Fig. 12.1
The main components of the Renin-Angiotensin-Aldosterone System (RASS) and its physiological role in the regulatory control of blood pressure, inflammation and cardiovascular function. ACE angiotensin converting enzyme, NEP neutral endopeptidase, AMP aminopeptidase, IRAP insulin-regulated aminopeptidase, SNS sympathetic nervous system, MCP-1 monocyte chemoattractant protein-1, IL-6 interleukin-6, TNF-α tumor necrosis factor alpha, ICAM-1 intercellular adhesion molecule-1, PAI-1 plasminogen activator inhibitor-1
Aldosterone is synthesized mainly by the adrenal gland (glomerular zone), brain and vascular endothelium. The heart expresses steroidogenic acute regulatory proteins and aldosterone synthase. However, evidence suggests that aldosterone in the heart is derived from circulating aldosterone. Aldosterone is basically a steroid hormone produced by the adrenal cortex that contributes significantly to maintaining the body’s balance of plasma sodium and fluid. The main factors responsible for aldosterone release are the plasma levels of potassium and Ang II. Low plasma levels of potassium and Ang II reduce the secretion of aldosterone, as in cases of volume expansion by excess salt intake. Aldosterone acts by binding to epithelial mineralocorticoid receptors in the kidney, essentially resulting in salt and water retention and potassium excretion. Mineralocorticoid receptors are also found in the brain, cardiomyocytes, and muscle cells of arteries. An excess of aldosterone may promote the deterioration of target organs such as the heart, kidney, and vessels—regardless of blood pressure—through perivascular inflammation that progresses to necrosis and finally diffuse fibrosis. These pro-inflammatory and pro-fibrotic effects of aldosterone have been demonstrated experimentally and in clinical studies with a higher prevalence of left ventricle hypertrophy, atrial fibrillation, microalbuminuria, chronic kidney disease, and endothelial dysfunction. The finding of metabolic syndrome is also more frequent in patients with hyperaldosteronism than in patients with essential hypertension [6–8].
Etiology and Prevalence
Primary aldosteronism includes a set of changes with autonomous overproduction of aldosterone that is not dependent on the normal functioning of the RAAS and is suppressed by excess of salt. The most common causes are adrenal adenoma, unilateral or bilateral adrenal hyperplasia, or more rarely, glucocorticoid-remediable hypertension (GRA).
It is believed that 10 % of all hypertensive patients have primary aldosteronism, which is now considered the most common cause of secondary hypertension. It is noteworthy that, among patients with resistant hypertension, 20 % have primary aldosteronism. According to the classification of the Seventh Report of the Joint National Committee on Prevention, Detection, Evaluation, and Treatment of High Blood Pressure (JNC VII), it is estimated that primary aldosteronism is found in 2 % of stage 1 hypertensive patients, 8 % of stage 2, and 13 % of stage 3 [9].
Diagnostic Approach
The diagnosis of primary aldosteronism is very important, because these patients show higher cardiovascular morbidity and mortality than patients of similar age, gender, and degree of blood pressure. Primary aldosteronism should be suspected in patients with stage 2 or 3 of hypertension or resistant hypertension (defined by the failure to reach goal blood pressure in patients adhering to full doses of an appropriate 3-drug regimen that includes diuretics), in hypertensive patients who have hypokalemia or hypokalemia induced by diuretics, in patients who have an adrenal incidentaloma, in hypertensive patients with a family history of early-onset hypertension or with stroke under the age of 40 years, and in all hypertensive patients who have a first degree relative with primary aldosteronism [4, 9–12].
In suspected cases, it is recommended that the clinician use a screening tool to differentiate between primary aldosteronism and secondary hyperaldosteronism due to excessive production of aldosterone that does not depend on hyperactivity of the RAAS. The most common test is the ratio of the plasma aldosterone concentration and plasma renin activity (ARR) [13]. Measurements should be made in the morning in an outpatient setting, with the individuals at least 2 h out of bed, at sitting or standing position for at least 15 min. There should be no limitation on the intake salt, and the physician should be aware of different antihypertensive drugs that can affect the results. In cases where antihypertensive medication is suspended for performing the test, it is recommended a close monitoring of blood pressure. If present, hypokalemia needs to be corrected, because it is a confounding factor in the interpretation of the test. After the plasma potassium is corrected, an interval of 4–6 weeks before performing the ARR should be observed. Care and specific recommendations for the collection and interpretation of the results have been recently compiled [11, 12]. Values that suggest primary aldosteronism are those greater than 30, considering the plasma aldosterone in ng/dl, and plasma renin activity in ng/ml/h. The higher the ARR, the greater the likelihood of primary aldosteronism [13].
After an altered screening test, it is necessary to perform a confirmatory test due to the high rate of false positive results, methodological variability and inappropriate sample collection. Confirmatory tests require the demonstration of no suppression of aldosterone production with expansion of plasma volume and/or blockade of the RAAS using an inhibitor of ACE. One of such tests is the high salt diet for 3–4 days; the diet causes a urinary sodium excretion > 200 mEq/24 h and a decrease in urinary aldosterone excretion to 12–14 μg/24 h. The patient’s blood pressure should be monitored, as the patient undergoes this test in an outpatient setting. Patients with resistant hypertension, heart failure, and chronic kidney disease should not perform this confirmatory exam. Another possibility is an inpatient-based test that involves an overload of salt and water with an infusion of 2 l of normal saline over 4 h and observing whether suppression of plasma aldosterone to 5–10 ng/dl occurs. The combined use of a high salt diet for 3–4 days with the administration of fludrocortisone 0.1 mg orally every 6 h causes an extremely high retention of liquid, and there is a suppression of plasma aldosterone to levels of 5–6 ng/dl. This confirmatory test needs to be done at a hospital, because there is a significant chance of edema and potassium loss. Finally, the use of captopril 25 mg (with measurement of plasma aldosterone 2 h later) can suppress the aldosterone to values lower than 12 ng/dl [4, 9–12].
Patients with a positive confirmatory test should undergo a computed tomography (CT) scan with 2–3 mm slices or a magnetic resonance imaging (MRI) of the adrenal glands. In particular, imaging helps to identify large masses (≥4 cm) that can be excised and those with higher risk of malignancy. It also may useful to visualize the anatomy for an adrenal venous sampling. However, the sensitivity and specificity of CT/MRI are low when using without a confirmatory test in advance, since adrenal imaging might fail to detect many small adenomas, and yet may demonstrate non-functioning nodules in the contralateral gland and apparently unilateral lesions in patients with bilateral adrenal hyperplasia. If only imaging is used, agreement with direct measurement from the adrenal veins only occurs in about 50 % of the cases. Hence, the isolated use of the imaging to define surgical therapeutic approaches leads to inadequate treatment in nearly half of the patients [4].
As a general rule, patients with a screening, confirmatory, and imaging test suggestive of primary aldosteronism should undergo a selective cannulation of the adrenal vein for measurement of aldosterone and cortisol. Nevertheless, this is an invasive procedure that requires a careful patient preparation, a defined protocol, an experienced interventional radiologist, and an accurate data interpretation. Right adrenal vein cannulation is more difficult, due to its smaller caliber and its flow directed to the inferior vena cava rather than the renal vein. The main purpose is to distinguish between unilateral and bilateral disease, detecting a unilateral overproduction of aldosterone that could possibly benefit from adrenalectomy. The relationship should be 3:1, 4:1, or greater for lateralization to be considered positive. The ratio between aldosterone and cortisol can be used to confirm lateralization, and in this case it should be greater than 2:1. In some centers, cosyntropin has been used in bolus or continuously to improve the sensitivity of the method. In reference centers, the sensitivity and specificity of this procedure to detect an unilateral overproduction of aldosterone is 95 and 100 %, respectively [4, 9–12].
In cases of selective cannulation failure of the adrenal vein for measurement of aldosterone and cortisol, the options are to repeat the procedure another time, to treat the patient with mineralocorticoid receptor antagonists or to consider surgical treatment guided by other tests and clinical findings. There are other tests with lower sensitivity and specificity, such as postural stimulation tests, scintigraphy with iodocholesterol, and the dosage of 18-hydroxycorticosterone [11].
Genetic and Molecular Basis
The understanding of the genetic and molecular basis of primary aldosteronism has quickly evolved. Three variants of familial hyperaldosteronism are known today [14]. Early onset of hypertension and severe target organ damage are hallmarks of the inheritable forms. The underlying gene defect has already been identified in familial hyperaldosteronism type I, with ongoing research for types II and III. A highly variable phenotype often precludes the discovery of the familial appearance of these syndromes, making the family history extremely important to discover some Mendelian pattern of inheritance. The identification of affected families is highly rewarding because all variants can potentially be cured or at least specifically treated. Testing the relatives of an index patient may sometimes allow the start of a preemptive treatment.
Familial hyperaldosteronism type I, also known as GRA, is an autosomal dominant disorder caused by a chimeric gene duplication formed from unequal crossover between the promoter sequence of CYP11B1 gene, that encodes the 11ß-hydroxylase, and the coding sequence of CYP11B2 gene, that encodes aldosterone synthase. This genetic abnormality results in an ectopic expression of aldosterone synthase in the zona fasciculate, with regulation of the mineralocorticoid production exerted by ACTH instead of by the normal secretagogue, Ang II. Familial hyperaldosteronism type II is an autosomal dominant disorder and may be monogenic. This type of hyperaldosteronism is not ACTH-dependent and therefore is not suppressible by dexamethasone. GRA mutation testing is negative. Linkage analyses studies have shown an association with chromosome 7p22. Familial hyperaldosteronism type III is characterized by severe hypertension in early childhood associated with marked aldosteronism, hypokalemia, significant target organ damage and poor response to full doses of several classes of antihypertensive drugs, including spironolactone and amiloride. This finding distinguishes familial hyperaldosteronism type III from the other familial forms and sporadic primary aldosteronism, because spironolactone is usually successful in controlling blood pressure in these familial forms, while type III patients do not respond and require bilateral adrenalectomy. Important advances made in the past year have included identification of KCNJ5 potassium channel mutations in the pathogenesis of both aldosterone-producing adenomas and familial hyperaldosteronism type III [14–16].
Current and Emerging Treatment
The therapeutic goals involve not only normalization of blood pressure and plasma potassium levels, but also reduction of the increased cardiovascular morbidity and mortality associated with excessive aldosterone secretion. The identification of the cause helps to implement the most appropriate treatment. Table 12.1 summarizes the first-line and second-line therapeutic options for the subtypes of primary aldosteronism, which are discussed in more details below.
Table 12.1
Current and emerging treatment strategies for primary aldosteronism
Subtype | First-line treatment | Second-line treatment |
---|---|---|
Unilateral | Unilateral laparoscopic adrenalectomy | Spironolactone Eplerenone Aldosterone synthase inhibitors |
Bilateral | Spironolactone Eplerenone Aldosterone synthase inhibitors | |
Glucocorticoid-remediable aldosteronism | Low-dose glucocorticoids (hydrocortisone or prednisone) | Spironolactone Eplerenone (preferable in children) Aldosterone synthase inhibitors |
Surgical Treatment
The recommended treatment in patients with unilateral adrenal adenoma or unilateral hyperplasia is unilateral laparoscopic adrenalectomy [11]. If the patient refuses surgery or there are contraindications for the procedure, aldosterone antagonists should be initiated. Total reversion of hypertension, with no need for any antihypertensive drug, occurs in 50 % of patients treated with surgery, whereas normalization of plasma potassium levels is observed in virtually all patients. Normalization of blood pressure is usually achieved 1–6 months after the surgical procedure. Chances of cure increase in patients with no other hypertensive family members and using one or two classes of antihypertensive medications. The laparoscopic technique is considered the best choice due to reduced pain, shorter hospitalization, and lower costs. Preoperative surgical care should be directed to maintain adequate blood pressure and potassium levels.
Medical Treatment
Bilateral hyperplasia and GRA should be treated medically. This therapy is also employed for all patients who decline or are not considered for surgery. Mineralocorticoid receptor antagonists that reduce blood pressure and promote cardiovascular protection from the deleterious effects of aldosterone are the drugs of choice to treat primary aldosteronism [4–7, 10–12, 17].
Spironolactone has been used for more than 4 decades. Treatment can be started with doses of 12.5–25 mg in a single daily administration, which can be progressively increased up to 400 mg/day to achieve a high-normal serum potassium concentration, without adding oral potassium supplementation. Maintenance doses usually range between 25 and 100 mg/day. Plasma potassium should be monitored, and special care should be taken in elderly patients with diabetes or renal dysfunction. Concomitant use of salicylates can decrease the effectiveness of spironolactone. The main adverse effects are dose dependent and include erectile dysfunction, pain, and increased sensitivity of the breasts in men and women, and changes in the menstrual cycle of premenopausal women. Small dosages of thiazide diuretics help prevent an excessive increase in potassium and better control blood pressure.
Eplerenone is a selective antagonist of the mineralocorticoid receptors with minimal effect on the sex steroid receptors. In comparison with spironolactone, it is better tolerated, more expensive and must be started in a dose of 25 mg twice daily, titrated upward to 100 mg/day. It is an alternative for patients presenting with adverse events during spironolactone therapy, as clinical trials comparing both drugs have shown similar antihypertensive effects [4, 18]. Side effects include dizziness, headache, fatigue, diarrhea, hypertriglyceridemia, and elevated liver enzymes.
Other drugs that can be used include antagonists of the sodium channels in renal epithelium, such as amiloride and triamterene. They do not have an antihypertensive efficacy similar to spironolactone, but are potassium sparing diuretics and have no sexual side effects. Finally, calcium channel antagonists and blockers of ACE 1 and 2 might be employed in some situations [4, 11].
Aldosterone synthase inhibitors are emerging as promising treatment agents acting as aldosterone antagonists—inhibiting its formation and preventing the reactive increases in aldosterone levels and their mineralocorticoid receptors-independent effects [19]. Several aldosterone synthase (CYP11B2) inhibitors are being developed. Fadrozole, an aromatase inhibitor or its dextroenantiomer (FAD286), has been shown to inhibit aldosterone synthase and to reduce mortality, cardiac hypertrophy, albuminuria, cell infiltration, and matrix deposition in the kidney in double transgenic renin rats (dTGR), yet without a profound effect on blood pressure [20]. Another agent, LCI699, was shown to reduce 24 h-ambulatory systolic blood pressure and effectively suppress supine plasma aldosterone concentrations in patients with primary aldosteronism [21, 22]. Although plasma cortisol concentrations did not change, the ACTH concentrations were elevated, the plasma cortisol response to ACTH stimulation was blunted, and the plasma potassium concentration was increased.
The main clinical goal for aldosterone-synthase inhibitors is to be as good as the mineralocorticoid receptor antagonists for blood pressure reduction, with better tolerability. LC1699 was only modestly effective in patients with primary aldosteronism, with the demonstration that 1 mg of LCI699 was not superior to 50 mg of eplerenone in blood pressure reduction in patients with stage 1 and 2 hypertension. As a consequence, the development of LCI699 was stopped in 2010 in favor of seeking more specific inhibitors. Future studies are needed to address the dose–response relationship, clinical implications and the potential blood pressure-independent organ-specific effects of these new agents [21, 22].
For patients with confirmed diagnosis of GRA, chronic use of physiologic doses of glucocorticoid is the first-line therapy. Care should be taken to not produce Cushing’s syndrome by excessive doses of glucocorticoids, especially when dexamethasone is employed in children. Preferable drugs are hydrocortisone (10–12 mg/m2 per day) or prednisone in equivalent doses, which ideally should be taken at bedtime to suppress the early morning ACTH surge. Treatment with mineralocorticoid receptor antagonists can be as effective as glucocorticoids, with the potential advantages of avoiding the iatrogenic side effects [11].
Hyperdeoxycorticosteronism
This is a distinct form of mineralocorticoid excess characterized by hypertension, hypokalemia, low aldosterone, low renin and excessive production of 11-deoxycorticosterone (DOC). Medical disorders associated with hyperdeoxycorticosteronism include two forms of congenital adrenal hyperplasia (11β-hydroxylase and 17α-hydroxylase deficiency), DOC-producing tumors, primary cortisol resistance, and genetic or acquired forms of apparent mineralocorticoid excess (AME) [23].
11β-hydroxylase deficiency accounts for about 5 % of all cases of congenital adrenal hyperplasia, with more than 40 mutations described in CYP11B1 gene. Diagnosis is made by the demonstration of high plasma levels of DOC and 11-deoxycortisol. There is also an excessive secretion of adrenal androgens that causes acne, hirsutism and virilization in girls. Boys may present with pseudoprecocious puberty. 17α-hydroxylase deficiency is a rare cause of congenital adrenal hyperplasia whose diagnosis is usually suspected at time of puberty, due to primary amenorrhea in genetic females, or pseudohermaphroditism or female phenotype in genetic 46,XY males. The main laboratorial findings to confirm the diagnosis are low levels of plasma adrenal androgens, 17α-hydroxyprogesterone, aldosterone, ARR, and cortisol, associated with high levels of DOC, corticosterone, and 18-hydroxycorticosterone. Glucocorticoid replacement is the treatment of choices for both disorders [23].
DOC-producing tumors are usually large and malignant, and they can also secrete androgens and estrogens causing virilization in women and feminization in men. Surgical resection of the tumor is the recommended therapy. Primary cortisol resistance is a rare familial syndrome caused by defects in the glucocorticoid receptors and the steroid-receptor complex, characterized by alkalosis, elevated serum levels of cortisol, adrenal androgens, and DOC. The signs and symptoms of Cushing’s syndrome are typically absent. AME is associated with reduced activity of HSD11B2 enzyme, which normally inactivates cortisol in the kidney. It can be hereditary or secondary to the use of licorice or some chewing tobaccos. Patients with AME present metabolic alkalosis, normal cortisol levels, and an altered ratio of cortisol to cortisone in a 24-h urine collection, up to tenfold above the normal values. Mineralocorticoid receptor antagonists or dexamethasone are used in the treatment of AME and primary cortisol resistance [23].
Pheochromocytoma and Paragangliomas
Pheochromocytoma is a catecholamine-producing tumor derived from chromaffin cells. When such tumors arise outside of the adrenal gland, they are termed extra-adrenal pheochromocytomas or paragangliomas. The term paraganglioma is sometimes also used for tumors originated from parasympathetic tissue in the head and neck, most of which do not produce catecholamines. The word pheochromocytoma (in Greek, phios means dusky, chroma means color, and cytoma means tumor) refers to the color the tumor cells acquire when stained with chromium salts [24].
The clinical presentation of a pheochromocytoma varies from an adrenal incidentaloma to a picture of severe hypertension and life-threatening arrhythmias due to intense, albeit frequently episodic, catecholamine secretion. Fortunately, these tumors are potentially curable. Therefore, early detection is crucial, making a systematic and effective diagnostic approach clearly warranted [25].
Location of the Tumors, Biosynthesis and Metabolism of Catecholamines
Pheochromocytomas are usually derived from the adrenal medulla, but may develop from chromaffin cells in or near sympathetic ganglia. Over 90 % of pheochromocytomas are located within the adrenal glands, and 98 % are within the abdomen. Extra-adrenal locations include the organ of Zuckerkandl (close to origin of the inferior mesenteric artery), bladder wall, heart, mediastinum, and carotid and glomus jugulare bodies [24]. The adrenal medulla, part of the sympathetic nervous system, consists of certain cells known as chromaffin cells, characterized by excessive production of catecholamines.
The clinical manifestations of a pheochromocytoma result from excessive catecholamine secretion by the tumor. The rate-limiting step in catecholamine biosynthesis involves conversion of tyrosine to 3,4-dihydroxyphenylalanine (L-dopa) by the enzyme tyrosine hydroxylase [26]. This enzyme is largely confined to dopaminergic and noradrenergic neurons of the central nervous system, and to sympathetic nerves and adrenal and extra-adrenal chromaffin cells in the periphery. Other sites of catecholamine synthesis include certain non-neuronal cells of the gastrointestinal tract and kidneys [27]. After that, L-dopa is converted to dopamine in the cytosol of the cell and transported into the cell’s chromaffin granules, where it is either stored or converted to norepinephrine by the enzyme dopamine β-hydroxylase. Some of the norepinephrine migrates back into the cytosol, where it is converted into epinephrine by the enzyme phenylethanolamine N-methyltransferase (PNMT). Then, the epinephrine returns to the chromaffin granules where it is stored. In the adult adrenal medulla, epinephrine (80–85 %) is the predominant catecholamine stored and secreted [28].
The catecholamines are inactivated by two enzymes, catechol-Omethyl-transferase (COMT) and monoamine oxidase (MAO), in the liver. Norepinephrine and epinephrine are metabolized by COMT to normetanephrine and metanephrine, respectively. In a next step, normetanephrine and metanephrine are metabolized to vanillylmandelic acid by MAO plus aldehyde dehydrogenase [28]. Unaltered epinephrine and norepinephrine and their inactive metabolites are finally excreted into the urine.
Most pheochromocytomas produce predominantly norepinephrine, many produce both norepinephrine and epinephrine, and a minority produce predominantly epinephrine [4]. Dopamine, which is usually efficiently converted to norepinephrine, is a minor component. However, some cases of paragangliomas have been identified that produce mainly dopamine [27, 29]. Pheochromocytomas also store and secrete in the blood many peptides like endothelin, erythropoetin, parathyroid hormone-related peptide, neuropeptide-Y, and chromogranin-A. Differently from the adrenal medulla, pheochromocytomas are not innervated, and catecholamine discharge is not precipitated by neural stimulation. The trigger for catecholamine release is unclear, but multiple mechanisms have been postulated, including direct pressure, medications, and changes in tumor blood flow [24].
Pathology and Molecular Genetics
The essential histopathologic characteristics are very similar among pheochromocytomas. Sporadic pheochromocytomas are generally solitary, well-circumscribed, well-vascularized, encapsulated tumors, with characteristic histopathological features [30]. Approximately 10 % of pheochromocytomas are malignant. Histologic criteria of cellular atypia, numerous mitoses, and invasion of adjacent tissues or vessels do not identify, with certainty, which tumors have the potential to metastasize. Actually, the 2004 World Health Organization criteria define malignancy in pheochromocytoma by the presence of metastases and not by local invasion [27, 30]. Common metastatic sites include bone, liver, and lymph nodes.
Improvements in genetics, diagnosis, and treatment of pheochromocytomas have altered the approaches to these tumors in recent years. The formerly used rule of “10 % tumor” for pheochromocytoma (10 % are bilateral, 10 % are found in children, 10 % are inherited, 10 % are malignant, and 10 % are found outside the adrenal gland) has been challenged [4, 31]. According to the latest studies, up to 24 % of tumors among patients with nonsyndromic pheochromocytoma may be hereditary. As shown in Table 12.2, there are associations with multiple endocrine neoplasia type 2 (MEN-2A or MEN-2B), neurofibromatosis type 1 (NF-1), von Hippel-Lindau (VHL) syndrome, and with germ-line mutations of genes encoding succinate dehydrogenase subunits B, C, and D (SDHB, SDHC, SDHD) [4, 32]. This novel genetic knowledge has led to routine screening in patients with identified mutations, even in the absence of typical clinical signs and symptoms of pheochromocytoma. Because hereditary tumors generally occur at a younger age than sporadic tumors, age at presentation is an important factor to consider when deciding to test for disease-causing genes. Besides patient’s age, the biochemical profile of catecholamine secretion, localization of the primary tumor, and previous family history must be carefully evaluated to choose a proper genetic test. For instance, MEN-2- and NF-1-related pheochromocytoma always secrete epinephrine, while those related to VHL secrete norepinephrine, and some related to SDHB cause elevation of dopamine together with norepinephrine. MEN-2, VHL, and NF-1 tumors are almost always found in the adrenal gland, whereas SDHB-related tumors are found in extraadrenal localizations. In those patients with malignant disease secondary to an extra-adrenal paraganglioma, around half of them harbor SDHB mutations [4].
Table 12.2
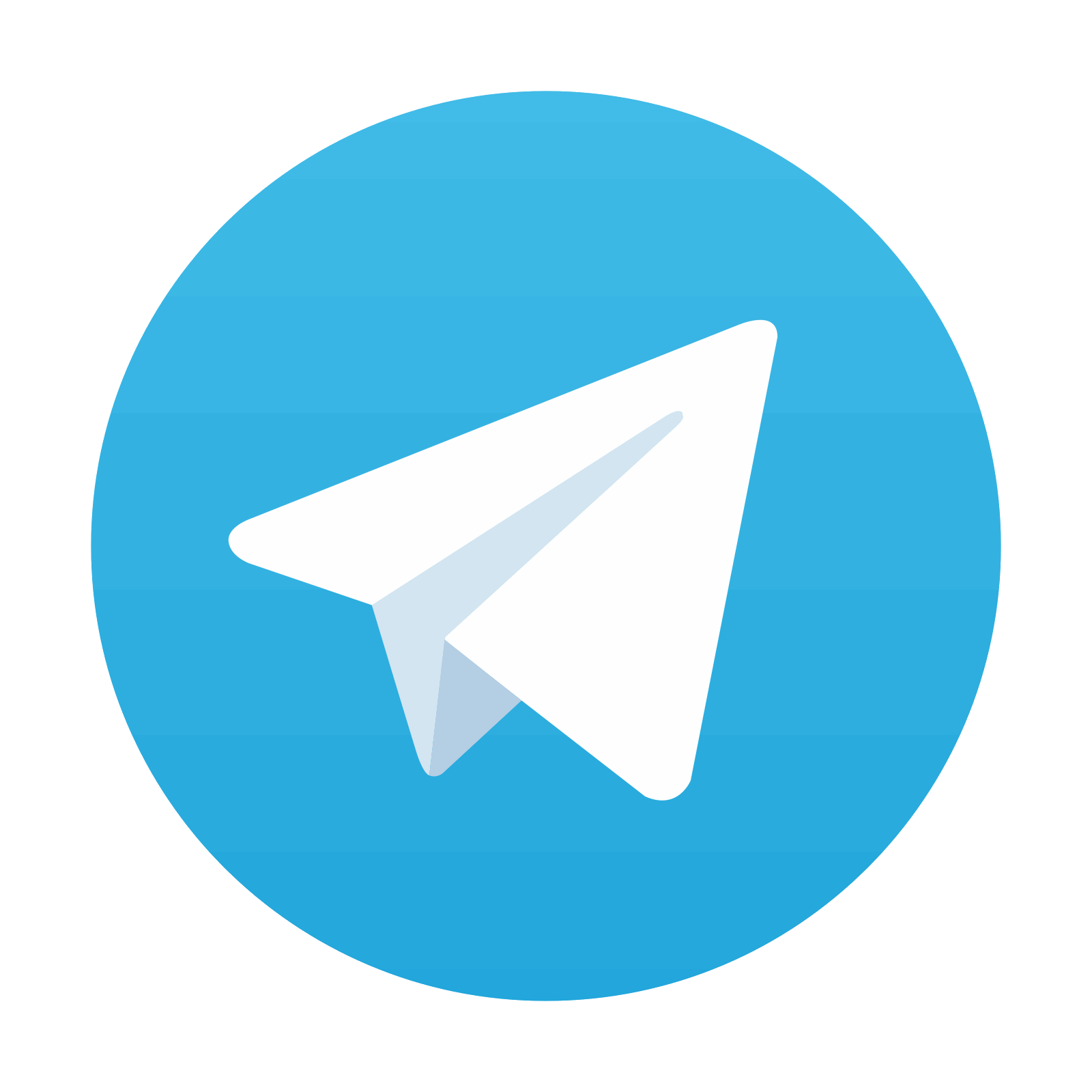
Hereditary disorders associated with pheochromocytomas/paragangliomas
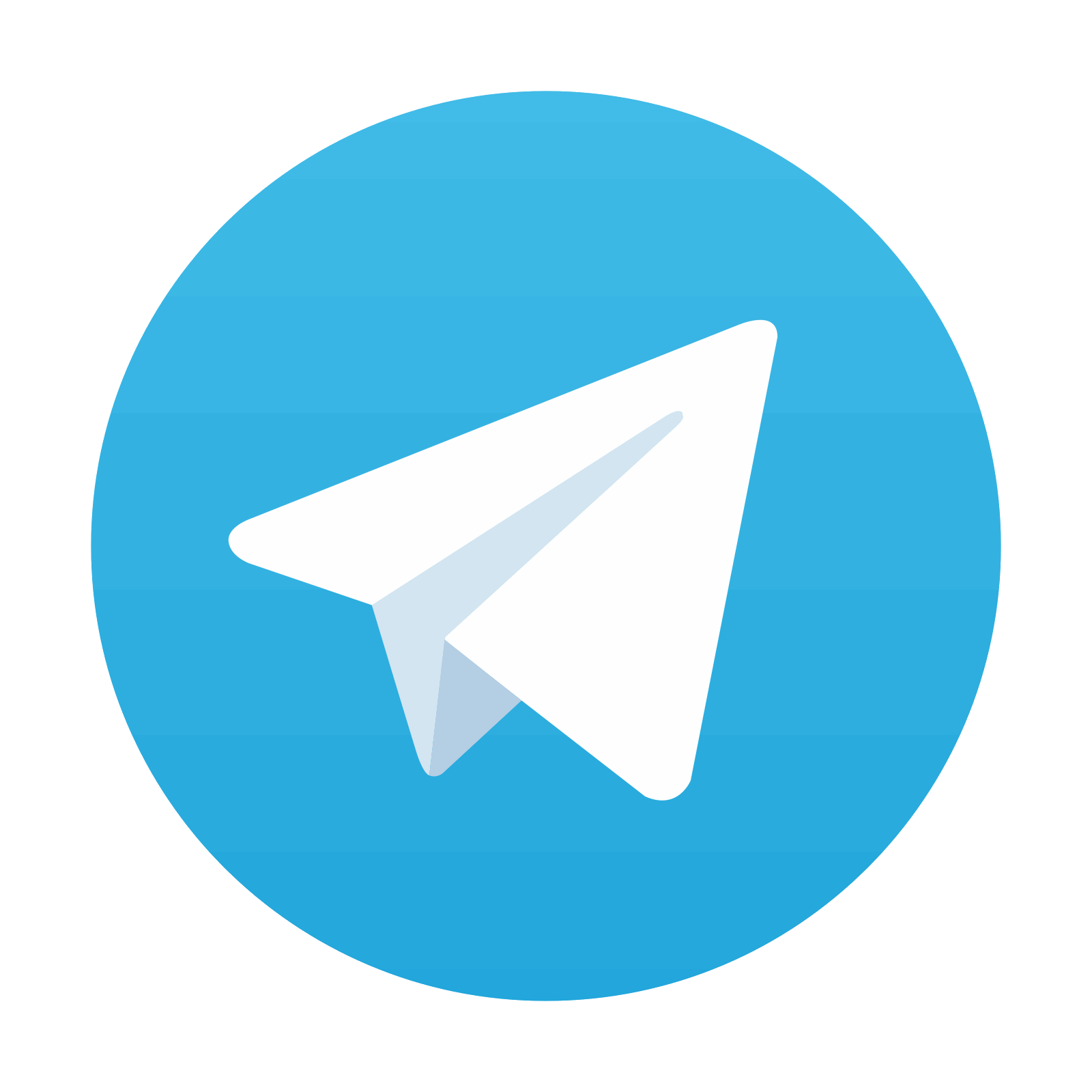
Stay updated, free articles. Join our Telegram channel

Full access? Get Clinical Tree
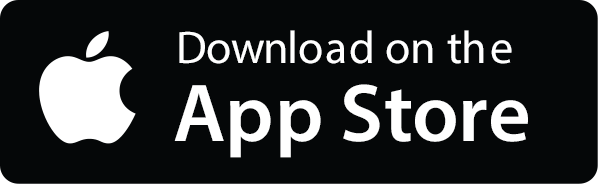
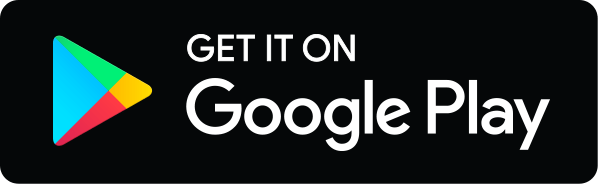
