42]. A modest gradual increase in the plasma concentration of FX is apparent until around 30 weeks’ gestation, following which there are reports of both a persistent elevation and a mild fall in levels through the remainder of pregnancy [2, 19, 20, 42]. Levels of prothrombin and FV remain unchanged throughout pregnancy [2, 19, 42, 44]. Data available regarding the effect of pregnancy on plasma concentrations of FIX and FXI are variable; several early studies reported a steady increase in FIX levels with advancing gestation [45–47], but no significant effect on FIX levels was shown in a subsequent cross-sectional study [19]. A longitudinal study has confirmed the earlier findings, demonstrating FIX levels at delivery to be above the non-pregnant upper reference value in approximately 50 % of women, with a further rise during the early puerperal period [42]. The finding of a prolonged activated partial thromboplastin time (APTT) at term not infrequently results in the incidental finding of reduced FXI levels, which subsequently normalize in the months following delivery. Indeed, a progressive decrease in FXI levels throughout pregnancy has been found in several studies, reaching a nadir at term [20, 48, 49]. There are also reports of unchanged FXI levels in pregnancy, although these studies report grouped data and may not detect individual trends [19, 42].
It has been shown that, following an initial early increase, FXIII levels have been shown to gradually fall throughout pregnancy, reaching levels around half nonpregnant values by term [50]. The significance of this is not clear because the minimum hemostatic level of FXIII is not established although some studies suggest that this might be low enough to increase the risk of bleeding.
There is considerable interindividual variation in the extent to which plasma levels of coagulation factors change with advancing gestational age. It is likely that some of the reduction is due to dilution caused by the progressive increase in plasma volume until around the 32nd week of gestation. However, as the plasma volume expands by approximately 50 %, increased synthesis is necessary to maintain clotting factor concentrations at equivalent nonpregnant values. This is most likely to be mediated by hormonal factors. Altered transcriptional activity in the presence of estrogenic factors has been demonstrated for some coagulation factors, with the identification of estrogen response elements in gene promoter regions [51]. Estrogen levels increase as pregnancy progresses; however, as with other placentally derived hormones, levels vary significantly between individuals [52].
1.4 Anticoagulant Pathways
The hemostatic response is confined to the site of injury by the action of inhibitory pathways preventing spontaneous activation of coagulation and generalized thrombosis. As already discussed, TFPI is the principal inhibitor of TF-mediated coagulation [53] and acts by binding and inhibiting FXa [54]. The resultant TFPI-FXa complex acts in a negative feedback loop through the binding and inactivation of TF-FVIIa [55] and in this way switches off the initiating procoagulant stimulus. This ensures that a small procoagulant stimulus does not elicit uncontrolled generation of thrombin. In addition to TFPI, the normal hemostatic response is regulated by anti-thrombin and the protein C–protein S pathway. Together, these pathways modulate the generation and activity of thrombin and are critical for appropriate and controlled hemostatic plug formation.
1.4.1 Antithrombin
The half-life of thrombin in plasma is around 14 s. This is mainly due to the inhibitory action of antithrombin (previously designated antithrombin III), a plasma-borne serine protease inhibitor synthesized in the liver. Although antithrombin inactivates several protease substrates of the coagulation system, its main physiological targets are thrombin and FXa, and it is through the inhibition of these serine proteases that antithrombin exerts its anticoagulant function. These interactions are enhanced by the action of heparan sulfate proteoglycans, as well as exogenously administered heparins, on the surface of endothelial cells. The activity of antithrombin is reduced in liver disease and hypercoagulable states including DIC. However, there is little variation in the plasma concentration of antithrombin both during healthy pregnancy and following delivery [2, 3, 19, 20, 42, 56]. In contrast, modest effects on the thrombin inhibitors α2-macroglobulin and heparin cofactor II (HCII) are found; the plasma concentration of α2-macroglobulin is reduced and HCII activity increased in normal pregnancy [2, 57, 58], while HCII activity is reduced in pregnancies complicated by hypertension and preeclampsia [59].
1.4.2 Protein C and Protein S
Thrombin generation is also downregulated by the anticoagulant activities of protein C and its cofactor protein S, both vitamin K-dependent glycoproteins that are synthesized mainly in the liver. Protein C is activated by thrombin bound to thrombomodulin (TM), an integral membrane protein expressed on the surface of endothelial cells. This activation process is enhanced by a second endothelial cell surface protein, the endothelial cell protein C receptor (EPCR). Both the TM and EPCR receptors are also expressed by placental trophoblasts. Aided by protein S, activated protein C (APC) catalyzes the inactivation of FVIIIa and FVa [60], thereby limiting further generation of thrombin. As the intact endothelium (i.e., adjacent to the site of vascular injury) normally expresses TM [61], thrombin diffusing away from the site of injury can bind to TM and its activity “switched” to impart an anticoagulant function [62]. Thus, the action of thrombin can be modulated depending on its location relative to the thrombus. In addition to its cofactor-mediated anticoagulant function, protein S has been shown to exhibit anticoagulant properties in the absence of APC, including cofactor activity for TFPI [63, 64].
Protein S circulates in plasma in two forms. Approximately 60 % is bound to complement 4b-binding protein (C4BP), a regulatory protein of the classical pathway of the complement system [65, 66], while the remaining 40 % is free. While it was previously thought that only protein S in its free form was active, APC cofactor activity for FVa and FVIIIa inactivation has now also been claimed for C4BP-bound protein S, albeit to a lesser degree than the activity of free protein S [67, 68]. Similarly, while TFPI cofactor activity has been suggested for bound protein S [63], recent data suggest that this is less potent than that of free protein S [69]. The implications of these recent findings for pregnancy-associated hemostasis remain to be clarified.
A decrease in the plasma concentration of protein S has been reported during both pregnancy and the puerperium, frequently to levels comparable to protein S-deficient heterozygotes. Two early cross-sectional studies showed plasma levels of both total and free protein S to be reduced in pregnancy, irrespective of whether the free protein S fraction was measured by an immunological or a functional method [70, 71]. The latter study showed more pronounced reductions in free protein S compared to total protein S that may be explained by the parallel increase in C4BP concentration; no change in C4BP was demonstrated in the former. Furthermore, concordant reductions in total and free protein S levels with no increase in C4BP have been found in women taking the combined oral contraceptive pill [71]. Together, these findings suggest that the reduction in protein S activity in pregnancy may not be wholly attributable to alterations in the free/bound protein S equilibrium. Similarly, progressive reductions throughout pregnancy in the plasma concentrations of both free and total protein S have subsequently been reported, with greater relative decreases in the free fraction [3, 19, 42]. The majority of studies report that the decrease in protein S levels begins early in the first trimester, with most reduction having occurred by the end of the second trimester [3, 19, 22, 42, 71]. A cross-sectional study demonstrated a significant decrease in the levels of free protein S from the 10th week of gestation, and total protein S levels from the 20th week, with no significant increase in C4BP at term compared to nonpregnant samples [72]. Total protein S has been shown to return to prepregnancy levels by the end of the first week postpartum [71], while levels of free protein S remain below the normal range in 15 % of women at 8 weeks postpartum [3, 22]; this should be considered when evaluating the results of thrombophilic tests.
It is possible that some of the discrepancies in findings are attributable to assay variability; however, the underlying mechanism for the decrease in protein S levels in pregnancy remains unclear. A dilution effect could account for some of the findings but would not explain the reduction in protein S levels associated with the combined oral contraceptive pill. Similarly, the reduction in free protein S levels may not be wholly attributable to increased C4BP levels, as has been the widely held view to date. Estrogen response elements have also been identified in the protein S gene promoter, which may alter the transcription activity of protein S, and this seems to offer the most likely explanation.
Unlike protein S, neither the concentration nor functional activity of protein C has been shown to be significantly affected by gestation [18, 19, 73]. Some minor variations in protein C activity have been demonstrated with a peak in levels in the second trimester; however, all values remained within the normal range [3, 18, 71]. Elevated protein C levels and activity have been reported in the early puerperal period with mean levels equivalent to nonpregnant upper reference values [19, 42, 74].
In addition to their anticoagulant activities, protein C and protein S appear to have other physiological effects including cellular protective, anti-inflammatory, and anti-apoptotic properties. Immunohistochemical analysis has recently demonstrated the deposition of protein S in damaged villi at the fetal-maternal interface, suggesting its involvement in the protection or restoration of damaged placental trophoblasts [72]. It is possible that protein S consumption at the fetal-maternal interface could contribute toward the observed reduction in its levels in pregnancy. Low levels of anti-protein S and anti-protein C antibodies have recently been shown in a cross-sectional study of healthy pregnant women, the significance of which is not known [75]. Plasma levels of anti-protein C antibodies were shown to decrease with advancing gestational age and were no longer detectable by the third trimester [75].
1.5 Fibrinolytic System
The principal fibrinolytic enzyme is plasmin, which circulates in its inactive zymogen form, plasminogen. The activation of plasmin is mediated by two types of plasminogen activator: tissue type and urokinase type. Tissue plasminogen activator (tPA) is released into the blood by endothelium in its active form but does not activate plasminogen until they are brought together when they bind to fibrin. Plasmin is inhibited both directly by plasmin inhibitor (α2-antiplasmin) and α2-macroglobulin and indirectly by plasminogen activator inhibitor-1 (PAI-1) produced by endothelial cells and platelets. Plasmin activation is reduced by TAFI, which removes the terminal lysine residues from fibrin, to which tPA and plasminogen bind.
It has been a long-held view that maternal plasma fibrinolytic activity decreases in pregnancy, which has been attributed, at least in part, to the production of a plasminogen inhibitor by the placenta known as plasminogen activator inhibitor-2 (PAI-2) [2, 78, 79]. The decreased fibrinolytic activity reported by many of these studies is based on fibrin plate and clot lysis analyses of euglobuin fractions (which contain factors important in fibrinolysis: plasminogen, plasminogen activators (primarily tPA) and fibrinogen), although it is unclear how accurately these reflect the blood fibrinolytic capacity in pregnancy. In addition to PAI-2, the placenta and decidua are sources of PAI-1, levels of which have been shown to rise progressively throughout pregnancy, reaching at least fivefold basal values by term [1, 3, 22, 79]. The decidual expression of PAI-1 appears to be regulated by progesterone by similar mechanisms to those described for TF [80]. Circulating tPA antigen levels have been shown to remain constant or rise during pregnancy [76, 79, 81–83], and the increase in PAI-1 and PAI-2 leads to a decrease in the release of endothelial tPA [84] and its measurable activity [22, 79]. A rise in levels of urokinase-type plasminogen activator (uPA) antigen, which also appears to derive from the placenta, has also been demonstrated [83, 85]. Fibrinolytic activity may be further depressed in pregnancy by increased thrombin generation leading to an increase in TAFI activity [86–89]. A slight initial rise in α2-macroglobulin levels followed by a steady fall throughout pregnancy and the postpartum period has been reported [2]. Levels of plasminogen and plasmin inhibitor appear to remain largely unchanged throughout pregnancy [83, 90]. A brisk fall in PAI-1 and rise in tPA activity immediately following delivery result in a rapid increase in fibrinolytic activity and a return to nonpregnant values within 3–5 days [2, 79, 83]. However, high levels of PAI-2 antigen persist for a further few days [83].
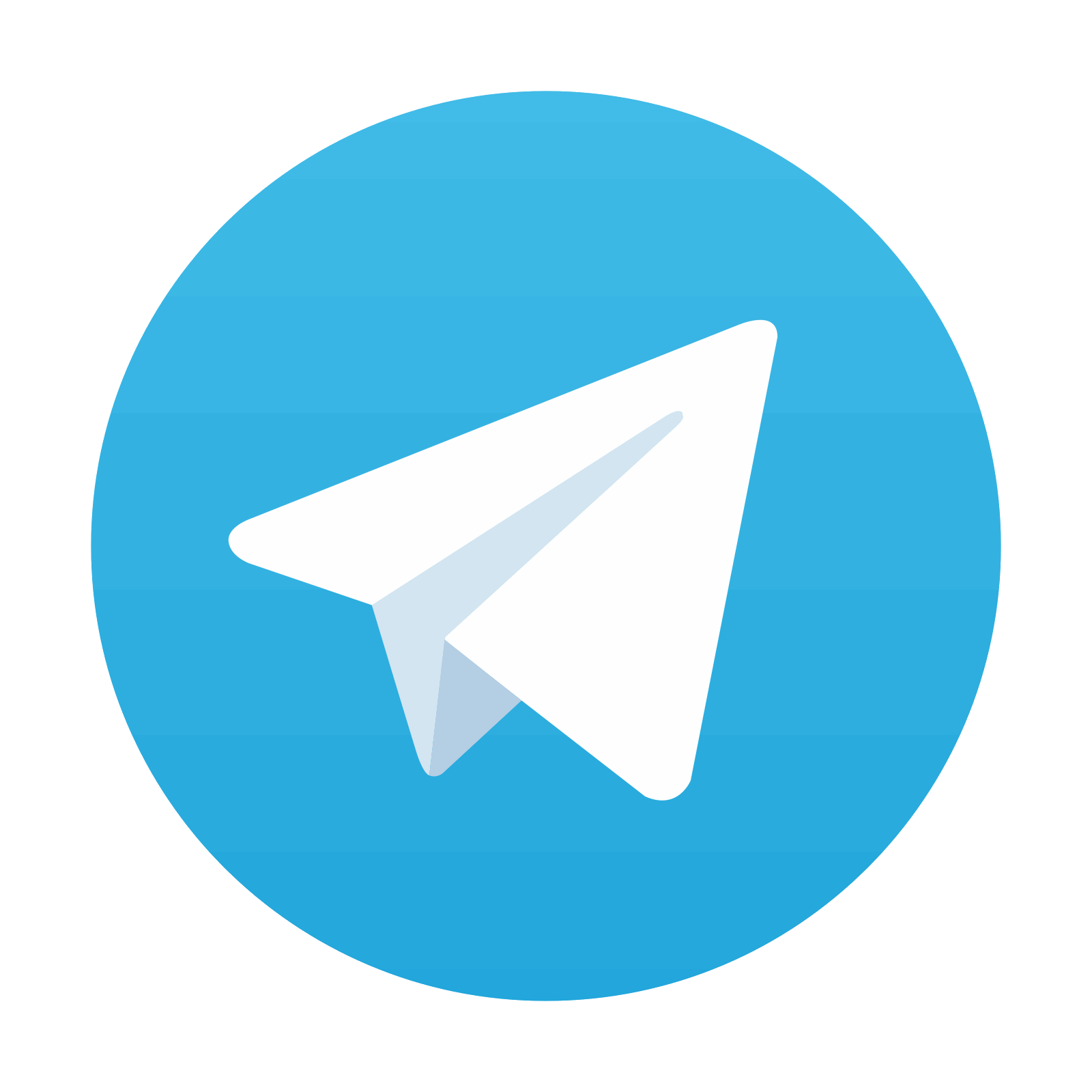
Stay updated, free articles. Join our Telegram channel

Full access? Get Clinical Tree
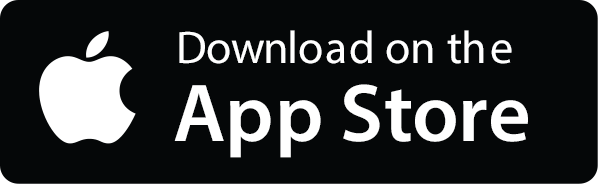
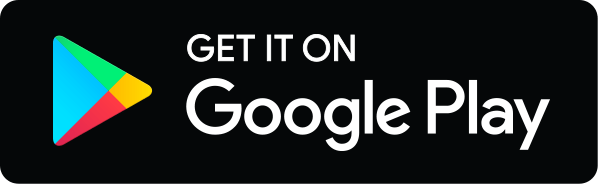