Aging, Disuse, and Disease
A common belief among the lay public, as well as among many health-care professionals, is that much of the disease and loss of function that commonly accompanies aging is inevitable and a result of the “aging process” itself. However, it has become clear that much of the physical decline and reduced physiological reserve previously blamed on aging is, in fact, caused by the complex interactions of true genetically determined aging, disease (often subtle or subclinical), disuse, and environmental exposure.
The myriad of possible interrelationships among these factors makes it difficult to ascribe specific causality for the loss of physical vigor or function. Thus, for example, preconceived societal notions about aging may predispose to greatly reduced expectations with regard to physical as well as mental performance. Such preconceptions may promote inactivity and disuse in women at an even earlier age than in men. With years of ensuing inactivity, disuse not only exaggerates and enhances any true age-related loss of endurance, strength, and flexibility, leading to further inactivity and disuse, but may also exacerbate previously subtle or subclinical diseases such as intra-abdominal obesity, glucose intolerance, osteopenia, hypertension, dyslipidemia, and coronary artery disease. These physiological disorders, the drugs used in their treatments, and the associated functional impairments and disability can, in turn, further limit activity and continue the vicious downhill spiral.
Physical activity level (and measured fitness) appears to be inversely related to the risk of mortality and is associated with a greater average life span (approximately 2 years in human studies). An inverse dose–response relationship has also been noted between physical activity and the risk of developing many important diseases (cardiovascular disease [CVD], stroke, hypertension, type 2 diabetes, osteoporosis, obesity, colon and breast cancer, anxiety, and depression). Many of these reports included older adults. Despite the clear advantages to physical activity, less than 40% of persons 65 years and older meet the CDC recommendations for activity, and this percentage falls significantly further in older age groups.
This chapter reviews the physiological effects of aging and exercise training on the most common measures of physical fitness: (1) endurance or maximal aerobic exercise capacity, (2) skeletal muscle strength and power, and (3) body composition. Next, it investigates the theoretical relationship between fitness and functional status, reviewing the available, albeit somewhat limited, data on the effects of increased activity on functional performance. The chapter then reviews the effects of aging and activity on disorders commonly observed in geriatric patients. Last, the risks associated with exercising are discussed and some suggestions are made with respect to prescribing an exercise program for older individuals.
While we and others commonly discuss health benefits of “exercise training,” it is now abundantly clear that many benefits can be accrued simply through a more active (nonsedentary) lifestyle in the absence of formal “exercise training.” This concept may be especially helpful in trying to encourage older individuals who feel unable or unwilling to engage in formal exercise training but can and will increase their physical activity.
Aging and Exercise
The best physiological measure of endurance work capacity is the amount of oxygen consumed at maximal exercise (maximal aerobic power or Vo2max). Cross-sectional studies have repeatedly demonstrated a significant age-related decrement in Vo2max in both women and men. Together, these cross-sectional data suggest that exercise capacity declines by approximately 1% per year, when Vo2max is expressed as milliliters of oxygen consumed per minute per kilogram of body weight (e.g., mL/min/kg). Longitudinal data in this area are somewhat more confusing, probably owing to (1) variation in the initial level of fitness of the subject populations, (2) spontaneous modifications in activity level between test periods, (3) alterations in body weight and composition, and (4) intervening illness. It is possible that the data are further influenced by a nonlinear decline over time (Figure 114-1), with a more rapid decline in Vo2max in early adulthood in sedentary individuals followed by a less steep decline later in life. Longitudinal studies in both men and women demonstrate approximately a 0.5 mL/min/kg decline per year after a 4-year follow-up period. Almost half of this decrement could be accounted for by independent changes in adiposity and self-reported physical activity.
Both cross-sectional and longitudinal approaches have been used to determine whether habitual endurance training slows the age-related decline in Vo2max. Cross-sectional comparisons of younger and older endurance athletes and normally active controls indicate that the slope of the regression line for age-related decline in Vo2max is not as steep in athletes as in their more sedentary counterparts. In fact, these comparisons suggest that habitual exercise may attenuate the rate of decline in Vo2max with aging by up to 50% (0.5% vs. 1.0% per year). Longitudinal studies of older endurance-trained men, followed for more than 10 to 22 years, indicate that the slower decline in Vo2max occurs only in men who continue to train vigorously for competition. One study followed both sedentary and trained men, and the average rate of decline in Vo2max was higher in the athletes than in the nonathletes (−2.9% vs. −1.5% per year). When athletes were characterized by the level of exercise training they maintained, the rate of decline was −0.3% per year in those who trained vigorously, −2.6% per year in those who trained moderately, and −4.6% per year in the low-training group. However, even in the low-training group, Vo2max remained higher at follow-up (33.8 mL/min/kg; age 74 years) than in the sedentary controls (25.8 mL/min/kg; age 70 years). Thus, the higher fitness levels of active individuals may afford them protection later in life, by allowing them to remain above the threshold of exercise capacity necessary to remain functionally active.
Vo2max is equal to the product of maximal cardiac output (Qmax) and the maximal ability of muscle to extract oxygen from the blood (a-Vo2 difference) and, thus, is determined by both central (cardiovascular) and peripheral (primarily muscle) components. Studies have uniformly detected important changes in body composition associated with aging (see the following section on body composition), including increases in fat mass and decreases in fat-free mass (FFM) or lean body mass (LBM). Furthermore, with aging, muscle mass comprises a lesser percentage of what is usually measured as FFM. Several studies of carefully screened older subjects confirm that differences in FFM can explain some of the age-related differences in Vo2max. In a cross-sectional study of very healthy men and women, muscle mass, as reflected by 24-hour creatinine excretion, was found to decline 23% between ages 30 and 70 years. When normalized for this decrease in muscle mass, the slope of the decline in Vo2max with age flattened significantly, and the predicted age-related decline in Vo2max between the ages of 30 and 70 years was reduced in both men (39% vs. 18%) and women (30% vs. 14%). Approximately half of the original age-related decline in Vo2max could be explained by the age-associated loss of muscle mass. Other peripheral mechanisms might also account for some of the age-related decrement in Vo2max by attenuating the a-Vo2 difference, including a reduced ability to direct blood flow to the working muscles or a diminished ability of muscle cells in older (inactive) individuals to use oxygen.
Declines in the central (cardiovascular) component of endurance capacity contribute substantially to the age-related reduction in Vo2max. In the absence of hypertension or coronary artery disease (clinical or asymptomatic), resting cardiac output, heart rate, and heart size are normal in older individuals. However, as illustrated in Figure 114-2, a number of age-related changes in resting cardiac function have been defined. These cardiovascular changes detected at rest in the elderly person are quite similar to those observed with hypertension. Indeed, it has been hypothesized that mild vascular stiffness and ensuing subclinical hypertension may initiate this group of compensatory responses noted at rest.
As with most age-related abnormalities, alterations in cardiovascular physiology are most apparent and most clinically relevant during stress, such as physical exercise. In the setting of maximal exercise, striking differences between young and older individuals are apparent. By far the most salient and consistent is a marked decline in maximal heart rate with age. However, beyond this one finding, there is little agreement. While some studies suggest that most of the age-related decrement in Vo2max is explained by a decline in cardiac output, others detect little decrease in maximal cardiac output with age. In fact, the majority of studies now support the concept that diminished central responses to maximal exercise substantially contribute to the reduced Vo2max with aging. The degree to which this defect can be explained by a lower maximal heart rate or a reduced stroke volume response to exercise remains unclear.
Certain individuals (e.g., master athletes) can maintain a reasonably high endurance exercise capacity well into old age. It is now clear that the fitness response to an endurance training program in previously sedentary, healthy older individuals is comparable to that in younger subjects. Depending on the type and duration of exercise employed, the improvement in Vo2max typically varies between 10% and 30%, with similar responses in both men and women. Furthermore, when young and older subjects are trained in the same program at the same relative intensity, the relative increments in Vo2max are similar.
Healthy older individuals can tolerate carefully supervised endurance training at relatively intense levels (e.g., 85% of heart rate reserve; HRR = 0.85 [(HR max – HR rest) + HR rest]) with acceptable attrition rates and few, if any, significant injuries. Indeed, it appears that speed is a more important determinant of injury than the actual overall intensity of the exercise. However, few older individuals choose to maintain this rigorous training intensity on their own, and a clinically more salient question is whether qualitatively similar adaptive responses can occur with lower-intensity training. Although not all studies agree, it appears that cardiovascular improvement can be obtained with moderate-intensity programs, where subjects train at approximately 50% of heart rate reserve. In addition, metabolic improvements can occur with low- to moderate-intensity exercise that is maintained over a sufficient period of time (see below). New recommendations suggest that multiple short (10 min) bouts of exercise may be comparable to a single larger training session.
As noted above, with aging there is a significant loss of FFM or LBM, mostly accounted for by a reduction in skeletal muscle mass. This decline has been estimated to be approximately 6% per decade between ages 30 and 80 years, although recent studies have suggested slower rates of decline. It is possible that the increasing prevalence of obesity has a “preserving” effect on LBM. Despite the loss of LBM with aging, body weight is sustained or increased as a result of the accumulation of adipose tissue. In fact, excess body fat is a stronger determinant of impaired physical function of older adults than is inadequate LBM. More importantly, with aging, fat is preferentially accumulated in a central distribution. This centralization of body fat seems to occur continuously with age (after puberty) in adult men, but, in women, a significant increase in central adiposity first occurs following menopause. This central distribution of adipose tissue mass is a major risk factor for many obesity- and age-related metabolic abnormalities and, in many studies, is independent of relative weight or other measures of obesity.
In cross-sectional studies comparing highly physically active older individuals with more sedentary controls, overall adiposity in the active group is consistently lower and, in fact, similar to younger individuals. This is true for both women and men, with the physically active groups having approximately 10% less body fat than sedentary controls. Furthermore, there is an inverse correlation between Vo2max and central adiposity, as defined by waist-to-hip ratio, within a population of healthy older men. However, relatively little difference in FFM or LBM has been observed in active, as compared to sedentary, healthy elderly persons. Therefore, even highly endurance-trained older individuals have significantly less LBM than do younger individuals.
Longitudinal endurance training studies in previously sedentary individuals support these cross-sectional findings. Endurance training has consistently produced small but significant decrements in percent body fat and overall fat mass in older men and women. In two studies, a loss of 2.5% body fat (1.5–3 kg of fat mass) followed a 6- to 12-month intensive endurance-training program in older subjects. Associated with this modest decrement in overall adiposity was a preferential decrease in the central distribution of fat. In fact, following endurance training in older men, there was a small fall in waist-to-hip ratio, but a 20% decline in intra-abdominal fat as measured by computed tomography. In contrast to the modest but consistent decrements in adiposity, little or no change in overall FFM has been detected following endurance training in the elderly population, even when it is quite vigorous. The lack of improvement in overall FFM may be countered by significant changes in specific muscle groups. For example, cross-sectional mid-thigh muscle mass, measured by computed tomography, was found to increase by 10% in older men, despite no change in overall FFM. This finding suggests a redistribution of FFM with intensive endurance training in older men. The lack of accretion of FFM with endurance exercise in older individuals may reflect a reduced anabolic hormonal milieu, with lower levels of sex steroids, growth hormone, and insulin-like growth factor I.
Although highly trained athletes are leaner than less-active controls, the loss of weight or fat with endurance training is small when compared to dieting with caloric restriction. However, as noted above, the effects of exercise may be magnified by the preferential loss of central, and specifically intra-abdominal, fat with endurance training. Endurance training can increase the usually low resting metabolic rate in the elderly person by approximately 10%, even when corrected for any observed change in FFM. It should be noted that vigorous exercise in older subjects might be associated with a compensatory decrement in activity during nonexercising periods and, thus, no increment in overall energy expenditure. Concomitant exercise training was found to be associated with a lower rate of long-term recidivism in subjects participating in a dietary weight reduction program. Exercise may mitigate the drop in resting metabolic rate, lipolysis, and fat oxidation that usually accompany dietary caloric restriction, which would otherwise lead to rapid regain of lost weight. While there are fewer data available, it appears that strength training has many of the same effects of endurance training on adiposity.
A preliminary report of 10 days of continuous bed rest in healthy older subjects dramatizes the importance of activity and the tremendous costs of being sedentary. After even such a short period of time, healthy older subjects experienced a 30% reduction in muscle protein synthesis, a 1.5 kg loss of lean mass (most from the lower extremities), and a 16% decrement in lower extremity strength.
Although cross-sectional studies suggest a significant age-related decline in cardiac output (see previous discussion), training status appears to positively affect this end point, with a greater maximal cardiac output found in well-trained older men and women when compared to matched sedentary controls. Most of the higher Vo2max in trained older individuals can be explained by their higher maximal cardiac output. In turn, a larger stroke volume explains the higher cardiac output, whereas no difference in maximal heart rate is detected between trained and untrained individuals. The relation between Vo2max and left ventricular performance seems to be linear over a wide range of fitness levels, suggesting that similar improvements could be expected with training, no matter what the initial fitness level.
Longitudinal studies of endurance training in previously sedentary older subjects have provided conflicting results on cardiac function. Initially, some found no improvement in estimated cardiac output following either low- or high-intensity endurance training. However, studies using more sensitive methods and more vigorous exercise training interventions provided evidence of enhanced left ventricular systolic and diastolic function. There is some evidence for a sex- and age-specific difference in the left ventricular response to endurance training, with an improvement in diastolic function demonstrated in young and older men and possibly only in young women. Others have been unable to show significant differences in left ventricular diastolic filling dynamics between older trained and sedentary men, with both being reduced compared to young controls.
The finding in some studies that older women do not increase maximal cardiac output in response to endurance exercise training, but that older men and young women and men do, raises the question of whether estrogens could be involved in this adaptation. When older women, either on or not on estrogen-based hormone therapy, underwent a 20-week endurance exercise program of stationary cycling, both groups of women had similar increases in estimated cardiac output at peak exercise in response to training. This contradicts previous findings, but it should be noted that the methodology used to evaluate cardiac function was not as sophisticated in this study as in the others, which found that maximal cardiac output did not increase in older women not on hormone therapy. The role that estrogens play in the cardiovascular adaptations to exercise, therefore, remains uncertain.
Vascular aging, including a decrease in large elastic artery compliance (increased stiffness) and vascular endothelial dysfunction, is a major independent risk factor for age-associated CVD in both women and men. In sedentary adults, arterial compliance and endothelial function decreases with advancing age even in the absence of clinical CVD. Cross-sectional studies demonstrate that the age-related loss of arterial compliance is attenuated in middle-aged and older endurance-trained men. Endurance training also mitigates the negative effects of estrogen deficiency on arterial compliance in postmenopausal women. Additionally, vascular endothelial function is preserved in endurance-trained men. Longitudinal studies demonstrate that short-term aerobic exercise programs (e.g., walking) can restore some of the age-associated loss of arterial compliance and completely restore endothelial function in previously sedentary middle-aged and older men. In previously sedentary postmenopausal women who chronically take hormone therapy, short-term aerobic exercise can restore arterial compliance to premenopausal levels.
Skeletal Muscle Strength and Power
Strength can be defined as the maximum force exerted by a muscle, and power is the rate of force development. Strength and power are not determined by muscle mass alone but also by intact neurological function. Consequently, as discussed in more detail below, strength and power can be increased by improving muscular function (e.g., muscle cell hypertrophy) or by improving neurological function (e.g., learning).
Strength assessment depends on the conditions of measurement, specifically the speed of muscular contraction, whether the muscle is shortening (concentric) or lengthening (eccentric) during the contraction, and the conditions of mechanical leverage. Muscle strength can be measured during isometric, isotonic, or isokinetic contractile activity. Isometric strength is the force generated against a fixed object, and isotonic strength is the force generated against a moving object (i.e., free weights and most resistance-exercise machines). Isokinetic strength also involves the generating force against a moving object, but, in this case, the speed of movement is controlled and constant. Muscle power can be determined simultaneously with the assessment of either isotonic or isokinetic strength by measuring the speed of movement.
For more than 100 years, cross-sectional studies have demonstrated that strength declines with age. After peak strength is reached sometime around the age of 40 years, cross-sectional studies show approximately a 30% to 40% reduction in strength by the age of 80 years. Longitudinal studies suggest that the true rate of decline may be underestimated by the cross-sectional data. For example, a longitudinal study estimated a 60% loss of handgrip strength between ages 30 and 80 years, while a cross-sectional analysis of the same data estimated loss at only 40%. Strength loss appears even more rapid at greater ages. Longitudinal studies have found approximately a 10% to 25% loss in quadriceps strength within only 5 to 7 years in 70-year-old adults. In the majority of these studies, muscle power was not measured. However, there is growing evidence that the rate of decline in muscle power is even greater than the decline in strength. Measurements of muscle strength and power for more than 10 years in the Baltimore Longitudinal Study on Aging indicated that the decline in muscle power was approximately 10% greater than the decline in strength.
It is now clear that the decline in muscle power is a stronger determinant than muscle strength of physical functional status in old age. This is not surprising if the decline in power is, indeed, more pronounced than the decline in strength. Leg muscle power was reduced in older women prone to falling, as compared with nonfallers, and was a stronger predictor than strength of self-reported functional status in elderly women. These findings suggest that intervention strategies should be aimed not only at preserving muscle mass and strength with aging, but also muscle power.
A close relationship exists between the size of a muscle, measured as cross-sectional area, and its ability to generate force. One would predict, then, that a 40% decline in muscle strength should be accompanied by a decline in muscle mass of a roughly equal amount—approximately 30% to 40%. This is the case, and, just as strength loss is more rapid at greater ages, loss of muscle mass is also reported as becoming more rapid with age. In laboratory animals, there is also an age-related decrease in muscle-specific force, which is the peak force per cross-sectional area. Single muscle fiber characteristics of tissue obtained from younger and older men and older women also indicate that the reduction in the capacity to generate force with aging is not solely a result of the reduction in fiber size or muscle mass and raise questions about how muscle fiber function and composition change with aging.
Skeletal muscle is comprised of groups of individual muscle fibers. These fibers contain myofibrils, which are, in turn, composed of a number of myofilaments. The myofilaments contain the proteins actin and myosin and are arranged to form sarcomeres—the contractile units of muscle.
There are two major types of muscle fibers: slow twitch and fast twitch. Furthermore, there are at least three subtypes of fast-twitch fibers: a, b, and c. The fast-twitch fibers are variously referred to either as FTa, FTb, and FTc or as type 2a, type 2b, and type 2c. The slow-twitch fibers are referred to as ST or type 1 fibers. Type 1 fibers can sustain tension for long periods, are slow to fatigue, and have high oxidative (aerobic) capacity. Type 2 fibers can rapidly develop high tension, but only for short periods of time, and have high glycolytic (anaerobic) capacity. Types 2a, 2b, and 2c fibers all differ in their relative oxidative capacity.
The evidence that muscle mass decreases with aging is unequivocal. There is less certainty regarding the extent to which this is caused by a loss of muscle fibers versus a decrease in fiber size. There is a loss in the number of muscle fibers with age, but whether some fiber types are lost more rapidly with age remains controversial. Some evidence suggests that type 2 fibers are lost more rapidly than type 1 fibers. Opposing evidence suggests a more rapid reduction in type 2 fiber size with age but not a more rapid reduction in the total number of type 2 fibers. One factor that may contribute to the variation in these findings is the tremendous plasticity of skeletal muscle. Fiber cross-sectional area, and even the relative proportion of fiber types, may reflect the response to existing or recent physical activity patterns. That is, type 2 fiber loss and/or atrophy could be caused by an age-related change in activity patterns. As older adults perform fewer activities requiring rapid development of muscular force, they may have selective reduction in the type 2 fibers that are required for such activities.
Muscle function depends on the coordinated activity of groups of muscle fibers and the motor neuron that innervates them, i.e., the motor unit. The motor neuron appears to control the size, contraction time, resistance to fatigue, and enzyme activity of the muscle fibers it innervates. Hence, neuronal mechanisms could account for many age-related changes in muscle fibers. There is growing evidence that the number of motor units decreases with age. This conclusion is supported by electromyographic studies that suggest a loss of functional motor neurons with age. Indeed, the loss of motor units may even exceed the loss of neurons.
The concept that the quality as well as the quantity of muscle declines with aging has emerged in recent years, although there is no consensus regarding the factors that determine muscle quality or how it should be measured. It has been suggested that muscle quality encompasses many factors (e.g., structural composition, innervation, contractility, capillary density, fatigability, intramuscular fat content, protein metabolism, mitochondrial function, oxidative damage, and glucose metabolism). In general, there is an age-related deterioration in most factors that are thought to influence muscle quality. However, whether these are inevitable consequences of aging seems unlikely, as almost all respond favorably to increased physical activity.
There is some epidemiological evidence that regular physical activity may prevent some of the age-related loss in strength. However, when activities are classified as to their expected effects on muscle strength, epidemiological studies have probably focused on a relatively narrow range of activity near the middle of the continuum. At one end of the continuum is bed rest. Bed rest is associated with a dramatic loss of strength—estimated as high as 1% to 5% per day. At the other end of the continuum is vigorous strengthening exercise (discussed below), which produces substantial gains in strength in older adults.
It is this perspective, integrating findings from studies of bed rest, epidemiology, and experimental interventions, that most clearly suggests that age-related losses in strength are mainly a result of age-related changes in physical activity. As a corollary, epidemiologic studies of adults with similar activity at all ages should show little or no loss in strength. Indeed, a cross-sectional study of workers at a machine shop showed no decline in grip strength between the ages of 20 and 60 years. But aging per se appears to have some role in loss of strength, as, at some point during life, strength will begin to decline despite continued activity patterns.
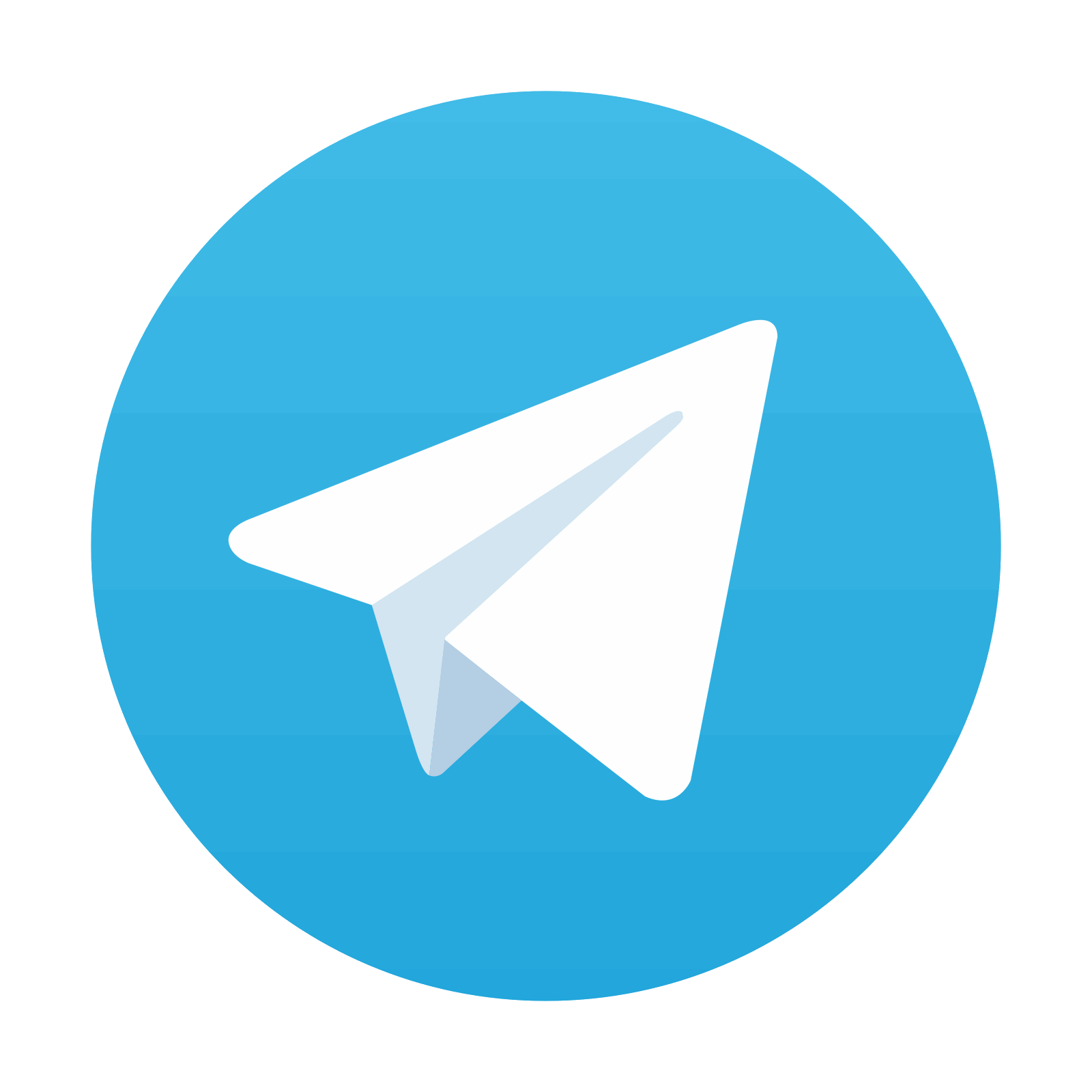
Stay updated, free articles. Join our Telegram channel

Full access? Get Clinical Tree
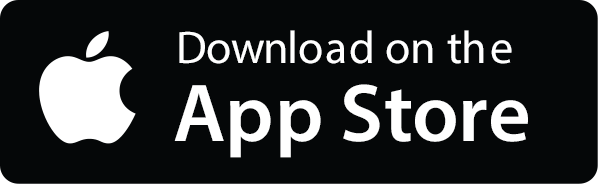
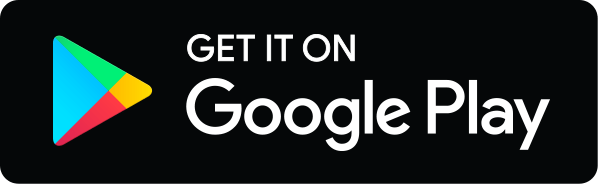