External Beam Radiation Treatment Planning
INTRODUCTION
The overarching goal of curative external beam radiation therapy planning is to create a plan that will comprehensively and consistently treat cancerous tissues without damaging uninvolved critical structures.
The steps involved in preparation for the planning process, including identification of gross target volumes (GTVs), clinical target volumes (CTVs), and internal target volumes (ITVs), were addressed in Chapters 4 and 5. In Chapter 7, we have discussed how to assure that the treatment is being delivered according to plan throughout its course. Chapter 6 focuses, in part, on the collaborative process that produces an optimized external beam treatment plan. The entire process of treatment plan creation and implementation requires a highly functioning team with all the characteristics discussed in Chapter 3. This team consists of a radiation oncologist, radiation therapy dosimetrist, radiation therapists, and a radiation physicist. Each member brings specialized expertise to the process and each helps to assure that high-quality treatment is administered safely. As with any multidisciplinary team, the quality of the end result depends on the quality of communication between team members. Figure 6.1 summarizes the steps involved in the development of a highquality treatment plan.
THE TREATMENT PLANNING DIRECTIVE
Before target volumes are finalized, the radiation oncologist should carefully double check each aspect of the patient’s contours (Table 5.2). For complex IMRT plans, peer review of the proposed planning directive and target volumes is recommended before the case is transmitted to a dosimetrist (Fig. 6.1). This evaluation can reduce the chance for error and can markedly improve the efficiency of the planning process by decreasing the need for subsequent revisions to the treatment plan.
Many factors influence the choice of planning target volumes (PTVs) and external beam technique. As the planning process progresses, some of these factors may be adjusted to meet technical and anatomic constraints. However, every plan should begin with a clearly articulated, precise treatment planning directive that is transmitted in writing from the radiation oncologist to the radiation dosimetrist. In this directive, the radiation oncologist should specify:
A list of CTVs and GTVs with designations that are matched precisely to the labels on the respective contours in the treatment planning system.
The margin(s) to be added to each GTV or CTV to generate a final PTV.
The desired dose and dose per fraction for each PTV.
Normal tissue constraints with emphasis on areas of particular concern.
A suggested field arrangement (e.g., four-field, AP-PA, or appositional electrons) or inverse planning approach (e.g., IMRT).
Information about previous treatments or areas that may receive additional exposure from sequential boosts or future brachytherapy.
If the skin is to be included in the target volume, this should be specified and the need for bolus discussed.
ACCOUNTING FOR UNCERTAINTIES—DESIGNATION OF THE PTV
Safe external beam radiation therapy requires a thorough understanding of the uncertainties underlying the treatment planning process and a recognition of the impact that even small errors can have on the ratio between the probabilities of tumor control and normal tissue complications. A failure to respect these uncertainties results in plans that look impressive but that fail to address the realities of neoplastic behavior and treatment delivery and that can easily lead to recurrence of disease inside or at the margins of the radiation treatment field (Figs. 6.2 and 6.3). Uncertainties that must be considered in the planning process include the following:
Uncertain boundaries of gross disease due to ambiguous diagnostic findings or distortion and degradation of reconstructed images. In general, these uncertainties are addressed in designation of the GTV.
Uncertainties about the extent of microscopic spread to lymph nodes or other tissues at risk for tumor involvement; these are addressed in the CTV.
Uncertainties caused by internal motion of target structures. These are usually incorporated in an ITV based on diagnostic findings and an understanding of the nature of internal organ motion (Chapter 5).
Uncertainties caused by inconsistent positioning of the patient (setup error). A PTV margin is typically added to the GTV, CTV, or ITV to account for these uncertainties.
![]() FIGURE 6.2 With IMRT, it is possible to achieve radiation dose distributions that conform tightly to unrealistic target volumes. This patient was referred for an infrarenal paraaortic node recurrence of endometrial cancer 2 years after completing adjuvant pelvic IMRT for a stage IAG2 endometrioid cancer. Only three central axis views of the doses from her previous treatment were available. However, this axial view suggests that her treatment was planned without sufficiently considering possible positioning uncertainties. The vaginal target was designated with no margin for internal organ motion or patient setup error. The patient also was simulated with a rigid obturator in the vagina; this is not recommended because such devices cause substantial vaginal distortion (Chapter 4). The distal obturator nodes also appear to have been excluded from the nodal target volume. Fortunately, the patient did not have a pelvic recurrence and has no evidence of disease 4 years after definitive treatment for her paraaortic node recurrence. |
Many of these issues are discussed in Chapter 5. The first section of this chapter focuses primarily on the last group of uncertainties, external factors that can cause the distribution of radiation dose within the patient to differ from that depicted in the radiation therapy treatment plan. The size of the PTV margin depends on the magnitude of these uncertainties, their impact on the delivered radiation dose, and on the probability of an adverse outcome if the actual dose that is delivered to the tumor is less than the prescribed dose. In the following section, factors that are used to determine a safe PTV margin are discussed with recommendations regarding the size of the PTV margin to be used under various conditions.
The Relationship between Radiation Dose and the Probability of Tumor Control
The range of doses that can achieve complication-free cure of gynecologic cancers is narrow, particularly if gross disease is present. Although high-quality dose response analyses are not available for external beam radiotherapy of gynecologic carcinomas, analyses of other tumor sites suggest that there is a very steep relationship between radiation dose and the probability of controlling macroscopic disease. Analyses of patients with gross lymph node metastases from head and neck cancers indicate that the likelihood of tumor control increases very steeply with increasing radiation doses between 55 and 65 Gy; within this range, the probability of tumor control appears to increase by at least 4% to 6% per 1 Gy of radiation added (Fig. 6.4).1,2,3 If anything, these early analyses may have underestimated the steepness of the dose-response relationship because radiation treatment plans that were generated without image guidance may have overestimated the dose delivered to gross nodal disease in some cases.
For the past 20 years, the range of doses used at MD Anderson to treat gross lymph node metastases from gynecologic cancers has been too narrow to permit a meaningful analysis of the relationship between dose and tumor response. However, in the 1980s, patients with gross paraaortic node metastases from cervical cancer usually received only 55 Gy because previous studies had demonstrated unacceptable complication rates when extended fields were treated to a dose of 60 Gy. A retrospective review of patients treated during this period demonstrated a high rate of paraaortic recurrence. In contrast, after the introduction of PET imaging, IMRT (using policies described in this book), and image guidance, nodal metastases were routinely boosted to at least
60 Gy; with these changes, the rate of infield paraaortic nodal recurrence dropped dramatically to <5%.4 These results also suggest that there is a very steep relationship between radiation dose and the likelihood of tumor control.
60 Gy; with these changes, the rate of infield paraaortic nodal recurrence dropped dramatically to <5%.4 These results also suggest that there is a very steep relationship between radiation dose and the likelihood of tumor control.
The probability of bowel complications also tends to be strongly correlated with the dose of radiation, with a steep dose-effect relationship between 40 and 55 Gy, when large volumes of bowel are irradiated, and between 60 and 70 Gy, when small volumes (e.g., single loops of bowel) are treated. Because the doses required for tumor control can also cause significant side effects in normal tissues, highly conformal treatment plans that minimize the volume of incidentally irradiated normal tissue are likely to be of value.
However, although IMRT, image guidance, and other modern advances have increased the precision of radiation therapy delivery, they have not eliminated positional uncertainties. The steep relationship between the dose of radiation and the probability of recurrence means that even a few treatments that fail to encompass a site of active tumor could increase the probability of recurrence. Because even the best immobilization and localization systems cannot eliminate uncertainty, it is critical to understand the limitations of our techniques and to build in safety margins that minimize the chance that a target will receive less than the prescribed dose. Incremental sparing of normal tissues at the expense of tumor recurrence is generally not an acceptable trade-off.
Factors that Influence the Reproducibility of Daily Treatment Setup
Although modern radiation therapy techniques have improved the reproducibility of treatment setup and delivery, residual uncertainties continue to demand a margin for error.
One of the most important sources of uncertainty is day-today variation in the position of the patient. Kim et al.5 evaluated the daily accuracy of clinical laser setups in 52 women treated with RT for gynecologic cancers; patients in their study were positioned with their feet together and a sponge under their knees. Accuracy of the initial setup was assessed using onboard KV imaging or cone beam CT to determined the shifts required to bring the patient’s isocenter into correct alignment with adjacent boney landmarks. In general, right-to-left directional errors were greater than anterior-posterior (AP) or superior-inferior errors, probably reflecting the fact that only one skin mark is available for right-left alignment, while two lateral points of reference are used to align the patient in other directions.5 Based on their determination of the frequency of random and systematic errors, the authors used the method of van Herk6 to estimate required CTV-to-PTV margins of between 7 and 10.5 mm. In a similar study, Santanam et al.7 concluded that a CTV-to-PTV margin of 7 mm was needed. However, it should be noted that both of these calculations were based strictly on the translational table shifts required to bring the isocenter into to the correct position. Possible rotational and tilt errors were not considered in these analyses.
Several factors can influence the magnitude of positional errors:
Body habitus. In their analysis, Kim et al.5 reported a significant correlation between a patient’s body habitus and the magnitude of positional errors.
Field size. Studies of pelvic setup reproducibility have focused on the translational shifts that are required to bring the isocenter into the correct position. However, as demonstrated in Figures 7.5 and 7.6, tilt errors and rotational errors can be equally important sources of errors in the setup of patients who have large fields. A mere 2-degree right-to-left tilt error will cause a shift of 5 mm at 15 cm from the isocenter and a shift of 7 mm at 20 cm (Fig. 6.5). Right-to-left rotational errors (Fig. 7.6) can also result in underdosage, particularly of presacral or inguinal targets. Because these errors are much more difficult to detect and correct than translational errors, they remain a significant problem even when treatments are set up using daily image guidance.
Immobilization method. In a comparison of several different pelvic immobilization methods, Malone et al.8 reported very little difference between the mean isocenter shifts required to achieve pubic alignment of prostate cancer patients treated in generic leg support devices (similar to that shown in Fig. 4.10a) or molded devices (similar to that shown in Fig. 4.10c). However, patients who were immobilized in HipFix thermoplastic devices were less likely to require large error shifts than patients immobilized in the other two types of devices. The ability to mark the isocenter directly on this type of device is a relative advantage of the thermoplastic device. The authors of this Canadian study did not report whether the device was equally effective for obese or thin patients.
Image guidance. The practical application of image guidance is addressed in Chapter 7. Daily image guidance is an important tool that significantly reduces the magnitude of alignment errors. However, image guidance does not eliminate all setup errors for several reasons:
Even the most acute observer cannot always detect 1- to 2-mm errors in isocenter alignment and will occasionally miss even 3- to 4-mm errors, particularly if the isocenter is not close to a clearly visualized boney landmark.
The relatively poor image quality and low resolution of reconstructed pelvic images and cone beam CTs often makes it difficult to accurately identify the borders of key landmarks.
As discussed above, rotations and tilts are important sources of potential errors in gynecologic treatments; these are easily missed during review of onboard images that have a limited field of view (Fig. 6.5). These complex positioning errors can also be difficult and time consuming to correct, particularly if the entire spine and pelvis must be brought into alignment; this process can easily exceed the patient’s tolerance.
Sources of Inaccuracy in the Treatment Machine
The Science Council of the American Association of Physicist in Medicine (AAPM) has published quality assurance guidelines for medical linear accelerators.9 Their recommendations included tolerance standards for the accuracy of localization lasers on the simulator and treatment machines (±1 mm for IMRT; ±2 mm for non-IMRT), accuracy of the port film graticule tray (±2 mm), coordinance of the onboard imaging and treatment coordinates (≤1 mm for stereotactic body RT [SBRT]; ≤2 mm for non-SBRT), and other features.9 With recommended daily and monthly monitoring routines, systematic errors should not occur because of these uncertainties. However, they do make a finite contribution to the overall uncertainties that must be considered in the determination of a PTV margin.
Other Sources of Uncertainty
Other factors can introduce small variations in the target position. Breathing is not a major cause of target motion for most gynecologic cancer treatments; however, the breathing cycle can cause small changes in the position of the upper abdominal spine and nodes. Patients may clench their gluteal or perineal muscles during treatment; these actions can displace distal target structures by several millimeters.
Changes in hydration and blood pressure cause small changes in the diameter of the inferior vena cava and other major veins, potentially displacing adjacent lymph nodes. Some accounting for these variations is considered in the designation of the nodal CTV, but the PTV margin adds an additional margin of safety. It is important to note that injection of intravenous contrast can cause much more dramatic expansion of venous structures than normal hemodynamic variations; as discussed in Chapter 4, we do not recommend contrast administration during simulation to avoid this potential source of systematic error.
Recommended CTV-to-PTV Margins
Recommended target-to-PTV margins for 3-D conformal and IMRT treatments are summarized in Table 6.1.
Note that a somewhat smaller GTV-to-PTV margin is recommended when the GTV boost is integrated within a larger CTV. This is because the steepness of the dose gradient
surrounding the GTV is less if the GTV is surrounded by a larger CTV-PTV that receives only a slightly lower dose than the GTV. This is also the rationale for occasional use of nested GTVs to treat large tumors that are close to critical structures (Chapter 5; CS 10.11, 14.1, 14.8, 15.1, 15.2).
surrounding the GTV is less if the GTV is surrounded by a larger CTV-PTV that receives only a slightly lower dose than the GTV. This is also the rationale for occasional use of nested GTVs to treat large tumors that are close to critical structures (Chapter 5; CS 10.11, 14.1, 14.8, 15.1, 15.2).
TABLE 6.1 Recommended CTV/GTV/ITV to PTV Margins for Radiation Therapy Planninga | |||||||||||||||||||||||||||
---|---|---|---|---|---|---|---|---|---|---|---|---|---|---|---|---|---|---|---|---|---|---|---|---|---|---|---|
|
NORMAL TISSUE CONSTRAINTS FOR EXTERNAL BEAM PLANNING
Although the primary objective of definitive radiation therapy is to cure the patient’s cancer, the goal is always to accomplish this with as few side effects as possible. Because most gynecologic cancers involve or are in direct contact with critical structures, because even the best immobilization and image guidance techniques do not obviate the need for a PTV margin, and because every radiation beam passes through some normal tissues on its way to the target, a high probability of cure cannot be achieved without at least some risk of causing acute or late side effects. However, a well-designed treatment plan that minimizes the dose of radiation to structures outside the PTV can undoubtedly reduce the probability of life-altering side effects.
Although many investigators have attempted to correlate radiation dose, radiation volume, and radiation-related side effects, it is very difficult to generate practical guidelines from the results. Some of the factors that contribute to this include the following:
For most studies, a single CT was used to calculate organ doses and volumes. These calculations do not accurately represent the doses delivered to mobile critical structures during a protracted course of radiation treatment.
Inconsistent methods have been used to designate normal tissue volumes.
Results that were based on site-specific multimodality treatments for nongynecologic cancers may not be broadly applicable to gynecologic cancer patients.
Many factors other than dose and volume contribute to the risks of side effects (Chapter 9).
The level of risk that is considered acceptable may vary according to the clinical situation (i.e., the potential benefit of a treatment).
Some general guidelines are discussed in the following sections. Where data are available to suggest very specific radiation dose or volume constraints (e.g., for kidney or duodenum), these will be emphasized. However, the goal should always be to conform the radiation dose as tightly as possible to the PTV in order to minimize the dose to uninvolved critical structures.
Bladder
In the course of four-field pelvic RT, most of the bladder usually receives the prescribed dose of 45 to 50 Gy. Although this appears to be tolerated fairly well, even 45 Gy has been associated with short- and long-term side effects (Chapters 7 and 9). Boothe et al.10 demonstrated markedly decreased rates of urinary tract infection in patients treated to the whole pelvis with IMRT versus four-field conformal treatment. The lack of data and the variable volume of the bladder make it difficult to make strict recommendations regarding the volume that should receive <45 Gy. However, with IMRT, it is often possible to reduce the volume of bladder receiving 30 Gy or more to <50%, particularly if the bladder is full during treatment (Figs. 6.6 and 6.8).
Patients who require a high dose of radiation dose to treat gross vaginal disease usually receive a similarly high dose to at least a portion of the bladder. Most of the data concerning the tolerance of the bladder to external beam doses of more than 65 Gy are based on studies of prostate cancer treatment11; based on these results, it has been recommended that no more than 50% of the bladder receive more than 65 Gy and no more than 35% receive more than 70 Gy.11 Patients who receive these relatively high doses should be treated with a full bladder (at least 200 cc, if possible).
Dose recommendations for patients receiving brachytherapy are summarized in Chapter 8.
Rectum
The rectal contour should extend from the anal verge superiorly to the point where it looses its round shape in the axial plane and turns toward the sigmoid.12
Because the rectum is intimately associated with common cervical and vaginal targets, it is rarely possible to prevent at least 50% to 70% from receiving at least 45 Gy. Fortunately, this dose is not often associated with major side effects. When pararectal targets require higher doses, an effort should be made not to treat more than 25% of the rectum to >65 Gy. It may be necessary to treat a larger volume to high dose in some cases (e.g., CS 15.2); however, the patient should be warned that the risk of radiation proctitis or stricture may be greater than usual.
Bowel
Contouring Methods
Although some clinicians contour individual loops to create a bowel avoidance structure, this method is unrealistic because bowel is constantly moving within the peritoneal cavity. A more efficient and, probably, equally valid method is to contour small and large intraperitoneal bowel as a single organ or “bowel bag.” In obese patients, fat at the root of the mesentery and in the retroperitoneal space may displace bowel away from the iliac or paraaortic nodes; it is reasonable to exclude this region from the contour if it contains no bowel. The rectosigmoid is usually contoured separately from the bowel bag. To allow closer scrutiny, in some cases, a separate contour may be designated for a loop of bowel that appears to be fixed in a critical location (e.g., adjacent to a site of gross disease).
Dose Constraints
Because intraperitoneal bowel is usually moving in and out of any specific location, small portions of the bowel bag can usually be treated to 60 to 66 Gy without causing major complications; however, if a portion of the bowel is fixed by scarring or adhesions, the risk of obstruction is greater; if possible, an effort should be made not to treat the entire circumference of a fixed bowel loop to more than 60 Gy.
If a bowel obstruction does occur, surgical resection is easier and less likely to have long-term consequences if the rest of the bowel is healthy. For patients who require 60 Gy or more to a portion of bowel, it is particularly important to minimize the volume of adjacent bowel that receives intermediate doses of 35 to 50 Gy. Whenever possible, IMRT is recommended for such cases.
Although there are very few data available, it is likely that the side effects of large volume RT on bowel increase fairly steeply between 45 and 55 Gy.13 In most cases, we do not recommend treating the whole pelvis to more than 45 Gy with AP-PA or four-field techniques because our data suggest that higher doses may be associated with a higher incidence of serious late effects.13 If available, IMRT is recommended if a large pelvic volume requires more than 45 Gy (CS 10.1).
Duodenum
At least the second and third portions of the duodenum should be contoured if paraaortic nodes are included in the target volume. The second (descending) portion of the duodenum is located to the right of the L1 to L3 vertebral bodies and the inferior vena cava; it then turns medially at about L3 (where it becomes the third part of the duodenum) and then passes anterior to the vena cava and aorta until it travels superiorly to the left of L3. Coronal views are typically very helpful for contouring the duodenum.14
It is recommended that no more than 15 cc of the duodenum receive more than 55 Gy and that no more than 2 cc receive more than 60 Gy.15
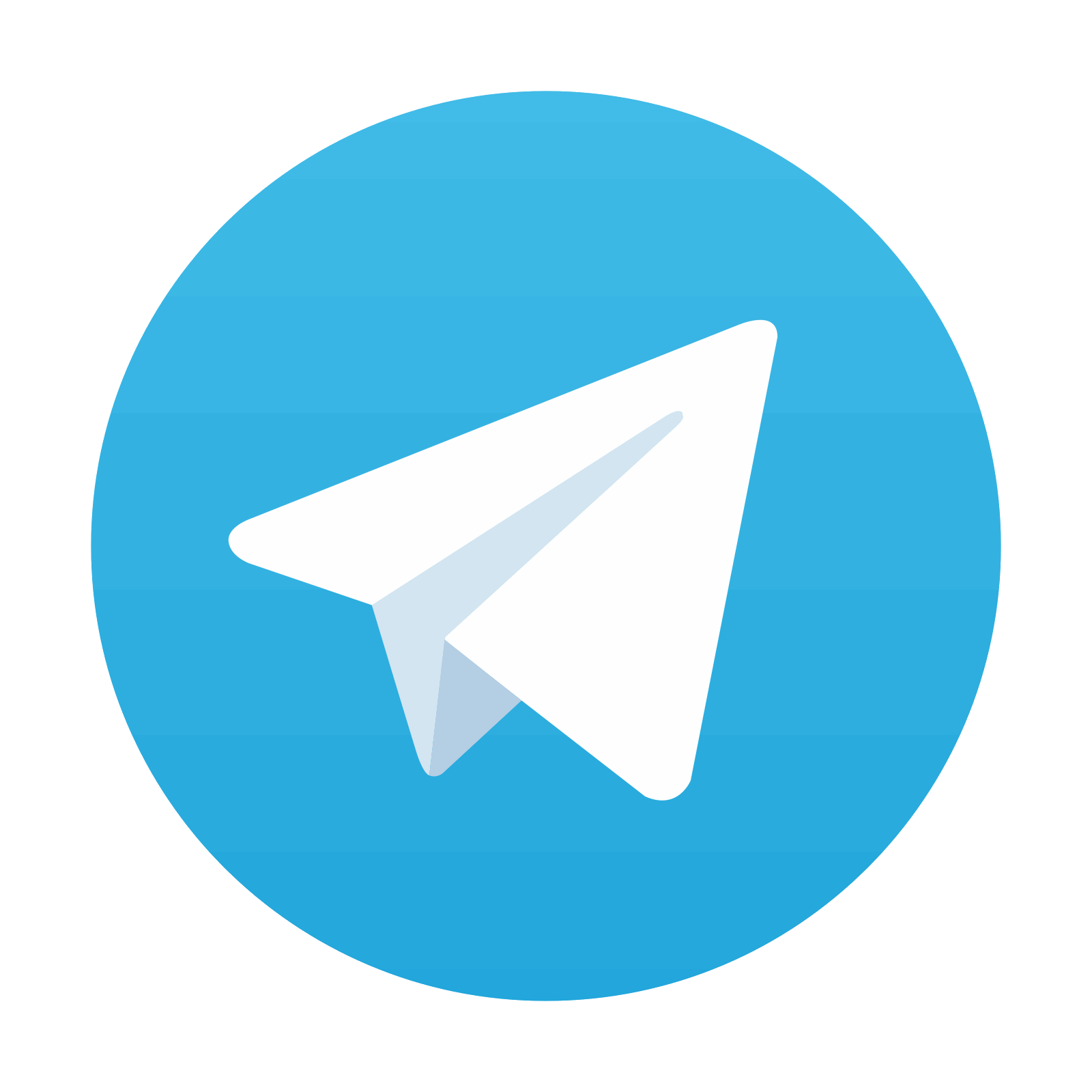
Stay updated, free articles. Join our Telegram channel

Full access? Get Clinical Tree
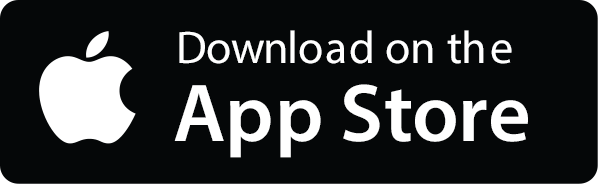
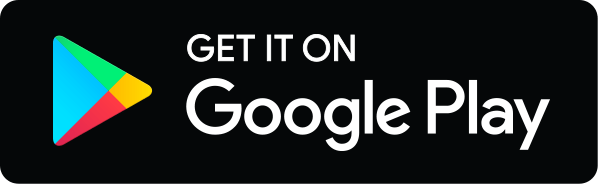