14 Functional Mapping
Basic Principles
Direct cortical stimulation has been employed in neurosurgery since 1930, first by Foerster,1 and then later by Penfield.2–4 In recent years, the technique of intraoperative cortical stimulation has been adopted for the identification and preservation of language function and motor pathways. Stimulation depolarizes a very focal area of cortex, which, in turn, evokes certain responses. Although the mechanism of stimulation effects on language are poorly understood, the principle is based on the depolarization of local neurons and also of passing pathways, inducing local excitation or inhibition, as well as possible diffusion to more distant areas by way of orthodromic or antidromic propagation.5 Studies employing optical imaging of bipolar cortical stimulation in monkey and human cortex have shown precise local changes, within 2 to 3 mm, after the activation of cortical tissue.6,7 With the advent of the bipolar probe, avoidance of local diffusion and more precise mapping have been enabled with an accuracy estimated to be approximately 5 mm.6
Rationale for Functional Mapping
Hemispheric gliomas are often located within or adjacent to functional areas (e.g., rolandic cortex, supplementary motor areas, corona radiata, internal capsule, and uncinate fasciculus). Because gliomas have a tendency to invade underlying white matter tracts, it is important to identify both cortical sites and their descending pathways for the motor and somatosensory systems. Although extensive resection of a tumor involving the nondominant temporal lobe may be achieved without functional consequences other than a quadrantanopia, surgical resections in the dominant temporal lobe are more challenging due to the variable localization of language. Thus, although traditional neurosurgical teaching restricts temporal lobe resections to within 4 cm of the temporal tip and limits the removal of the superior temporal gyrus, dominant temporal lobe resections can nevertheless be associated with permanent postoperative language deficits.
Thus, prediction of cortical language sites through classic anatomic criteria is inadequate, as there is significant individual variability of cortical organization,8–11 distortion of cerebral topography from tumor mass effect, and functional reorganization through plasticity mechanisms.12–14 A consistent finding of language stimulation studies has been the identification of significant individual variability among patients.10 Speech arrest is variably located and can go well beyond the classic anatomic boundaries of Broca’s area for motor speech. It typically involves an area contiguous with the face–motor cortex and, yet, in some cases is seen several centimeters from the sylvian fissure. This variability has also been suggested by studies designed to preoperatively predict the location of speech arrest based on the type of frontal opercular anatomy15 or using functional neuroimaging.16–22 Similarly, for temporal lobe language sites, one study of temporal lobe resections assisted by subdural grids demonstrated that the distance from the temporal pole to the area of language function varied from 3 to 9 cm.23 Functional imaging studies have also corroborated such variability.24 Furthermore, because functional tissue can be located within the tumor nidus,25 the standard surgical principle of debulking tumor from within to avoid neurologic deficits is not always safe. Consequently, the use of intraoperative cortical and subcortical stimulation to accurately detect functional regions and pathways is essential for safely removing dominant hemisphere gliomas to the greatest extent possible.
It is recommended that, for any tumor involving the dominant temporal, mid- to posterior frontal, and mid- to anterior parietal lobes, an awake craniotomy should be employed to identify language sites before the tumor is removed. Functional magnetic resonance imaging (fMRI) may also provide preoperative assessment of sensory and motor pathways and has been shown to be valuable in determining the rolandic cortex. This method is not reliable, however, for identifying language sites and does not provide an adequate replacement for intraoperative stimulation mapping.
Preoperative Assessment
The patient’s neurologic status should be assessed preoperatively to determine the extent of motor or language function impairment, if any. If the patient has severe hemiparesis or hemiplegia, motor mapping will often not be useful. However, if antigravity movements are present preoperatively, it is usually possible to stimulate both cortical and subcortical motor pathways intraoperatively. In children younger than 5 or 6 years of age, due to cortical electrical inexcitability, somatosensory evoked potentials (SSEPs) can be used to identify the central sulcus.
In addition to testing motor and sensory function, it is also imperative to assess the patient’s language function intraoperatively to determine if the baseline naming error rate is < 25%. Similarly, those patients who will undergo intraoperative mapping for language sites should be preoperatively tested for language errors by being presented a series of slides with common objects to be named. After confirming that the face–motor cortex and Broca’s area are functional by asking the patient to protrude the tongue and count to 10, slides of common objects are shown. Each slide will start with a phrase such as “This is a …” or “These are …” to test reading and speech output. Patients must be able to name common objects with less than a 25% baseline error rate, based on presenting each slide three times.
Pearl
• Preoperative steroids or neoadjuvant chemotherapy may improve naming, reading, and motor speech significantly enough to allow a patient with difficulty in these functions to be successfully mapped.
In patients who have moderate to severe dysphasia in either comprehension or expression, successful language mapping will not be possible. Therefore, this group of patients may either be operated on asleep, without any attempt to do more than an internal decompression, or challenged with steroids for 7 to l0 days and reevaluated regarding their baseline error rate in naming. An alternative approach may be to biopsy the tumor, confirm histopathology, and then treat the lesion with chemotherapy to reduce its size and induce functional improvement that will subsequently allow for intraoperative mapping.
In 85% of the population, the left hemisphere is dominant for language, whereas language representation is bilateral in 9%, and right-side dominance is present in only 6%. The dominant hemisphere is on the left for 98 to 99% of right-handed individuals. When in doubt, cerebral dominance may be verified using Wada’s (intracarotid amytal) test or estimated using fMRI or magnetic source imaging (MSI). On preoperative MRI, the central sulcus, and the motor strip that is located within the gyrus directly in front of it, is identified using the most cranial (rostral, superior) cuts of axial T2-weighted MRI. This landmark is a reliable marker for the motor cortex, regardless of mass effect, and allows one to predict where the functional motor region will be before surgery. On midsagittal and nearmidsagittal MRI, the rolandic (i.e., somatosensory-motor) cortex is identified by following the cingulate sulcus posteriorly and superiorly to its termination point. These MRI landmarks serve as useful guides to preoperatively determine the proximity of the lesion to the motor cortex.
Intraoperative Preparation
The patient is brought to the operating room and placed in the position appropriate for the area to be exposed. Special care is given to padding and protecting all extremities. A Foley catheter is inserted regardless of the need for osmotic diuretics. The head is fixed in position using a Mayfield clamp and local analgesia. The area of the scalp around the incision is infiltrated with a local anesthetic consisting of a 1:1 ratio of lidocaine (0.5%) to Marcaine (0.25%), combined with bicarbonate. A heating blanket is used to keep the core temperature above 36.5°C. If the patient’s temperature drifts too low, especially under general anesthesia, cortical stimulation mapping will be difficult due to cortical inhibition. An intravenous propofol drip maintains the sedative hypnotic anesthesia to keep the patient asleep. An alternative is dexmedetomidine, which lowers the risk of respiratory depression and therefore is advantageous for patients with potentially high intracranial pressure, although emergence from this agent is less rapid than from propofol. In case of a decrease in the arterial oxygen saturation, oxygen is administered through a nasal cannula. Prophylactic antibiotics are routinely used and given during the induction phase of anesthesia. Preoperative antiepileptics (e.g., 1 g fosphenytoin) are administered to minimize the risk of intraoperative seizures.
Intraoperative Stimulation of Cortical and Subcortical Pathways
In general, a limited craniotomy should expose the tumor and up to 2 cm of surrounding brain.
Prior to the dural opening, the patient should be awakened and encouraged to hyperventilate briefly in order to relax the brain. Using bipolar electrodes, cortical mapping is started at a low stimulus (1 mA per channel) and increased to a maximum of 6 mA, if necessary. A constant-current generator delivers biphasic square wave pulses (each phase, 1.25 ms) in 4-second trains at 60 Hz across 1-mm bipolar electrodes separated by 5 mm. Stimulation sites (approximately 10 to 20 per subject) can be marked with sterile numbered tickets. Throughout motor and language mapping, continuous electrocorticography should be used to monitor afterdischarge potentials and, therefore, eliminate the chance that speech or naming errors are caused by subclinical seizure activity.
This will abruptly stop the seizure activity originating from the irritated cortex without using short-acting barbiturates. The current necessary to evoke motor movement will vary depending on the anesthetic condition of the patient, with lower currents used under awake conditions. The motor strip is stimulated in the asleep patient with a starting current of 2 mA per channel, and reduced to 1 mA when stimulating the awake patient. The amplitude of the per-channel current is adjusted in 1- to 2-mA increments until motor movements are identified. A total current above 16 mA (8 mA per channel) has never been necessary to evoke sensory or motor response. Most commonly, the inferior aspect of the rolandic cortex is first identified by eliciting responses in the face and hand. As the leg motor cortex is tucked away against the falx, a strip electrode may be inserted along the falx, and stimulation using the same current applied to the lateral cortical surface may be delivered through it to evoke leg motor movements. This maneuver is safe due to lack of bridging veins between the falx and the leg motor cortex. Similarly, if the craniotomy is near but not overlying the rolandic cortex, a subdural strip electrode may be inserted under the dural edge and stimulated to evoke the desired response.
Pearl
• Because the dura is pain-sensitive, the area around the middle meningeal artery should be infiltrated with the lidocaine-Marcaine mixture to alleviate discomfort while the patient is awake.
• The best management of intraoperative stimulation-induced focal motor seizures or stimulation-induced afterdischarge potentials is rapid cortical irrigation at the stimulation site with ice-cold Ringer’s solution.
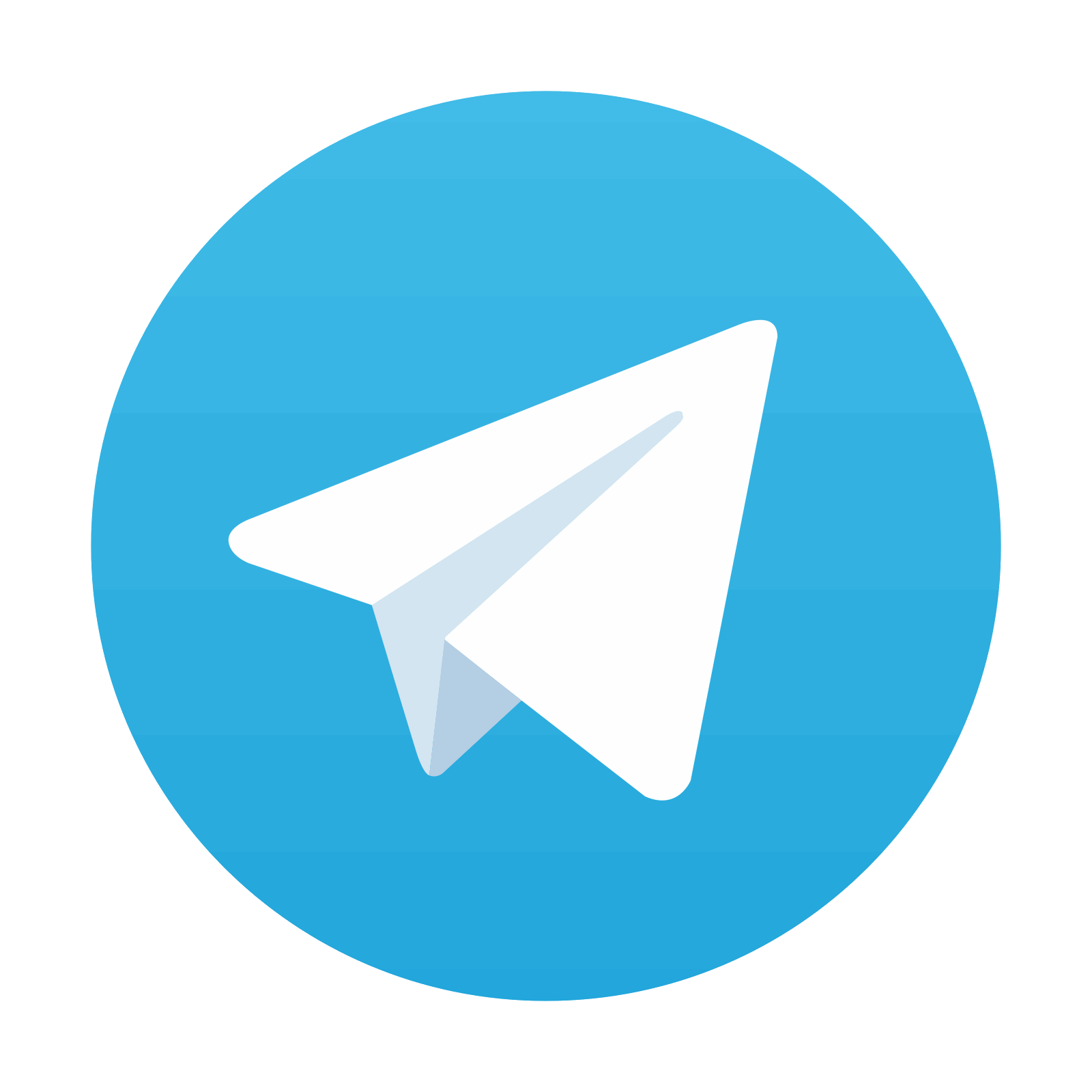
Stay updated, free articles. Join our Telegram channel

Full access? Get Clinical Tree
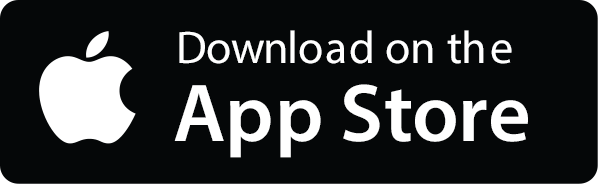
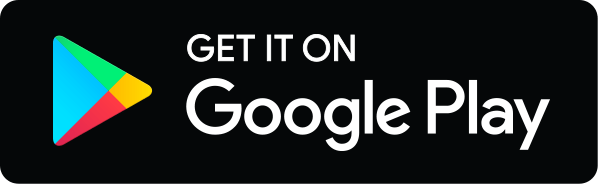