Fig. 2.1
Coagulation mechanism and targets for action of different anticoagulants. UFH unfractionated heparin, AT antithrombin, LMWH low-molecular-weight heparin
The amount of activated factor X is further enhanced by the simultaneous formation of factor IXa which, in the presence of factor VIIIa and calcium, also leads to activation of factor X. The tissue factor/VIIa complex is inhibited by circulating tissue factor pathway inhibitor (TFPI), but fibrin generation continues due to the back-activation of factor IX, V and VIII by thrombin.
Antithrombin regulates coagulation by irreversibly binding to the active serine site of thrombin (factor IIa) and other activated clotting factors including Xa, IXa, XIa and XIIa. In the absence of heparin, the rate of inactivation is slow. Heparin-like glycosaminoglycans such as heparan sulphate are expressed on the surface of endothelial cells. Once heparan sulphate binds to a lysine residue on the antithrombin molecule, a conformational change occurs at the arginine reactive centre resulting in a marked increase in activity.
Thrombomodulin is expressed on endothelial cells and platelets. Thrombomodulin binds thrombin, and the thrombin-thrombomodulin complex is a potent activator of protein C. Protein C and protein S are both vitamin-K dependent plasma proteins. In its activated form protein C, together with protein S as a cofactor, then inactivates factors Va and VIIIa. In addition, protein C possesses profibrinolytic activity that results from neutralisation of plasminogen activator inhibitor-1 (PAI-1) activity.
Older anticoagulants such as unfractionated heparin (UFH), low-molecular-weight heparin (LMWH) and warfarin, and other vitamin K antagonists (VKA), act on a number of targets whereas the newer agents (e.g. direct thrombin inhibitors, anti-Xa inhibitors) have been designed to have highly specific targets within the coagulation mechanism (Fig. 2.1).
2.3 Parenteral Anticoagulants
2.3.1 Unfractionated Heparin (UFH)
Heparin is a negatively charged, sulphated glycosaminoglycan, which exerts its anticoagulant effect primarily by activating antithrombin. Only one third of the heparin chains contain the critical pentasaccharide sequence, which allows for high-affinity binding to antithrombin. At therapeutic concentrations the remaining two thirds have minimal anticoagulant activity [1]. The binding of heparin to antithrombin induces the conformational change in the reactive site of antithrombin, which leads to rapid inhibition of the procoagulant activity of thrombin and factors IXa, Xa, XIa and XIIa. The average length of the heparin chains in unfractionated heparin (UFH) preparations is 45 saccharide units, with the molecular weight ranging from 3,000 to 30,000 Da (mean 15,000 Da). Only heparin chains of 18 saccharide units or longer are of sufficient length to simultaneously bind to antithrombin and thrombin, which is required to catalyse the inhibition of thrombin. The inhibition of thrombin is particularly important as it prevents fibrin formation but also thrombin-induced activation of platelets and factors V, VIII and XI. In contrast, the inhibition of factor Xa can be achieved with shorter heparin chains containing the pentasaccharide sequence [1].
2.3.1.1 Pharmacokinetics of UFH
Heparin is poorly absorbed from the gastrointestinal tract and must be administered parenterally, either by continuous intravenous infusion or subcutaneous injection [2]. The bioavailability of UFH after subcutaneous administration is poor and variable (20–30 %) [3, 4] and its use has largely been superseded in clinical practice by subcutaneous low-molecular-weight heparins (LMWHs).
UFH has complex pharmacokinetics, resulting in a nonlinear anticoagulant response at therapeutic doses, with both the intensity and duration of anticoagulant effect rising disproportionately with increasing doses [5, 6]. There is also wide inter-patient variation in the response to UFH and the adequate dose must be determined on each occasion.
Once UFH reaches the bloodstream, it binds to many plasma proteins as well as to endothelial cells and macrophages [7, 8] which contributes to the inter-individual variability of its anticoagulant effect. It is cleared through a combination of a rapid saturable mechanism and a slower, non-saturable, renal mechanism [5, 6, 9].
2.3.1.2 Safety and Efficacy of UFH in Pregnancy
Several studies have found that UFH does not cross the placenta [10, 11] and therefore lacks the potential to cause fetal bleeding or teratogenicity [12]. Ginsberg et al. [13] examined 186 reports describing fetal/infant outcomes in 1,325 pregnancies associated with anticoagulant therapy. They found that pregnancy outcomes in UFH-treated women were similar to those in the normal population. This was after exclusion of pregnancies in which women had comorbid conditions independently associated with adverse outcomes. A retrospective cohort study of 100 pregnancies in 77 women who received UFH for the prevention or treatment of venous thromboembolism (VTE) (98 pregnancies) or prosthetic heart valves (2 pregnancies) also noted that the rates of prematurity, miscarriage, stillbirth, neonatal death and congenital abnormalities were similar to those in the normal population. There were two bleeding episodes and no symptomatic thrombotic episodes. The authors concluded that maternal UFH therapy is safe for the fetus [13].
Therapeutic anticoagulation with UFH requires a continuous intravenous infusion with regular monitoring of the activated thromboplastin time (APTT) ratio and resultant dose changes in accordance with local nomograms. Twice daily subcutaneous injections have also been advocated; however, Hull et al. [3] showed in a randomised double-blind trial that intermittent subcutaneous heparin was inferior to continuous intravenous heparin infusion in preventing recurrence of VTE in non-pregnant patients with an acute proximal DVT. The need for labour intensive monitoring, coupled with its variable pharmacokinetics, has largely relegated the use of intravenous UFH to specific clinical situations, where a faster ‘on-off’ anticoagulant effect is required in preference to the longer acting LMWH. Intravenous UFH is advisable in the context of massive pulmonary thromboembolism with cardiovascular compromise where thrombolysis or thrombectomy might be required [14].
Non-hemorrhagic side-effects of UFH such as heparin-induced thrombocytopenia (HIT) and heparin-induced osteoporosis will be discussed in more detail later in this chapter.
2.3.2 Low-Molecular-Weight Heparins
LMWHs are fragments of UFH produced by either chemical or enzymatic depolymerisation. The resulting heparin chains are approximately 15 saccharide units in length and have a mean molecular weight of 4,000–5,000 Da. Like UFH, LMWHs exert their anticoagulant effect through the catalysis of antithrombin activity, but the chain length of most of the fragments is too short to achieve inhibition of thrombin (IIa) [16]. On the other hand, factor Xa inhibition does not depend on chain length and is unaffected. This explains why UFH has an equivalent inhibitory effect on Factor Xa and thrombin whilst LMWHs possess proportionately greater anti-Xa than anti-IIa activity (2:1 to 4:1) [6, 16]. There is, however, no evidence that this difference impacts on clinical outcomes [6].
2.3.2.1 Pharmacokinetics of LMWHs
LMWHs have a more favourable pharmacokinetic profile compared to UFH. Their lower affinity for plasma proteins and cells leads to a more predictable dose-response relationship [17], longer plasma half-life [18, 19] and lower incidence of side-effects. Table 2.1 summarises key pharmacokinetic parameters for commonly used LMWHs: dalteparin (Fragmin; Pfizer) [20]; enoxaparin (Clexane; Sanofi-Aventis) [22] and tinzaparin (Innohep; Leo-Laboratories) [16, 21, 23–25].
Table 2.1
Comparison of key pharmacokinetic parameters for commonly used LMWHs
LMWH | Mean molecular weight (Da) | Bioavailability (post SC) | Tmax plasma anti-Xa (post SC) | Plasma T1/2 (post SC) | Anti-Xa/anti-IIa ratio |
---|---|---|---|---|---|
Dalteparin [20] | 4,000–6,000 | 87 % | 3–4 h [21] | 3.5–4 h | 2.7 [16] |
Enoxaparin [22] | 4,500 | ~100 % [23] | 1–4 h | 4–5 h | 3.8 [16] |
Tinzaparin [24] | 6,500 [25] | 90 % | 4–6 h | 90 min | 1.9 [16] |
After a subcutaneous injection, the bioavailability of UFH is only around 20–30 % whilst the bioavailability of LMWHs depends on the product used but is generally around 90 % or more (Table 2.1) [26]. This means that therapeutic treatment levels can be achieved with once or twice daily subcutaneous dosing, rather than a continuous intravenous infusion as is required for anticoagulation with UFH.
With LMWH, peak anti-Xa levels are achieved 3–5 h after subcutaneous administration. LMWHs are eliminated by a non-saturable renal mechanism [27], with an elimination half-life of approximately 3–6 h after a subcutaneous injection that is independent of dose [26].
In the non-pregnant state, the dose for therapeutic anticoagulation in patients with a normal body mass index (BMI) is usually based on the actual body weight and administered every 24 h without the need for any specific monitoring. For patients with extreme BMIs at either end of the spectrum, anti-Xa monitoring should be considered; in very obese patients a twice daily dosing regimen is sometimes used.
LMWHs can accumulate in renal failure, leading to concerns about an increased bleeding risk especially when therapeutic doses are administered. If LMWH is used in patients with an estimated creatinine clearance of less than 30 mL/min (less than 20 mL/min with tinzaparin), an empirical dose reduction is recommended (limited data available) and concurrent monitoring of anti-Xa activity should be considered [6].
2.3.2.2 Dosing and Monitoring of LMWHs During Pregnancy
Physiological changes during pregnancy lead to a marked increase in the glomerular filtration rate (GFR). Studies of healthy pregnant women found a 40–65 % rise in the GFR by the end of the first trimester [28], which is maintained for most of the pregnancy [29], then decreases during the last 4–6 weeks before term [29, 30]. In addition, the volume of distribution is increased in pregnancy due to a 35–45 % increase in plasma volume together with a variable degree of weight gain. These changes, along with the production of placental heparinase, might all affect the pharmacokinetics of heparin in pregnancy.
Both Casele et al. [31] and Blomback et al. [32] investigated pregnant women on a fixed prophylactic dose of LMWH (enoxaparin 40 mg once daily and dalteparin 5,000 units once daily, respectively) and found that the clearance of LMWH was increased during pregnancy.
In a study by Lebaudy et al. [33], the pharmacokinetics of prophylactic dose enoxaparin were examined in 75 pregnant and 38 non-pregnant women. In accordance with the physiological changes in pregnancy, the clearance of the drug was higher throughout pregnancy compared with clearance in non-pregnant women. The volume of distribution changed according to the increase in body weight, with an additional 41 % increase in the last trimester of pregnancy, which occurred independent of changes in body weight.
Notwithstanding these findings, prophylactic dose LMWH is generally prescribed once daily. However, a twice daily weight-based dosing regimen would seem preferable when therapeutic anticoagulation is required, and this is in line with UK RCOG recommendations [14]. Advice on dosing for various clinical indications is detailed in individual chapters.
Controversy also exists around the question of whether monitoring with anti-Xa levels and dose adjustments are required in pregnancy, particularly for therapeutic anticoagulation. The RCOG guidelines on the management of VTE in pregnancy state that “routine measurement of peak anti-Xa activity for patients on LMWH for treatment of acute VTE in pregnancy is not recommended except in women at extremes of body weight (less than 50 kg or more than 90 kg) or with other complicating factors, such as renal impairment or recurrent VTE” [14]. The American College of Chest Physicians (ACCP) 2012 guidelines state that monitoring of anti-Xa levels may be advisable when treatment doses of LMWH are given during pregnancy [6].
The study by Rodie et al. [34] is often quoted in support of the notion that anti-Xa monitoring is not required during pregnancy. This case series comprised 33 pregnant women receiving therapeutic dose enoxaparin 1 mg/kg subcutaneously (sc) twice daily based on their early pregnancy weight for treatment of VTE. Peak anti-Xa levels taken 3 h after administration of enoxaparin remained within the therapeutic range, defined in this study as 0.44–1.0 IU/mL, throughout pregnancy. Three women required a dose reduction because peak anti-Xa levels were above the therapeutic range (1.2, 1.2 and 1.1 IU/mL). No woman had a further thromboembolic event. Of note, trough anti-Xa levels were not measured in this study.
In contrast, Jacobsen et al. [35] reported on 20 pregnant women treated with therapeutic dose dalteparin. Thirteen women were commenced on a conventional dose of 100 IU/kg sc twice daily and nine women required a dose escalation to reach the target peak anti-Xa activity of 0.5–1.0 IU/mL. Stable target anti-Xa levels were achieved after dose adjustments to a median of 113 IU/kg bd (range 105–125 IU/kg bd). Six women initially started with higher doses of dalteparin, median 112 IU/kg bd (range 105–125 IU/kg bd) and target anti-Xa levels were achieved in all six women. Only one woman required a dose reduction but her starting dose was significantly higher at 133 IU/kg bd. No recurrence of VTE was reported. Based on their findings the authors suggested that pregnant women should receive a 10–20 % higher dose of dalteparin than non-pregnant patients from the outset, which should then be adjusted on a regular basis according to weight gain.
Similar observations were made by Barbour et al. [36] who reported on 13 pregnant women who received therapeutic dose dalteparin at a regular dose of 100 IU/kg sc twice a day. With this dose, therapeutic peak levels of anticoagulation (anti-Xa level 0.5–1.0 IU/mL) were achieved in only 54 % of women at the start of treatment and by the third trimester this had dropped to 12 %. Despite twice daily dosing very few patients achieved trough levels within the therapeutic range. Even in patients with high therapeutic peak anti-Xa levels, trough levels were in the therapeutic range only 15 % of the time.
More recently, Friedrich and Hameed [37] reported on 15 pregnant women who were treated with subcutaneous enoxaparin 1 mg/kg ± 20 % twice a day. All peak anti-Xa levels (3–4 h after injection) were within the therapeutic range as reported by Rodie et al. [34]. However, 20 % of the 8-h and 73 % of the trough levels were reported to be subtherapeutic.
It remains unclear whether these short periods of what is considered to be subtherapeutic anti-Xa levels are significant but there is some concern that anticoagulation failure might occur as a result. Nevertheless, no further venous thromboembolic events were reported in any of the above studies but the numbers were too small to detect such differences with statistical validity.
It should be noted that, whilst recognising its limitations, the anti-Xa assay is the most informative assay available for monitoring of LMWH therapy [38, 39]. Inter-assay variability of results is high [38] and data to support the association between the clinical efficacy of LMWH and peak anti-Xa levels are limited [40]. In clinical trials evaluating the efficacy of LMWHs in non-pregnant patients, dosing was weight-based and not guided by target anti-Xa levels, but dosing data for special populations are lacking.
The International Society of Thromb Haemost (ISTH) Control of Anticoagulation Subcommittee of the ISTH Standardisation Committee stated in 2002: “Use of anti-Xa assays may provide some clue to the pharmacokinetics of LMWH when used to treat thrombosis in those in whom standard or weight adjusted dosing is likely to be unreliable, especially subjects with severe renal failure, the obese, the pregnant, neonates and infants” [41].
2.3.2.3 Safety and Efficacy of LMWH in Pregnancy
There is a paucity of data regarding the efficacy of LMWHs in pregnancy and recommendations are based largely on extrapolation of data from non-pregnant patients as well as case reports and case series from pregnant patients. Several large clinical trials in non-pregnant patients showed that LMWH is at least as effective and safe as UFH for the treatment of patients with acute DVT and PE [42, 43], and for the prevention of DVT in patients who undergo surgery [44]. There are also studies in non-pregnant patients showing that long-term LMWH (and UFH) is as effective and safe as warfarin for the prevention of recurrent VTE [45–47].
In 2005, Greer and Nelson-Piercy published a systematic review on the safety and efficacy of LMWH for thromboprophylaxis and treatment of VTE in pregnancy [48]. Sixty-four studies reporting on 2, 777 pregnancies were included in the analysis. The risk of VTE recurrence using treatment dose LMWH was reported as 1.15 %, which compares favourably with recurrence rates of 5–8 % in non-pregnant patients treated with LMWH or UFH followed by warfarin. LMWH was also shown to have a reduced risk of bleeding when compared to UFH. In 55 of 2,777 (1.98 %) pregnancies, significant bleeding occurred of which 12 (0.43 %) were antenatal bleeding complications, 17 (0.61 %) were wound hematomas and 26 (0.94 %) were postpartum hemorrhage (PPH; blood loss >500 ml). In most cases of PPH a primary obstetric cause was identified. This compares favourably with the estimated risk of PPH after vaginal delivery of 4 % in the general population and a rate of massive PPH (blood loss >1,500 mL) of 0.7 % from one prospective study of women not receiving LMWH [49]. There was a reported rate of 1.8 % of skin reactions, no reports of heparin-induced thrombocytopenia and a low rate of heparin-induced osteoporosis (0.04 %). The authors concluded that LMWH is both safe and effective to prevent or treat VTE in pregnancy.
There is no evidence in the literature to suggest that placental transfer of LMWH occurs during pregnancy. No anti-Xa activity was detected when fetal blood samples or cord blood of women receiving LMWH during the second and third trimesters of pregnancy were tested [11, 50, 51]. LMWH therefore lacks the potential to cause fetal bleeding or teratogenicity. It should however be noted that several multidose formulations of LMWH contain benzyl alcohol as a preservative. These should be avoided in pregnancy because benzyl alcohol can cross the placenta and has been associated with fatal “gasping” syndrome in premature neonates [52].
2.3.2.4 Use in Breastfeeding
There are limited data available regarding the excretion of LMWH into human breast milk. Richter et al. [53] reported on 15 women receiving 2,500 IU of dalteparin daily as thromboprophylaxis after Cesarean section. Low anti-Xa activity was detected in the breast milk of 11 women. The oral absorption of LMWH is, however, extremely low as it is inactivated in the gastrointestinal tract so the risk to a breastfeeding infant from these drugs is negligible [15].
2.3.3 Fondaparinux
Fondaparinux [54] is a synthetic analogue of the heparin pentasaccharide sequence required for antithrombin binding and was the first of a new class of selective factor Xa inhibitors. It has a molecular weight of 1,728 Da and high affinity for antithrombin. Its specific anti-Xa activity is seven times higher than that of LMWH [55]. After binding to antithrombin, a conformational change occurs which significantly increases the ability of antithrombin to covalently bind to factor Xa. Fondaparinux is then released and available to activate further antithrombin molecules. The saccharide chain of fondaparinux is too short to bridge the distance between antithrombin and thrombin, so it does not have any inhibitory effect on thrombin [55].
2.3.3.1 Pharmacokinetics of Fondaparinux
After subcutaneous dosing, fondaparinux is completely and rapidly absorbed (absolute bioavailability 100 %), reaching peak levels approximately 2 h after administration. There is negligible binding to plasma proteins or red cells. Fondaparinux is not metabolised and there is no formation of active metabolites. The elimination half-life (t1/2) is approximately 17–21 h. Approximately 64–77 % of the drug is renally excreted as unchanged compound. It is contraindicated in severe renal impairment (creatinine clearance less than 30 mL/min) and should be used with caution in women weighing less than 50 kg or with moderate renal impairment [54].
Fondaparinux is usually administered as a once daily subcutaneous injection, without the need for anticoagulant monitoring in most situations. For venous thromboprophylaxis a dose of 2.5 mg is recommended and the treatment dose is based on body weight [54].
2.3.3.2 Use in Pregnancy
In an ex vivo model with the use of dually perfused human cotyledon, no placental transfer of fondaparinux was observed [56]. Dempfle [57] reported on five pregnant women who were treated with fondaparinux at a dose of 2.5 mg once a day because they had severe cutaneous allergic reactions to LMWH. In four patients, the anti-Xa activity was elevated in the cord blood sample and the concentration of fondaparinux was approximately 1/10 of the level in the maternal plasma [57]. The concentration of fondaparinux detected in the cord blood was well below the concentration required for effective anticoagulation and no adverse effects were noted in the newborns.
Winger and Reed [58] reported in a retrospective study on the use of fondaparinux (2.5 mg sc once daily) in 29 pregnancies. Safety and efficacy outcomes were compared with the results from 98 pregnancies treated with enoxaparin (30 mg sc twice daily) over the same time period. The decision regarding which anticoagulant to use was based mainly on physician’s choice. The women had a history of miscarriage and/or infertility and the anticoagulant was commenced on day 6 of the conception cycle. The pregnancy success rate was similar in the two groups. Treatment was well tolerated and no severe bleeding complications or serious allergic reactions were observed [58].
Knol et al. [59] recently published a report on ten pregnant women who were switched from LMWH to fondaparinux due to hypersensitivity skin reactions to LMWH. The data were collected as part of a prospective cohort study and no increase in bleeding, thromboembolic complications or fetal abnormalities were noted in relation to the use of fondaparinux.
In view of the limited data, the ACCP recommends in its 2012 guidelines that the use of fondaparinux in pregnancy should be reserved for women with heparin-induced thrombocytopenia (HIT) or a history of HIT who cannot receive danaparoid [60]. To date no embryo/fetal harm has been reported with the use of fondaparinux in pregnancy and it should not be withheld if clinically indicated [15].
2.3.3.3 Use in Breastfeeding
2.3.3.4 Other Non-hemorrhagic Side-Effects
Other non-hemorrhagic side-effects are very uncommon and include skin reactions that can progress to necrosis, alopecia and hypersensitivity.
2.3.4 Heparin-Induced Thrombocytopenia
Heparin-induced thrombocytopenia (HIT) is an antibody-mediated adverse effect of heparin that is strongly associated with arterial as well as venous thrombosis and can lead to life-threatening complications if not diagnosed early. The pathophysiological basis of HIT is the development of IgG antibodies to a complex of platelet factor four (PF4) and heparin that leads to intravascular platelet activation [61]. PF4 forms tetramers, which allow for the interaction with heparin. The binding of heparin to PF4 leads to a conformational change and this is thought to stimulate antibody formation [62]. The ability to induce conformational change depends on the chain length and degree of sulphation of the glycosaminoglycan, which explains the difference in incidence of HIT observed with different heparins [63].
The frequency of HIT among patients exposed to heparin is highly variable and is influenced by the heparin preparation used, duration of heparin exposure and patient population [60]. For patients receiving UFH in therapeutic doses, the risk of HIT has been estimated at 1 % at most [64]. For obstetric patients receiving prophylactic dose UFH, the incidence of HIT is considered to be infrequent (0.1–1 %), whilst for those receiving only LMWH or Fondaparinux, the risk of HIT is considered rare (<0.1 %) [60].
The RCOG guidelines [14] recommend that platelet monitoring for HIT is not required for obstetric patients receiving LMWH unless they have also received UFH. The ACCP [60] and British Committee for Standards in Haematology (BCSH) guidelines [63] concur with this approach. If acute or subacute HIT is strongly suspected or confirmed then heparin (UFH/LMWH) should be discontinued and therapeutic anticoagulation with an alternative non-heparin anticoagulant is required. The data to guide the choice of an alternative anticoagulant are of poor quality. The ACCP guidelines suggest danaparoid over other non-heparin anticoagulants, with the use of fondaparinux only if danaparoid is not available [60]. The BCSH guidelines concur with this [63]. In patients with a past history of HIT who require thromboprophylaxis, the limited available evidence suggests that the longer the re-exposure to heparin, the higher the likelihood of re-emergence of HIT antibodies and thus the potential for acute HIT. Therefore, in pregnancy where generally prolonged thromboprophylactic anticoagulation is required, a non-heparin anticoagulant should be used. As 25 % of patients with HIT develop thrombosis before the platelet count falls, platelet count monitoring may not detect sensitisation.
2.3.5 Effect of Heparin on Bone Turnover
During pregnancy and lactation, significant changes occur in maternal calcium and bone metabolism. The daily transfer of calcium from mother to fetus in the first trimester is 2–3 mg/day whereas the rate at 35–36 weeks’ gestation is estimated at 250 mg/day [65, 66]. Maternal absorption of calcium from the intestine increases significantly to meet the calcium requirements of the fetus. Maternal bone loss may occur in the last months of pregnancy when the fetal skeleton is rapidly mineralising. During lactation there is additional mobilisation of calcium from maternal bone which can lead to a transient loss of approximately 3–7 % of bone mineral density (BMD). This is rapidly regained after weaning [67]. The rate and extent of recovery is influenced by the duration of lactation and postpartum amenorrhea and differs by skeletal site, but recovery is thought to be complete for most women [65, 68].
The long-term use of UFH has been associated with an increased risk of bone loss and osteoporotic fractures [69–71]. This is particularly relevant in pregnancy, as this is one of the few times when long-term prophylaxis or treatment with heparin might be required. Muir et al. [72] demonstrated in a rat model of heparin-induced osteoporosis, that UFH and LMWH both cause a dose-dependent decrease in bone density by reducing the number and activity of osteoblasts, but only UFH was found to increase the number and activity of osteoclasts leading to increased bone resorption.
In vitro the inhibitory effect of LMWH on bone nodule formation was found to be six to eightfold lower than that of UFH [73]. Longer chain length and higher net negative charge of heparin appear to determine the extent of suppression of bone nodule formation [72, 74]. The effect of heparin on osteoblast activity correlated with molecular weight and was pentasaccharide-independent.
Rajgopal et al. [75] reviewed the published clinical trials on this subject and found that pregnant women who received UFH at a dose of 12,000–40,000 IU/day for 25–35 weeks were reported to have a 5 % [76] to 7 % [77] reduction in BMD compared to untreated controls. The reported symptomatic vertebral fracture rate as determined by radiography was 2.2–3.6 % with UFH [78, 79].
Several studies have investigated the effect of prophylactic doses of LMWH on BMD in pregnant women. Nelson-Piercy et al. [80] reported on 61 women receiving enoxaparin 20–40 mg/day for 7.5 months and 30 % had a significant reduction in BMD. Two studies conducted by Pettila et al. [79] and Carlin et al. [81] were unable to demonstrate a difference in the reduction of BMD between the treatment group (dalteparin 2,500–7,500 IU/day) and the control group (no treatment with heparin). In the randomised controlled substudy of the multicentre randomised TIPPS (Thrombophilia in Pregnancy Prophylaxis Study) trial, thrombophilic pregnant women were randomised to either dalteparin 5,000 IU once daily until 20 weeks followed by 5,000 IU twice daily, or to the control group. Thirty-three patients received a mean of 212 days of dalteparin in the intervention group and 29 patients received a mean of 38 days of postpartum thromboprophylaxis with dalteparin in the control group. There was no difference in the mean BMD at 6 weeks postpartum between the intervention group and the control group, suggesting that the use of long-term prophylaxis with dalteparin in pregnancy is not associated with a significant decrease in the mean BMD [82]. Greer and Nelson-Piercy [48] reviewed 64 reports on the use of LMWH for thromboprophylaxis and treatment of venous thrombosis in a total of 2,777 pregnancies. Only one case of osteoporotic fracture was reported in a woman receiving high doses of dalteparin (15,000 IU/day) for a total of 36 weeks (overall risk 0.04 %).
These data combined suggest that the reduction in BMD is more pronounced with UFH than with LMWH and that the risk for developing an osteoporotic fracture after long-term use of prophylactic dose LMWH in pregnancy is low, although data on therapeutic dose LMWH are limited.
2.3.6 Danaparoid Sodium
Danaparoid [85] is a mixture of low molecular weight sulphated glycosaminoglycuronans derived from animal mucosa, comprising heparan sulphate (approx 84 %), dermatan sulphate (approx 12 %) and a minor amount of chondroitin sulphates A and C (approx 4 %) [85, 86]. It has a mean molecular weight of approximately 5,500 Da. Although often termed a low molecular weight ‘heparinoid’, it is free of heparin or heparin fragments and differs in chemical structure. Danaparoid has a high anti-Xa to anti-IIa activity ratio [86]. It exerts its anticoagulant effect primarily through inhibition of factor Xa in an antithrombin-dependent fashion [6], resulting in an effective inhibition of thrombin generation and thrombus formation [85].
2.3.6.1 Pharmacokinetics of Danaparoid
After subcutaneous administration, the absolute bioavailability of danaparoid approaches 100 % and the time to reach peak plasma anti-Xa activity levels is approximately 4–5 h [85]. Based on anti-Xa levels, its elimination half-life is approximately 25 h after subcutaneous or intravenous administration and is independent of the dose [85]. Steady-state levels of plasma anti-Xa activity are usually reached within 4–5 days of dosing.
Renal excretion is the main route of elimination, accounting for approximately 40–50 % of the total plasma clearance of anti-Xa activity following intravenous administration [87]. The manufacturer recommends that it should be used with caution in patients with moderately impaired renal and/or liver function with impaired hemostasis, and it should not be used in patients with severe renal impairment/hepatic function unless the patient has HIT and no alternative treatment is available [85].
Plasma anti-Xa activity is linearly related to the dose of danaparoid administered. Anti-Xa assays have been used to monitor danaparoid but whether these are useful is not clear. Expert opinion suggests that monitoring may be of value in patients with severe renal impairment and extremes of body weight (<55 and >90 kg) [63, 85, 88].
2.3.6.2 Efficacy and Safety in Pregnancy
In pregnancy the use of danaparoid is limited to women with current HIT, a past history of HIT or other forms of heparin intolerance. The in vitro cross-reactivity of danaparoid to HIT antibodies can be up to 50 % depending on the sensitivity of the assays used [89] but in vivo cross-reactivity is rare [90] and danaparoid remains one of the therapeutic options for the management of HIT within consensus guidelines [60, 63].
The evidence in the literature regarding the safety of danaparoid use in pregnancy is limited. Animal studies have not demonstrated any teratogenic effect or placental transfer [85, 91]. In a recent review of 91 pregnancies in 83 women treated with danaparoid, it was also concluded that none of the fetal adverse events that occurred were attributable to maternal danaparoid use [92]. Of note, there was an increase only in the premature birth rate. None of the six fetal cord blood samples or five breast milk samples obtained showed transfer of anti-Xa activity [92].
2.3.6.3 Use in Breastfeeding
Studies have not formally evaluated the use of danaparoid in lactation, but the molecule is large and should not be expected to pass into human milk. In addition, the drug will be inactivated in the human gastrointestinal tract so is unlikely to cause harm to a breastfeeding infant [15].
2.3.7 Lepirudin
Lepirudin is a direct, irreversible thrombin inhibitor with a molecular weight of approximately 7,000 Da, used in the treatment of HIT. There are only a small number of case reports describing a favourable outcome with its use in pregnant women [93–96]. However, data from animal studies suggests that placental transfer of lepirudin might occur [97, 98] and at present there is not enough evidence to evaluate the safety of its use in pregnancy. The ACCP guidelines therefore recommend its restriction to women with severe allergic reactions to heparin (including HIT) who cannot receive danaparoid [99]. It should be noted that the manufacturer ceased to supply Refludan® (Lepirudin) leading to the permanent discontinuation of Refludan® in the European Union from 1st April 2012. This decision was not related to any safety concerns.
2.3.8 Argatroban
Argatroban [100] is a synthetic inhibitor of thrombin and is derived from L-arginine. It exerts its anticoagulant effect by reversibly binding to the active site of thrombin. It is primarily indicated as an anticoagulant for the treatment of thrombosis in patients with HIT. Administration is by continuous infusion, and subsequent dose adjustments are based on APTT monitoring. The drug undergoes partial metabolism in the liver. The terminal elimination half-life is 39–51 min [100]. The published evidence of the use of argatroban in pregnancy is limited to a small number of case reports [101–103]. It is not known whether argatroban crosses the placenta, but one would expect some fetal exposure due to the low molecular weight (527), low metabolism and moderate serum binding of the drug [15]. The effect of argatroban on reproduction has been incompletely studied in animal experiments, as technical issues have limited systemic exposure [15, 100].
2.3.9 Reversal of Anticoagulant Effect
One advantage of UFH over LMWH is that its anticoagulant effect can be rapidly and completely reversed by the intravenous administration of protamine sulphate. However, severe hypotension and anaphylactoid reactions have been reported, particularly with large doses and rapid administration [6, 105]. When used at doses in excess of that required to neutralise anticoagulation, protamine sulphate may exert its own anticoagulant effect and cause bleeding complications [105].
Protamine sulphate only partially reverses the effects of LMWHs. It neutralises the anti-IIa activity (hence normalising the APTT and thrombin time) but only partly neutralises the anti-Xa activity [106]. In addition, the longer half-life of LMWH compared to UFH and the possibility of continued absorption from a subcutaneous depot, means that repeated doses of protamine may be required for up to 24 h after subcutaneous administration of LMWH [105].
There is no known pharmacological antidote to fondaparinux. In the event of severe bleeding, initiation of appropriate therapy such as surgical hemostasis, blood replacement, fresh plasma transfusion or plasmapheresis should be considered [54]. There are also no known pharmacological antidotes to danaparoid, argatroban or lepirudin. Management of bleeding should be through cessation of treatment and general hemostatic measures, with activation of the hospital’s major hemorrhage protocol if appropriate.
2.4 Antiplatelet Agents
2.4.1 Aspirin
Aspirin (acetylsalicylic acid) irreversibly inactivates cyclooxygenase by acetylation. Cyclo-oxygenase is required for the synthesis of prostaglandins and thromboxane and exists in two isoforms COX-1 and COX-2. COX-1 is present in most cells and involved in the physiological production of prostaglandins. COX-2 is induced by cytokines, mitogens and endotoxins in inflammatory cells and is responsible for the production of prostaglandins during inflammatory processes.
Cyclooxygenase converts arachidonic acid to prostaglandin H2, which is the precursor for the different prostanoids. Subsequent steps differ depending on cell type. Human platelets primarily produce thromboxane A2 leading to the induction of platelet aggregation and promotion of vasoconstriction [107].
On the other hand, vascular endothelial cells produce prostacyclin (PGI2) via the COX-1 and, to a greater extent, the COX-2 pathways [108], leading to the inhibition of platelet aggregation and induction of vasodilation [107]. In the gastric mucosa the activation of COX-1 leads to the production of prostacyclin, which exerts a cytoprotective effect.
Aspirin blocks the COX channel by acetylation of a serine residue that prevents access of the substrate to the catalytic site of the enzyme [109]. Inhibition of the COX-1-dependent platelet function can be achieved with low-dose aspirin whilst high-dose aspirin inhibits both COX-1 and COX-2. The platelet inhibitory effect of aspirin lasts for the lifespan of the platelet [110–112], which is approximately 8–10 days in humans.
2.4.1.1 Pharmacokinetics of Aspirin
Aspirin is absorbed by passive diffusion of unionised drug in the stomach and upper small intestine and has a bioavailability of 40–50 % [113]. The plasma half-life of aspirin is approximately 20 min as it is rapidly converted to salicylate once absorbed. Both aspirin and salicylate are bound to serum albumin and are widely distributed (including to the synovial cavity, central nervous system and saliva). Both have pharmacological activity although only aspirin has an anti-platelet effect. Measurable inhibition of platelet function occurs within 60 min of oral intake [113]. Salicylate is eliminated mainly by hepatic metabolism but is also partly excreted unchanged in the urine (the amount depends on plasma concentration and urinary pH).
2.4.1.2 Aspirin—Other Points for Consideration
An expert consensus document on reducing the gastrointestinal (GI) risks associated with the use of antiplatelet agents and non-steroidal anti-inflammatory drugs (NSAIDs) acknowledged that the use of low-dose aspirin is associated with a two- to fourfold increase in the risk of upper gastrointestinal side-effects which is not reduced by the use of buffered or enteric-coated preparations [114]. Anecdotal reports of reduced dyspepsia with these products, however, have probably contributed to their continued uptake in clinical practice [115].
Whilst ranitidine is considered safe for use in pregnancy and breastfeeding [15], the level of acid suppression provided by traditional doses does not prevent most NSAID-related gastric ulcers [114]. There are few data on the use of ranitidine in conjunction with aspirin. If therapeutic or prophylactic gastroprotection is required, a proton pump inhibitor is the preferred option.
Based on findings from a large Swedish cohort study [116], the administration of omeprazole during pregnancy appears to pose little risk to the fetus. In a multicentre prospective controlled study, exposure to omeprazole, lansoprazole or pantoprazole in the first trimester of pregnancy did not cause any increase in the rate of major congenital malformations compared to the control group [117]. Case reports of women receiving omeprazole or pantoprazole during lactation report detection of traces of the drug in the breast milk but no adverse effects were seen in the infants [118, 119].
2.4.1.3 Use in Pregnancy
The use of low-dose aspirin in pregnancy is based on the suspected role of prostanoids in normal pregnancy physiology. An imbalance in prostanoids, with a deficiency in vasodilatory prostacyclin (PGI2) and/or dominance of vasoconstrictory thromboxane A2, was reported in women with a history of recurrent early miscarriage [120] and in women with late pregnancy complications such as intrauterine growth restriction and preeclampsia [121]. Thromboxane A2 overproduction was also found in pregnant women with systemic lupus erythematosus and detectable antiphospholipid antibodies [122]. Low dose aspirin (defined as 40–150 mg) predominantly inhibits COX-1, leading to a reduction in the synthesis of thromboxane A2 without affecting the synthesis of prostacyclin (PGI2). It thereby restores the ratio of the two substances to a more normal value in women with a history of these obstetric complications.
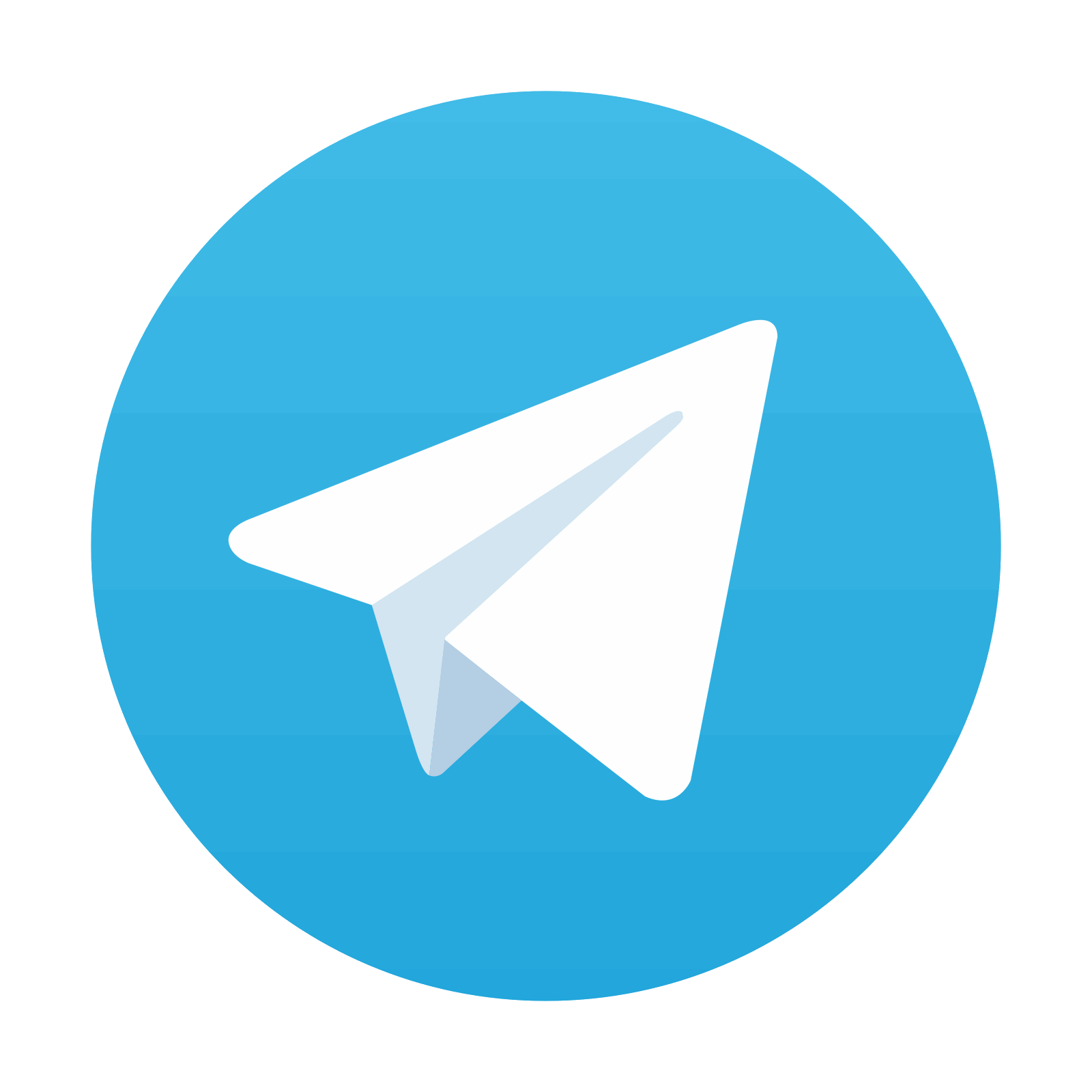
Stay updated, free articles. Join our Telegram channel

Full access? Get Clinical Tree
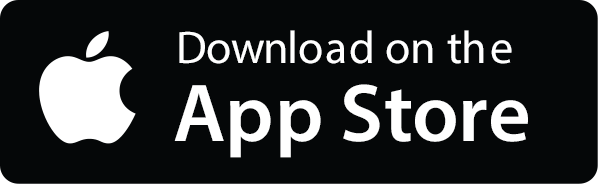
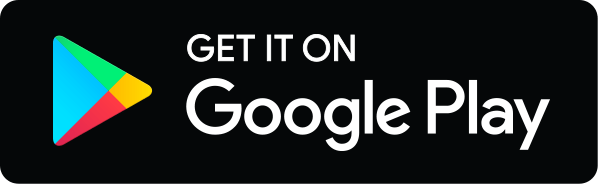