Pancreatic ductal adenocarcinoma remains a clinical challenge. Thus far, enlightenment on the downstream activities of Kras, the tumor’s unique metabolic needs, and how the stroma and immune system affect it have remained untranslated to the clinical practice. Given the numbers of diverse therapies in development and a growing knowledge about how to evaluate these systems preclinically and clinically, this is expected to change significantly and for the better over the next 5 years.
Key points
- •
Pancreatic ductal adenocarcinoma remains a clinical challenge.
- •
Thus far, enlightenment on the downstream activities of Kras, the tumor’s unique metabolic needs, and how the stroma and immune system affect it have remained untranslated to the clinical practice.
- •
Given the numbers of diverse therapies in development and a growing knowledge about how to evaluate these systems preclinically and clinically, this is expected to change significantly and for the better over the next 5 years.
Pancreatic ductal adenocarcinoma (PDA) is an aggressive malignancy that carries a poor prognosis with a 5-year survival on the order of 6% ; new and innovative treatments are needed. Several factors underlie its aggressive nature and resistance to treatment: the genetic framework, early metastasis, a dense stroma, propensity for growth in a nutrient-deplete environment, and immunomodulation have all made therapeutic progress a challenge. This article focuses on recent advances in understanding the tumor genetics and cell biology of pancreatic cancer. It reviews the established genetic hallmarks, examines more recently described mutations and altered pathways, and highlights key biological principles identified in PDA with a focus on those most likely to lead to future therapeutic targets.
Genetics
Pancreatic adenocarcinoma shows genetic homogeneity on one level with mutations in KRAS , found in anywhere from 90% to 95% of advanced pancreatic cancers, and additional frequent and well-characterized mutations in the key tumor suppressor pathways TP53/p19ARF , RB/CDKN2A/INK4A , and TGFBeta/SMAD4 . The biological significance of many of these mutations has been investigated in numerous contexts and model systems and the main impact of each on the disease is briefly highlighted later. Beyond this element of genetic homogeneity, PDA is broadly characterized by general genetic instability with widespread mutations and chromosomal translocations, including the recent discovery of many additional mutated loci and classes of genes whose full cancer-specific functions have yet to be fully explored. These genes include members of the SWI/SNF family, MLLs, and the DNA damage repair system, including ATM . The genetic hallmarks of PDA are described below and the broad implications of recent tumor genetic analyses are discussed later with regard to understanding of the natural history of the disease ( Box 1 ).
- •
KRAS is the most common mutational hallmark of PDA and can activate the RAF/MEK/ERK and PI3K pathways.
- •
Mouse models with K-RAS mutations typically require subsequent genetic events, such as loss of tumor suppressor genes INK4A, P53, or SMAD4, to develop tumors similar to human PDA.
- •
Finding that loss of SMAD4 has a greater propensity for metastatic spread is an example of how genetic sequencing may help tailor future therapy to the individual.
Genetics
Pancreatic adenocarcinoma shows genetic homogeneity on one level with mutations in KRAS , found in anywhere from 90% to 95% of advanced pancreatic cancers, and additional frequent and well-characterized mutations in the key tumor suppressor pathways TP53/p19ARF , RB/CDKN2A/INK4A , and TGFBeta/SMAD4 . The biological significance of many of these mutations has been investigated in numerous contexts and model systems and the main impact of each on the disease is briefly highlighted later. Beyond this element of genetic homogeneity, PDA is broadly characterized by general genetic instability with widespread mutations and chromosomal translocations, including the recent discovery of many additional mutated loci and classes of genes whose full cancer-specific functions have yet to be fully explored. These genes include members of the SWI/SNF family, MLLs, and the DNA damage repair system, including ATM . The genetic hallmarks of PDA are described below and the broad implications of recent tumor genetic analyses are discussed later with regard to understanding of the natural history of the disease ( Box 1 ).
- •
KRAS is the most common mutational hallmark of PDA and can activate the RAF/MEK/ERK and PI3K pathways.
- •
Mouse models with K-RAS mutations typically require subsequent genetic events, such as loss of tumor suppressor genes INK4A, P53, or SMAD4, to develop tumors similar to human PDA.
- •
Finding that loss of SMAD4 has a greater propensity for metastatic spread is an example of how genetic sequencing may help tailor future therapy to the individual.
KRAS
KRAS is a member of the RAS family of GTP-binding proteins that controls cellular proliferation and survival. Inactivation of RAS occurs via GTP hydrolysis with the aid of GTPase-activating proteins (GAPs). Activating KRAS point mutations at codon 12, the most common in PDA, occur near the nucleotide binding site, desensitizing RAS to GAPs and inhibiting GTP hydrolysis, resulting in a constitutively active RAS. This process leads to a unique genetic/biochemical/signaling paradigm compared with other oncogenes, because KRAS is not so much biochemically turned on via mutation, but rather cannot be turned off. Consequently, mutant RAS is a difficult therapeutic target because the loss of its GTPase enzymatic activity is not easily pharmacologically restored. This challenge has shifted efforts from targeting KRAS directly to focusing on downstream signaling pathways of KRAS .
KRAS mutation leads to constitutive activations of key mitogenic and survival signaling pathways, including RAF/MEK/ERK and phosphatidylinositol 3 kinase (PI3K). The relative importance of each of these has been evaluated in several in vitro and in vivo systems. In vitro studies revealed that RAS activates RAF and mitogen-activated protein (MAP) kinases, crucial for DNA synthesis, in the absence of other growth factors, and that its activities could also be abrogated with PI3K dominant negatives. Mouse models have provided further insight into the relative importance of PI3K and RAF/MAP/MEK pathways. PI3K-activated models without Kras mutation showed no pancreatic abnormalities, whereas Braf -mutated models developed PanIn (pancreatic intraepithelial neoplasms; these are discussed later), leading investigators to conclude that this may be the dominant branch of KRAS -mediated signaling in PDA. Furthermore, a Braf and Tp53 mutated model showed clear evidence of PDA with extensive metastasis. Treatment with MEK inhibitors suppressed phosphorylation of ERK but led to increased levels of phosphorylated AKT, a marker of PI3K activation, and cell lines treated with both MEK and AKT inhibition led to a synergistic antitumor effect. This antitumor effect, albeit modest, is also seen in dual MEK/PI3K treatment studies in mouse models. Thus, although RAF/MEK/ERK activation may be a dominant effector of KRAS activity, its pharmacologic inhibition led to activation of other compensatory downstream pathways driving survival and proliferation, explaining PDA’s resistance to single-agent MEK inhibition clinically.
Oncogene addiction has been described as a process in which effector proteins are involved in an intricate network of synergistic positive-feedback and negative-feedback loops to maintain tumor growth, homeostasis, and structural integrity; a process that is not simply a summation of the effects of acquired mutations. The addiction of subsets of PDAs to KRAS has been well described, shown by tumor regression with mutant Kras extinction. Dependence on mutant KRAS can be overcome, however, similar to the mechanisms of resistance described in many targeted therapies, and was recently described via amplification and overexpression of YAP-1. Yap-1 is a transcriptional coactivator involved in cell proliferation, epithelial-to-mesenchymal transition (EMT), and metastasis, previously linked to liver and esophageal tumors. Other routes to KRAS independence are also described, suggesting that there may be additional molecular routes by which KRAS-independent growth of PDA may be sustained.
INK4a/CDKN2A/P16
Loss of INK4a function brought about by mutation, deletion, or promoter hypermethylation occurs in about 80% to 95% of sporadic PDA. The INK4a gene encodes the tumor suppressor protein p16, which inhibits CDK4/6-mediated phosphorylation of RB, thereby blocking entry into the S phase (DNA synthesis) of the cell cycle (for a more in-depth review of INK4a/ARF , see Sharpless ). The 9q21 locus that contains INK4a also encodes the tumor suppressor gene ARF , whose protein product p19 stabilizes p53 by inhibiting MDM2-dependent proteolysis. Pancreatic cancers with mutations at this locus may sustain loss of both INK4a and ARF tumor suppression pathways, although some mutations have been found to affect loss of p16 alone. The importance of Ink4a in restraining PDA has been shown in murine models with engineered mutations in this locus designed to affect both p16Ink4a and p19Arf genes or p16Ink4a alone.
TP53 Tumor Suppressor Pathway
Mutations, predominantly missense, in the TP53 tumor suppressor gene occur in approximately 75% to 80% of human PDA cases. In normal unstressed cells, p53 is bound to MDM2, which targets p53 for proteasomal degradation. In response to cell stress and/or oncogene activation, p53 protein is stabilized and targets activation of genes involved in cell cycle arrest, apoptosis, DNA damage repair, and cellular metabolism. The role of TP53 loss in cancer is well established in that its mutation has been described in most human cancers and germline mutation of TP53 leads to the early development of sarcomas and carcinomas, also known as the Li-Fraumeni syndrome (for in-depth review of p53, see “The p53 Family,” subject collection in CSH Perspectives in Biology , 2010). Evidence from PDA precursor lesions (PanINs) suggests that loss or mutation of TP53 is a late event possibly caused by selective pressure after the collective accumulation of genetic aberrations, reactive oxygen species (ROS), and telomere erosion. The loss of p19ARF coexists with TP53 mutations in only about 40% of tumors, whereas the loss of TP53 or inactivation of this pathway is a more common feature of PDA, leading many to believe that these tumor suppressors have some overlapping function as well as independent capabilities.
DPC4/SMAD4/Transforming Growth Factor Beta
The SMAD4 ( DPC4 ) gene is located within chromosome 18q21 and is a key transcriptional regulator of the transforming growth factor beta (TGF-β) signaling cascade, a pathway that mediates proliferation, cell migration, apoptosis, and EMT. Loss of SMAD4 function has been reported in ∼50% to 60% of tumors and is associated with poor outcome in surgical patients. In a rapid-autopsy program examining 76 cases of PDA, SMAD4 loss was reported in only 22% of locally advanced cases with no metastatic disease at autopsy, but in 78% of those with widespread metastatic disease. These clinical findings, along with associated poor outcome in patients with SMAD4 loss, imply that intact SMAD4 function may help constrain metastatic spread, and investigators suggest that such patients with functional SMAD4 may benefit from intensive locoregional therapies.
Somatic mutation of Smad4 , as well as the type 2 TGF-β receptor in the murine pancreatic epithelium, neither disrupted pancreatic development nor induced malignancy, but hastened PDA development when coupled with Kras mutation. Although SMAD4 is mutated in about 50%, almost all tumors express high levels of both receptor and TGF-β ligands. In both human and murine model systems, activation of this pathway has been found to underlie cellular migration, metastasis, and EMT, raising the question of TGF-β inhibition as a therapy. Studies have been mixed depending on models used and underlying tumor genetics.
Advanced Genetic Sequencing Reveals Novel Mutations
The application of whole-genome sequencing to PDA has revealed additional new mutations in genes and pathways not previously recognized as being important in its pathogenesis as well as insights into its genetic evolution. Exon sequencing of 24 PDAs uncovered 1562 somatic mutations in 1327 genes and investigators organized these into a collection of 12 core signaling pathways ( Table 1 ). Although most of the 1327 genes identified were mutated in a small minority of cases, each core pathway was mutated in 67% to 100% of the 24 tumor specimens, suggesting that, although the mutational spectrum may be broad and heterogeneous, the physiologic result is distilled down to effects on conserved pathways. Separately, whole-exon sequencing of 99 patients confirmed these described mutations and identified novel mutations in PDA, including the axon guidance pathway genes SLIT and ROBO, previously known to be important in embryogenesis and central nervous system development ( Table 2 ). SLIT2/ROBO2 inactivating mutations were present in 5% of the cohort with copy number losses in ROBO1 and SLIT2 in another 15% of the population. The biological impact of SLIT/ROBO mutations in PDA is unexplored but, in other contexts, affects both MET and WNT signaling. In addition, novel mutations were discovered in EPC1 (3%) and ARID2 (3%), which affect chromatin modification, and in ATM (5%), with likely more of the cohort affected because of copy number variation losses in a small percentage of each gene. ATM , which encodes for a serine/threonine kinase DNA damage repair protein, has already been linked to familial cases of PDA along with BRCA2 and PALB2 ; this finding supports a role in sporadic PDA as well.
Core Pathway | Key Mutated Genes |
---|---|
KRAS signaling | KRAS, MAP2K4, RASGRP3 |
Regulation of G1/S phase | CDKN2A, FBXW7, CHD1, APC2 |
TGF-β signaling | SMAD4, SMAD3, TGFBR2, BMPR2 |
DNA damage control | TP53, ERCC4, ERCC6, RANBP2 |
Hedgehog signaling | TBX5, SOX3, LPR2, GL1, BOC, CREBBP |
WNT/Notch signaling | MYC, GATA6, WNT9A, TCF4, MAP2, TSC2 |
Apoptosis | CASP10, VCP, CAD, HIP1 |
C-Jun N-terminal kinase signaling | MAP4K3, TNF, ATF2, NFATC3 |
Regulation of invasion | ADAM11, DPP6, MEP1A, PCSK6, APG4A, PRSS23 |
Homophilic cell adhesion | CDH1, CDH10, PCDH15, PCDH17, FAT |
Small GTPase-dependent signaling (non-KRAS) | AGHGEF7, CDC42BPA, DEPDC2, PLCB3 |
Integrin signaling | ITGA4, ITGA9, LAM1, FN1, ILK |
Jones et al, 2008 | Biankin et al, 2012 |
---|---|
MLL3 (transcriptional activator) | ARID2 (chromatin modification) |
CDH10, PCDH15, PCDH18 (cadherin homologs) | ATM (DNA damage repair) |
CTNNA2 (alpha-catenin) | ZIM2 (transcriptional regulator) |
DPP6 (dipeptidyl-peptidase) | NALCN (Na-channel activity) |
BAI3 (angiogenesis inhibitor) | MAGEA6 (protein binding) |
GPR133 (G protein–coupled receptor) | MAP2K4 (toll-like receptor signaling pathway) |
GUCY1A2 (guanylate cyclase) | SLC16A4 (monocarboxylate transporter) |
PRKCG (protein kinase) | SLIT2, ROBO1, ROBO2 (axon guidance pathway genes) |
Q9H5F0 (unknown function) | SEMA3A, SEMA3E (semaphorins, axon guidance) |
Beyond the identification of genes harboring mutations that may be causal to the disease, these studies have offered more global insights into the genetic landscape and trajectory of the disease. In order to understand the relationship of a primary tumor to its attendant metastatic sites, somatic genetic rearrangements in 13 primary PDA tumors were compared with metastases. Although most of the 206 rearrangements were found in the primary tumor and all metastatic sites, supporting a conserved origin, clonal evolution was also identified. Furthermore, some genetic aberrations found in metastatic sites were organ specific, including mutations in MYC and CCNE1 that were found exclusively in lung metastases, suggesting that certain subclones may evolve in an organ-specific manner.
Genetic Pathogenesis
PDA may arise from at least 3 types of precursor lesions: PanIN, intraductal papillary mucinous neoplasm (IPMN), and mucinous cystic neoplasm (MCN). The most prevalent precursor is PanIN, a microscopic lesion graded I to III, encompassing a spectrum of increasing dysplasia and architectural disruption. High-grade PanIN lesions are commonly found in association with invasive PDA. Furthermore, advanced PanINs harbor many of the same genetic mutations as PDA, linking these entities molecularly as well as pathologically. IPMNs are less common precursors and PDA derived from IPMNs are thought by some clinicians to represent a different disease process, especially given its better prognosis and 5-year survival rate of 42% in those surgically resectable patients. Sixty-six percent of IPMNs harbor mutations in GNAS . Furthermore, investigation of 95 surgical PDA specimens without IPMN yielded no mutations in GNAS . These findings were corroborated through sequencing of 48 IPMNs that identified GNAS mutations in 79% of patients; only 50% harbored KRAS mutations and fewer showed loss of p16 (36%) and SMAD4 (24%). The genetics of MCNs are not clearly delineated, but a presentation in young women, mainly in the body and tail of the pancreas, suggests potential differing biology. Although there are key shared genetic traits between PanINs, PDA, and the lesser common precursors IPMNs and MCNs, these recent studies show how different genetic events may explain the divergent phenotypes of the malignancies arising from each type of precursor ( Box 2 ).
- •
More than 1500 mutations have been identified to occur in PDA; focusing on the core signaling cascades these affect may show greater promise than focusing on individual mutations.
- •
Next-generation sequencing efforts have identified many novel genetic aberrations in PDA, such as those in axon guidance pathways and chromatin modification, which require further investigation.
- •
PanIns are precursor lesions that have tight genetic linkage with PDA; IPMNs are less common PDA precursor lesions found to have frequent mutation of GNAS and slightly better outcomes, suggesting a potential divergent phenotype.
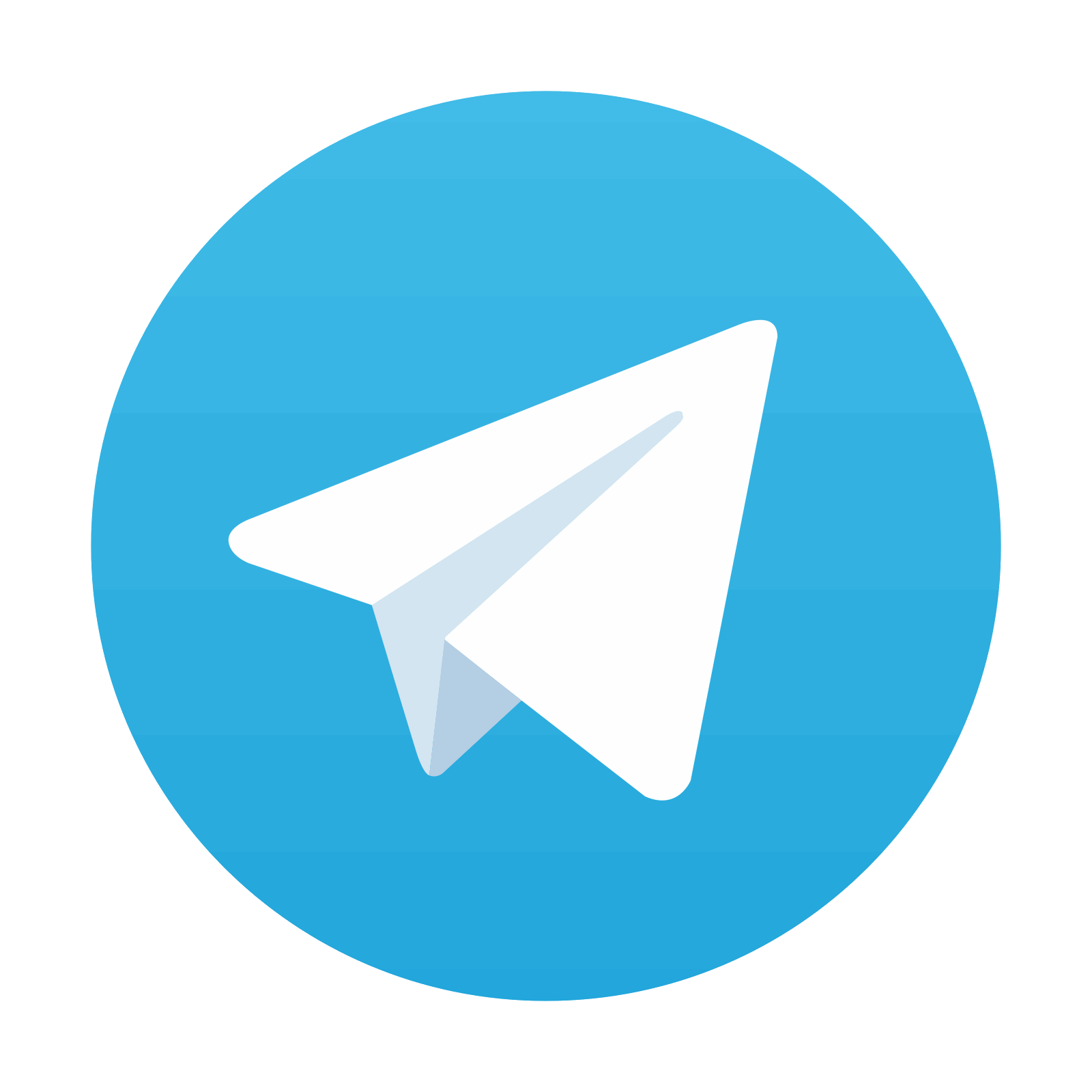
Stay updated, free articles. Join our Telegram channel

Full access? Get Clinical Tree
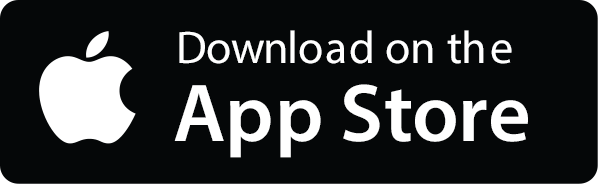
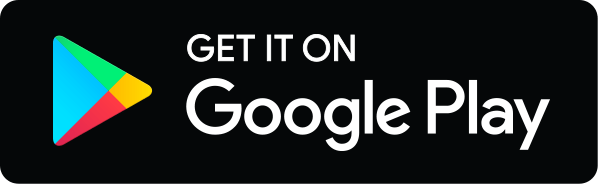
