Definition
Dyslipoproteinemia, also referred to as dyslipidemia, encompasses a range of disorders of lipoprotein lipid metabolism that include both abnormally high and low lipoprotein concentrations, as well as abnormalities in the composition of these lipoprotein particles. Dyslipoproteinemias are clinically important because of their role in the pathogenesis of cardiovascular disease (CVD), which includes coronary artery disease (CAD), cerebrovascular disease, peripheral vascular disease, and renal disease. Thus the term dyslipoproteinemia is broader than the term hyperlipidemia, which focuses solely on the concentrations of the lipoproteins.
There is considerable evidence that dyslipoproteinemia is a risk factor for CVD in adults aged 60 to 80 years, with an expanding body of literature demonstrating dyslipoproteinemia as a risk factor for people older than 80 years of age. The consensus guidelines for the management of dyslipidemia are continually being reevaluated. Over the past several years, National Cholesterol Education Program (NCEP) Adult Treatment Panel (ATP) consensus guidelines have made the target lipoprotein concentrations more stringent for individuals with CVD. These guidelines have in turn led to more stringent recommendations for patients with CVD equivalents such as diabetes mellitus and renal disease, and for individuals with multiple CVD risk factors who are at increased risk for CVD events. Epidemiologic and clinical trial data suggest that the optimal concentration for low-density lipoprotein cholesterol (LDL-C) maybe <100 mg/dL, or even as low as 70 mg/dL for some high-risk patients. Similarly, the optimal concentration for high-density lipoprotein cholesterol (HDL-C) maybe >60 mg/dL. As discussed below, triglyceride, cholesterol, and LDL-C levels tend to rise with increasing age, before falling late in the sixth decade. HDL-C decreases at puberty and then is relatively constant across the age-span until the age of 70 years when it may increase (Figure 110-1). As a result, a majority of older individuals could be classified as having undesirable lipoprotein lipid concentrations and are candidates for lifestyle intervention and potentially for pharmacological therapy if their concentrations exceed treatment cut points. The changes in the treatment guidelines are reflected in secular changes in prescribing patterns of drugs to treat hyperlipidemia, as the number of older adults on cholesterol-lowering medications has quadrupled over the past 15 years. In 2002, more than 30% of Medicare patients between the ages of 65 and 84 years were prescribed a statin for cholesterol reduction.
Figure 110-1.
Time-series analyses of lipid concentrations in U.S. adults aged 20–75+ yr. Data are from the NHANES surveys of 1976–1980, 1988–1994, and 1999–2002 (http://www.cdc.gov/nchs/nhanes.htm). NHANES data are a representative sample of the civilian, noninstitutionalized population. Age groups are indicated on the abscissa, lipid concentrations in mg/dL on the ordinate. Each age group contains mean lipid concentrations obtained at three time points. Over 26 yr of follow-up, LDL cholesterol concentrations have dropped in men and women. There is more variability in the patterns of change for HDL (data not shown) and triglycerides; however overall over the last 26 yr, triglyceride concentrations have generally increased. Data represent values for all races combined. Concentrations are expressed as arithmetic means for LDL cholesterol and geometric means for triglycerides. (Data adapted from Carroll MD, Lacher DA, Sorlie PD, et al. Trends in serum lipids and lipoproteins of adults, 1960–2002. JAMA. 2005;294:1773–1781.)
Many older individuals have metabolic abnormalities that promote atherosclerosis, but are not routinely measured. These risk factors include elevated lipoprotein (a) [Lp (a)], elevated apolipoprotein (apo) B, small dense LDL particles (the atherogenic LDL pattern B phenotype), oxidized LDL, apo E4 genotype, insulin, high-sensitivity C-reactive protein (hsCRP), homocysteine, and other markers of inflammation. These biomarkers provide incremental benefit in predicting CVD risk beyond the routine measures that are included in the Framingham Risk score. The role of genetic analyses in the assessment of CVD risk is an evolving field of research. It is also becoming increasing clear that the measurement of structural markers of arterial vulnerability (carotid intimal-medial thickness, assessment of coronary artery calcium among others) and functional markers of arterial vulnerability (brachial artery reactivity assessment of endothelial function, arterial stiffness, and ankle-brachial blood pressure index [ABI]), similarly provide incremental benefit in predicting CVD risk in elderly people and help identify older individuals who might benefit from risk factor intervention.
Given the high prevalence of physical inactivity, hypertension and type 2 diabetes (T2DM) in the elderly, the geriatrician must have a firm understanding of the pathogenesis and treatment of disorders of lipoprotein metabolism in the geriatric population. This chapter reviews the epidemiology and mechanisms underlying age-associated changes in lipoprotein lipid concentrations, evidence that hyperlipidemia is a risk factor for coronary heart disease (CHD) in the elderly population, and controversies regarding the screening and treatment of hyperlipidemia in elderly patients.
Pathophysiology
Triglycerides (TG) and cholesterol of exogenous (dietary) and endogenous origin are transported in the blood stream as part of lipoprotein particles. These lipoprotein particles contain TG, cholesterol, cholesterol esters, phospholipids, and apolipoproteins (apo). Historically, the lipoprotein particles in the plasma have been classified on the basis of their density or by their electrophoretic mobility. Using ultracentrifugation, five major classes of plasma lipoproteins can be identified in ascending order of density: chylomicrons, very low-density lipoproteins (VLDL), intermediate density lipoproteins (IDL), low-density lipoproteins (LDL), and high-density lipoproteins (HDL). The major classes of lipoproteins comprise subpopulations of lipoproteins that differ in composition, metabolic function, and atherogenic potential.
Lipoprotein metabolism involves the absorption of exogenous dietary fat from the GI tract, and the endogenous synthesis and secretion of triglyceride-rich lipoproteins by the liver. The physiological regulation of this process provides an understanding of the pathogenesis of atherosclerosis as the metabolic mechanisms by which therapies can target key regulatory sites to reduce CHD risk. In summarizing this process, we highlight a few key regulatory sites of normal and abnormal metabolic regulation and their consequences (Figure 110-2).
Figure 110-2.
Schematic overview of the Apo B containing lipoproteins. Lipids in the diet are hydrolyzed in the small intestine, and the resultant fatty acids and monoglycerides are repackaged with apo B-48 into TG-enriched chylomicron particles by the intestinal enterocytes and secreted into the lymphatics (1). Dietary chylomicrons bypass the liver and enter the plasma via the thoracic duct. They acquire apo E and C-II from HDL, and are hydrolyzed by lipoprotein lipase (LPL) to smaller TG-depleted, cholesterol-rich chylomicron remnants (2). These remnants are taken up by the liver primarily by the LDL receptor-related protein receptor (LDL-R) and degraded, affecting endogenous TG synthesis and secretion (3). Endogenous TG is synthesized in the liver from carbohydrate and free fatty acid (FFA, derived from adipocyte hydrolysis by hormone-sensitive lipase) precursors assembled in the Golgi with apo B-100, E, C-II, C-III, and secreted as triglyceride-rich very low-density lipoproteins (VLDL-TG) (4). VLDL-TG are hydrolyzed by LPL to VLDL remnants and IDL, which are taken up by scavenger cells or apo E-specific hepatic receptors and degraded in the liver. VLDL remnants are removed irreversibly or hydrolyzed further by LPL and hepatic lipase to cholesterol-rich LDL particles (5). LDL has its cholesterol removed from plasma by the LDL receptor or oxidized and taken up by CD36 scavenger receptors on macrophages to generate an intracellular cholesterol pool. The hydrolysis of TG from VLDL-TG by LPL also generates free cholesterol, and phospholipids (PL), substrates for lecithin cholesterol acyl transferase (LCAT). Nascent HDL interacts with the ATP-binding cassette transporter-1 (ABCA1) on peripheral lymphoctes to remove excess cholesterol from cells and then binds with apo A-I, the principal activator of LCAT, to esterify cholesterol forming large, spherical cholesterol-ester-rich HDL2 particles (6). [Thus apo A-I and the ABCA1 transporter play critical roles in the formation of HDL and reverse cholesterol transport.] Cholesterol is transported back to the liver directly by HDL or via transfer to apo CII containing VLDL or IDL by the cholesterol ester transfer protein (CETP). Hepatic lipase stimulates HDL-C uptake by the hepatic scavenger class B type 1 receptor (SR-B1) (7). [Cholesterol is also synthesized endogenously from acetate precursors, regulated by the enzyme HMG-CoA reductase. The accumulation of intracellular free cholesterol suppresses endogenous cholesterol synthesis by inhibiting HMG-CoA reductase, the rate-limiting enzyme in cholesterol synthesis, stimulates cholesterol reesterification by acyl-CoA: cholesterol transferase, and downregulates LDL receptor synthesis (8).] However, if there is excess VLDL-TG production or dietary fat intake, CETP exchanges cholesterol from HDL with TG from VLDL, raising the TG and lowering the cholesterol composition of HDL (9). Hepatic lipase hydrolyzes HDL TG and phospholipid, converting HDL2 back to smaller HDL3 particles, reducing the efficient clearance of cholesterol from cells. Cholesterol can then be resecreted from the liver on VLDL, increasing endogenous LDL synthesis, excreted directly into the bile, or converted to bile acids for reabsorption (enterohepatic circulation) or excretion in stool and bile (10).
The major inherited forms of dyslipoproteinemia, and strategies for drug and dietary treatments for hyperlipidemia should be directed at these key regulatory sites of lipoprotein metabolism. Increased knowledge of the metabolic regulation of the lipoprotein subclasses by enzymes, cofactors, and transfer proteins, and various receptors has provided a framework for the understanding of pathogenesis of disorders of lipoprotein metabolism and their treatment. These disorders arise either from accelerated synthesis, decreased degradation of the lipoproteins, or both. The primary dyslipoproteinemias can be caused by biochemical defects resulting from single-gene mutations or can be caused by polygenetic or multifactorial causes. Secondary dyslipidemias, caused by systemic disorders such as obesity, diabetes, hypothyroidism, renal and liver disease, are more common in the elderly population.
Age, environmental factors, diet, and other lifestyle factors affect the phenotypic expression of both the single-gene and polygenetic disorders. The prevailing dogma is that dyslipidemias that arise from the single-gene mutations have both an earlier age of onset and clinical manifestations of atherosclerosis at a younger age than the polygenetic disorders. For example, individuals with familial hypercholesterolemia, both the homozygous and heterozygote genotypes, can be identified in childhood. Familial combined hyperlipidemia might not be phenotypically manifest until after puberty, and is usually clinically and biochemically diagnosed in the fourth decade. Familial hypertriglyceridemia and other disorders associated with increased TG production and decreased clearance may not become clinically apparent until middle age when age-associated increases in adiposity, physical inactivity, and the development of insulin resistance enhance the expression of the metabolic defects. Most older individuals presenting to the geriatrician with hypercholesterolemia have multifactorial disorders. Age-associated increases in adiposity, decreased fitness, decreased number or function of the LDL receptor, and hormonal changes associated with menopause act in concert with polygenetic factors to raise LDL-C concentrations in older age. Similarly, the interaction of genetic, lifestyle, and environmental factors increase the age-associated prevalence of atherogenic LDL pattern B phenotype. Early detection and treatment of hyperlipidemia is critical for primary and secondary prevention of CHD in the elderly.
A number of mechanisms have been proposed to account for the age-related changes in lipoprotein concentrations, particularly change in LDL-C concentration. These mechanisms include age-related increases in dietary fat content and adiposity, declines in physical activity and physical fitness, and decreases in the number and function of the hepatic LDL receptor.
Age-associated changes in dietary content are reviewed in Chapter 38. In men in the Baltimore Longitudinal Study of Aging (BLSA), total caloric intake, cholesterol intake, the percent of calories obtained from fat, and the percent of calories obtained from saturated fat declined with age. Such age-associated changes in dietary content would be expected to result in lower levels of total and LDL-C, a result that is opposite to the commonly observed age-associated increase in these parameters. Others have reported changes in the distribution of energy consumed in older adults with a larger relative contribution of calories consumed at breakfast and snacks, with fewer calories consumed at lunch and dinner. The increased snacking may result in a greater portion of calories from sweets and carbohydrates. It is well recognized that low total energy consumption is associated with inactivity, deconditioning, sarcopenia, and frailty.
The prevalence of obesity increases with aging, with a preferential accumulation of fat in visceral abdominal sites. These changes are associated with hyperinsulinemia, which predisposes older adults to develop glucose intolerance, type 2 diabetes, and other metabolic risk factors for CHD. The Italian Longitudinal Study on Aging showed higher insulin concentration was associated with low HDL-C and apo AI concentrations, higher TG and glucose concentrations, hypertension, and higher body mass index (BMI), but not high total or LDL-C in a cohort of 5632 people aged 65 to 84 years. Among the subjects with diabetes, TG concentration was higher but HDL-C concentration did not differ across insulin quartiles; however, total and LDL-C concentrations were lower and white blood cell count higher in women with diabetes with high insulin concentrations.
The prevalence of metabolic syndrome increases with age. There is an ongoing controversy as to the utility of the metabolic syndrome as a disease construct (also see the “Special Issues” section at the end of the chapter). A joint statement in 2005 from the American Diabetes Association and the European Association for the Study of Diabetes noted that the metabolic syndrome is imprecisely defined, there is a lack of consensus on the underlying pathophysiology, and there is little evidence that the metabolic syndrome denotes greater CVD risk per se than the sum of its parts (low HDL-C, hypertension, glucose intolerance, hypertriglyceridemia, increased waist circumference). Longitudinal follow-up and the assessment of mortality will determine the relationship of hyperinsulinemia and the metabolic syndrome to CHD risk.
Regular physical activity has a significant impact on plasma lipoprotein concentrations. Older athletes have higher HDL-C, lower TG, and LDL-C concentrations than age-matched sedentary counterparts, and the concentrations are often similar to those seen in younger adults. The interpretation of these cross-sectional comparisons is confounded by differences in body composition, as the trained subjects are significantly leaner than the sedentary population. The differences in obesity contribute the most to the difference in TG and HDL-C concentrations between older athletes and their sedentary counterparts, while the differences in maximal aerobic capacity (VO2 max) account for a small percentage of the variance in these lipoproteins. Current physical activity guidelines from the Centers for Disease Control and American College of Sports Medicine recommend that older adults participate in moderate-intensity aerobic activities 3 to 5 days a week for at least 30 minutes each session, daily stretching for flexibility, and strength-building activities 2 to 3 days per week (see Chapter 114). These guidelines are based on experimental and epidemiologic data that energy expenditure and metabolic responses to exercise training reduce lipoprotein lipid, insulin-glucose, and blood pressure associated CVD risk, and directly affect CVD mortality. Vigorous exercise improves cardiovascular fitness indexed as VO2 max, reduces visceral adiposity, and has salutary effects on lipid profiles, insulin sensitivity, glucose metabolism, and blood pressure, thereby reducing major components and risk associated with the metabolic syndrome.
A number of studies have examined the metabolism of LDL in young and older subjects. Miller pooled data on the fractional catabolic rate of LDL apo B from four groups of subjects; normal men, normal women, individuals with heterozygous familial hypercholesterolemia, and in individuals with hypercholesterolemia. In these four groups the LDL apo B fractional catabolic rate decreased with age, whereas the LDL production rate did not change with age. The age-related rise in LDL was attributed to an acquired defect in the LDL receptor function. Similar findings subsequently were reported by Ericson, who suggested that there might be an age-associated reduction in hepatic LDL receptor expression. Kinetic studies with isotopes suggest that the age-associated increase in VLDL apo B-100 is caused by an increased rate of production, whereas the age-associated increase in LDL apo-B 100 is caused by increased residency time. Matthan and colleagues determined that decreased clearance of TG-rich lipoprotein and of LDL contributed to the increase in TG and LDL in postmenopausal women, rather than increased production of these particles. These studies did not control for differences in body composition and physical activity among age groups, factors which may elevate plasma-free fatty acid concentrations, glucose, and insulin concentrations in the older subjects and result in a raise in hepatic production of TG. We would contend that many of the purported age-related changes in lipoprotein concentrations reflect interactions between primary age-related changes in LDL metabolism and the effects of obesity, physical inactivity, diet, and other lifestyle habits, or secondary aging.
Epidemiology
CHD remains the leading cause of death in older men and women. Despite secular declines in mortality rates from CHD, since 1950, as a result of the aging of the population since 1950, the number of CHD deaths in the United States has actually increased in people 65 to 74 years of age, and has more than doubled in those older than age 75 years. It is estimated that almost 16 million adults in the United States have prevalent CHD and 5.6 million have had a stroke. Based on National Health and Nutrition Examination Survey (NHANES) data, 23% of men and 15% of women aged 60 to 79 years have prevalent CHD. For those aged 80 years and older, 33% of men and 22% of women have prevalent CHD. However, these numbers severely understate the burden of CHD in the older adult population because much of the disease is clinically silent. Electron beam tomography, a noninvasive technique to detect subclinical coronary artery calcification, as indicator of atherosclerotic plaque burden and CHD, showed that two-thirds of the people older than 65 years of age in the Cardiovascular Health Study had either subclinical atherosclerotic disease or clinically apparent CHD. According to American Heart Association statistics, in 2004, 83% of the deaths from CHD and 88% of deaths from stroke occur in people older than 65 years of age. Furthermore, the projected growth of the population older than 80 years of age by 2030 suggests there will be a huge increase in the burden of disease from CHD and CVD. Many of these deaths and some of this burden maybe preventable (see “Prevention” later in the chapter).
Many cross-sectional studies have examined age-associated changes in lipoprotein concentrations in both men and women. Unfortunately cross-sectional studies alone cannot distinguish changes caused by biological aging from those caused by lifestyle and those caused by disease. To do this longitudinal studies are needed. Knowledge of longitudinal changes in lipoproteins is limited because of the inherent difficulties in following cohorts of individuals over extended periods of time, and methodologies that limited widespread measurement of HDL-C and apolipoproteins prior to the mid-1970s. The window of opportunity to perform longitudinal studies of the natural history of lipoprotein changes with aging has closed, as treatment of clinically apparent dyslipidemia is standard clinical practice. In general, the cross-sectional studies demonstrate that total cholesterol, LDL-C, and TG concentrations increase in both men and women from the third to seventh or eighth decades of life, with a more pronounced increase in LDL-C concentration in women and important changes in women around the menopause. These increases in total cholesterol, LDL-C, and TG in general parallel changes in body composition seen with aging.
Typically, there is a decline in LDL-C and total cholesterol concentration in oldest age cohorts. For example, in NHANES III of 1988–1991, total cholesterol concentrations were on average 189 mg/dL in men aged 20 to 34 years and 221 mg/dL for men aged 55 to 64 years, but down to 205 mg/dL for men older than the age of 75 years. In the Honolulu Heart Study, total cholesterol levels were 8% lower in men older than age 85 years, as compared to men aged 71 to 74 years. This decline in cholesterol levels in the population older than age 75 years could reflect selective mortality, or be caused by changes in body composition, coexistent comorbid diseases, and poor nutrition. Indeed, longitudinal change in body weight over an 8-year period of time was an independent predictor of changes in total cholesterol, LDL-C, and HDL-C in older men and women in Rancho Bernardo, California.
The overall pattern of change in total cholesterol profiles across the age span in women is similar to that for men, but on average is 20 to 30 mg/dL above men from age 55 to 75 years. In NHANES III, 39% of women and 22% of men aged 65 to 74 years had a total cholesterol concentration above 240 mg/dL. However, the implications of “elevated” cholesterol in elderly people are not as straightforward as in younger age groups. In a subgroup of men aged 71 to 93 years from the Honolulu Heart Study, the age-adjusted incidence rates of coronary heart disease exhibited a significant U-shaped relationship with both total cholesterol and LDL.
In addition to the changes in the concentration of LDL, there are age-related changes in the LDL subclass population distribution that affect the atherogenicity and susceptibility of LDL to oxidation. The prevalence of individuals with a predominance of small, dense apo B-enriched LDL particles increases with age. In women, there are marked changes in lipoprotein concentrations and composition associated with menopause. In the Framingham Offspring Study, postmenopausal women had 16% higher values of total cholesterol, 62% higher TG, 23% higher LDL-C, and smaller LDL particles than premenopausal women. These changes in LDL composition adversely impact CAD risk, and are not detected by routine methods for measurement of lipoproteins.
There are also changes in HDL-C concentrations across the age span in both men and women. In men at puberty, there is a drop in HDL concentrations. The HDL-C concentration then remains fairly constant until the sixth or seventh decade of life, at which point there maybe an increase in the HDL-C concentration. This increase in HDL-C concentrations at older age noted in the cross-sectional studies also maybe the result of selective morbidity and mortality, as opposed to true age-associated changes in HDL metabolism. However, some studies have found lower HDL-C concentrations in postmenopausal women. Analogous to LDL, there are also compositional changes in the HDL subfractions associated with menopause that may increase CHD risk.
Based on ATP III guidelines, the marked age-associated increase in the prevalence of hypercholesterolemia (60% of men and 77% of women older than 65 years of age with total cholesterol > 200 mg/dL) make millions of older adults candidates for lifestyle intervention. Millions of older individuals with prevalent CHD, CHD equivalents (diabetes, stroke, peripheral vascular disease, chronic kidney disease), or with multiple risk factors for CHD events also are candidates for cholesterol lowering-drugs. Controversies on whom to treat and the target criteria are discussed in greater detail later in the chapter. As discussed in the next section, an increasingly informed and proactive older population, and changes in professional opinion toward ever more aggressive therapy for hyperlipidemia are reflected in the large number of elderly people prescribed HMG-CoA reductase inhibitors (statins).
Secular Changes in Lipoprotein Lipid Concentrations and Prescribing Patterns
There have been substantial changes in lipoprotein lipid concentrations in the U.S. population over the past four decades (Figure 110-1). NHANES data demonstrates that from 1960 to 2002, secular changes in diet, increased use of lipid-lowering medications, and other factors resulted in lower mean total cholesterol concentration across the age span in both men and women. The greatest declines have occurred in the older populations. There was no change in mean HDL-C and a trend for an increase in TG. The increase in the proportion of adults using lipid-lowering medication, particularly in older age groups, likely contributed to the decreases in total and LDL-C concentration, while the heightened prevalence of obesity in the U.S. population probably contributed to the increase in plasma TG levels.
The secular decline in cholesterol concentration maybe accelerating. A retrospective analysis of data from nearly 80 million LDL-C tests performed at Quest laboratories across the United States from 2001 through 2004 for adult patients aged 20 years and older demonstrated that the average serum LDL-C concentration declined from 124 mg/dL at the beginning of 2001 to 112 mg/dL at the end of 2004. The decline in average LDL-C concentration was observed across all age groups, but was most pronounced for tests performed on older patients. The decrease was greatest (approximately 13%) for people aged 70 years and older and least pronounced (approximately 7%) for the 20-to-39-year age range. These analyses were not adjusted for medication usage or disease, and patients referred for assessment of their lipoprotein lipids may not be representative of the general population.
This decline in cholesterol concentration could have a significant impact on CVD morbidity and mortality. Conversely, secular changes resulting in an increased prevalence of obesity may worsen the manifestations of the metabolic syndrome, i.e., raise TG concentration, lower HDL-C concentration, and shift the distribution of HDL and LDL lipoprotein particle distribution toward smaller, denser, more atherogenic particles, worsen insulin resistance, and raise blood pressure. Therefore, LDL-C concentration alone maybe misleading as in these older people there will be more LDL particles for any cholesterol concentration if the LDL particles are small and dense. Such changes may require a change in the approach to the identification and treatment of dyslipoproteinemia toward therapies that modify both the concentration and composition of HDL and LDL, and reduce the high number of apo B and dense LDL particles, as well as treat other components of the metabolic syndrome. Combination therapy of LDL-lowering drugs such as HMG-CoA reductase inhibitors with niacin, cholesterol absorption inhibitors (ezetimibe), or fibrates in combination with insulin sensitizers to reduce insulin resistance, glucose, and plasma triglyceride maybe required to achieve lipid-lowering targets (see “Management” later in the chapter.).
In 1997, fewer than 12% of the 38 million Medicare beneficiaries (4.4 million persons) used at least one statin. By 2002, roughly 27% of the 41 million Medicare beneficiaries (11 million individuals) used the drugs. The 2005 U.S. Agency for Healthcare Research and Quality (AHRQ) statistics (http://www.meps.ahrq.gov/mepsweb) indicates that statin use doubled between 1997 and 2002 in the Medicare recipients older than 65 years of age. The statistics from AHRQ’s Medical Expenditure Panel Survey do not include Medicare patients in nursing homes or other institutional settings. When examined by age category, about 31% of those 65 to 74 years of age and 30% aged 75 to 84 years used a statin in 2002, but only 12% the of beneficiaries were older than 85 years.
Despite the large increase in number of prescriptions, many feel that statin therapy is underutilized. A study by Ko of elderly residents in Ontario demonstrated that paradoxically, older individuals with the highest cardiovascular risk and risk for death were the least likely to be prescribed statins. This was because of a largely sharp age-related decline in the prescribing of statins. This problem of underuse of statins is further compounded by patient noncompliance. Concerns about adverse side effects may also deter health care providers from prescribing these medications to the elderly. However, concerns about side effects are not supported by the available data, as a meta-analysis of 35 randomized controlled trials and examination of Medicare data bases demonstrate that statins as a class are safe with a risk of transaminase elevation in about 4/1000 patients and a risk of fatal rhabdomyolysis of about 0.15 per 1 million statin prescriptions (the risk was 12-fold higher in the now withdrawn cerivastatin). Contrary to some clinical observations, the rate of myalgias (5%) and creatine kinase elevations (1%) in the meta-analysis was similar to placebo. Concerns about statins causing an increased risk of cancer are probably unfounded, as epidemiological data suggest that statins may actually reduce the rates of several cancers. Of note, the protective effects of statin therapy may go beyond simple lipoprotein reduction to include possible anti-inflammatory, antioxidant, and antithrombotic effects, as well as effects on nitric oxide metabolism. Several studies are currently underway addressing the benefits of statins with a variety of comorbid medical conditions including congestive heart failure, cerebrovascular disease, type 2 diabetes, and aortic stenosis.
Lipoproteins as Risk Factors for Coronary Heart Disease
Many case–control and prospective studies examining lipoprotein risk factors for atherosclerosis have focused on the impact of the traditional lipoprotein risk factors, that is, total cholesterol, LDL-C, and HDL-C. There is also substantial evidence that many older individuals have abnormalities in lipoprotein metabolism that are not detected by routine lipid profiles. These dyslipidemic syndromes include the apoE4 genotype, elevated levels of Lp(a), and the atherogenic LDL pattern B phenotype. These disorders of lipoprotein metabolism are more common than familial heterozygous hypercholesterolemia and familial multiple lipoprotein hyperlipidemia in both the general population and CHD patients, and may play major roles in the pathogenesis of CHD in older individuals. In this section, the evidence that dyslipidemia is a risk factor for CHD in the elderly population is reviewed.
There is convincing evidence that elevated levels of total cholesterol and of LDL-C increase the risk for CHD in middle-aged men. The evidence supporting elevated levels of LDL-C as a risk factor for CHD in the elderly population, particularly elderly women, is mixed. In pooled data presented at a National Heart, Lung, and Blood Institute (NHLBI) conference, in 21 of 24 studies of men aged 65 years and older, the relative risk was >1.00 for total cholesterol concentrations >240 mg/dL, as compared to cholesterol levels <200 mg/dL. The pooled relative risk was 1.32. By comparison, for women aged 65 years and older, in 10 of 16 studies, the relative risk was >1.00 for cholesterol >240 mg/dL, as compared to cholesterol levels <200 mg/dL. The pooled relative risk was 1.12. Although statistically significant, the relative risk for CHD in older individuals with hypercholesterolemia is lower than the relative risk observed in studies of men younger than 65 years of age.
There are many factors that may account for the disparities between the studies that have examined the relationship between cholesterol levels and CHD in the elderly. One explanation maybe a nonlinear relationship between cholesterol levels, CHD events, and total mortality, that is, a J- or U-shaped curve. In this situation, individuals with the highest or lowest cholesterol levels are at increased risk for CHD, as compared to those with intermediate levels. Another confounder in the association between cholesterol levels and CHD outcomes in the elderly person is that cholesterol levels measured at a given time may not accurately reflect the lifelong average exposure to plasma cholesterol, leading to spurious conclusions. For example, at age 70 years, the measured total cholesterol level maybe 220 mg/dL, whereas the average cholesterol level over the adult life span could have been 180 or 250 mg/dL. As a result, longitudinal studies that examine both the baseline cholesterol level and its change over time are needed to provide insight into the predictive values of cholesterol levels for subsequent CHD and all-cause mortality in the elderly population. For example, in a Finnish 30-year longitudinal study, there was a U-shaped relationship between the change in cholesterol level and coronary and all-cause mortality. The authors concluded that this U-shaped relationship may confound the association of cholesterol with CHD risk in the elderly. A potential way out of this conundrum is to focus on LDL cholesterol as advocated by the ATP III guidelines. Not only is this more closely linked to CVD, but increasing clinical trial data tie improvements in LDL-C to those in CHD clinical endpoints (see below).
The majority of studies report data on the relative risk of hypercholesterolemia for CHD morbidity and mortality. However, some investigators suggest that the attributable risk is a more useful parameter for making clinical decisions regarding treatment of hypercholesterolemia in the elderly. In this context, attributable risk is the difference in the CHD mortality rate in subjects with a high lipid concentration and the CHD mortality rate in subjects with a lower or desirable lipid concentration. Attributable risk provides an estimate of the degree to which cause-specific mortality (in this case CHD mortality) would be lowered if the putative risk factor were lowered to a normal level. Unlike relative risk, attributable risk is very sensitive to the underlying mortality rate in “normals”, that is, the mortality rate in subjects with normal or desirable cholesterol levels. The MRFIT data permits an analysis of how the relative and attributable risks of hypercholesterolemia for CHD mortality vary with age. In MRFIT, the relative risk for CHD mortality for a man in the upper quintile of the population declined from over 7 in men aged 35 to 39 years to 2 for men aged 55 to 59 years (Figure 110-3). However, as a result of age-associated increases in the CHD death rate in men with “normal” cholesterol, the attributable risk for CHD in the upper versus lowest quintile increased progressively with age, from 0.7% for men aged 35 to 39 years compared to 1.9% for men aged 55 to 59 years. As a result of the age-related increase in the attributable risk for hypercholesterolemia, there maybe substantial public health benefit, i.e., the ability to prevent a large numbers of deaths by treating hypercholesterolemia in the elderly. Furthermore, as discussed in the Management section of this chapter, the number of subjects who have to be treated to prevent one death or the inverse of the attributable risk is substantially lower in older than in younger adults. Thus, pharmacologic interventions to decrease morbidity and mortality from CHD maybe more cost-effective in older compared to younger people.
Figure 110-3.
The effect of age on the relative and attributable risk of hypercholesterolemia for CHD death in patients of the Multiple Risk Factor Intervention Trial (MRFIT). Although the relative risk (RR) for CHD death for individuals with hypercholesterolemia declines with aging, because of age-associated increases in the absolute death rate from CHD, the attributable risk (AR) for CHD in the upper versus lowest quintile increased progressively with age. (From Malenka DJ, Baron JA. Cholesterol and Coronary Heart Disease. The importance of patient-specific attributable risk. Arch Intern Med. 1988;148:2247–2252.)
A preponderance of studies demonstrate that measurement of HDL-C provides additional information on risk for CHD and stroke in the elderly. In the Framingham Study, 4- and 12-year follow-up data demonstrate inverse relationships between HDL-C concentrations and CHD incidence. In Framingham men 50 to 79 years of age, the relative risk for all-cause mortality in men with an HDL-C < 35 mg/dL compared to those whose HDL-C >54 mg/dL was 1.9 and risk of CHD mortality was 4.1. In women, the relative risk for all-cause mortality was 1.5 and the risk for CHD mortality was 3.1 (HDL-C < 45 mg/dL vs. > 69 mg/dL). In data from three Established Population for the Epidemiologic Study of the Elderly (EPESE) sites, subjects aged 71 to 80 years in the lowest HDL-C category (<35 mg/dL) were four times more likely to die from CHD than those with HDL-C above 60 mg/dL. A 10-year prospective study of lipid risk factors for CHD in 989 older subjects in Marshfield, Winconsin, showed that survival among men was associated with higher HDL-C and a lower total cholesterol/HDL ratio. However, there was no relationship between any of the lipid concentrations and all-cause mortality in women older than 65 years of age. There was a 1.24-fold increased risk of having peripheral arterial disease and a 1.36 risk of having a stroke for a 10 mg/dL decrement of HDL-C in older men and women, after controlling for other risk factors. Therapy with gemfibrozil increased HDL-C and reduced the risk of stroke in older men with prevalent CHD. These findings support the use of measurement of HDL-C along with total cholesterol to predict CHD risk in older individuals.
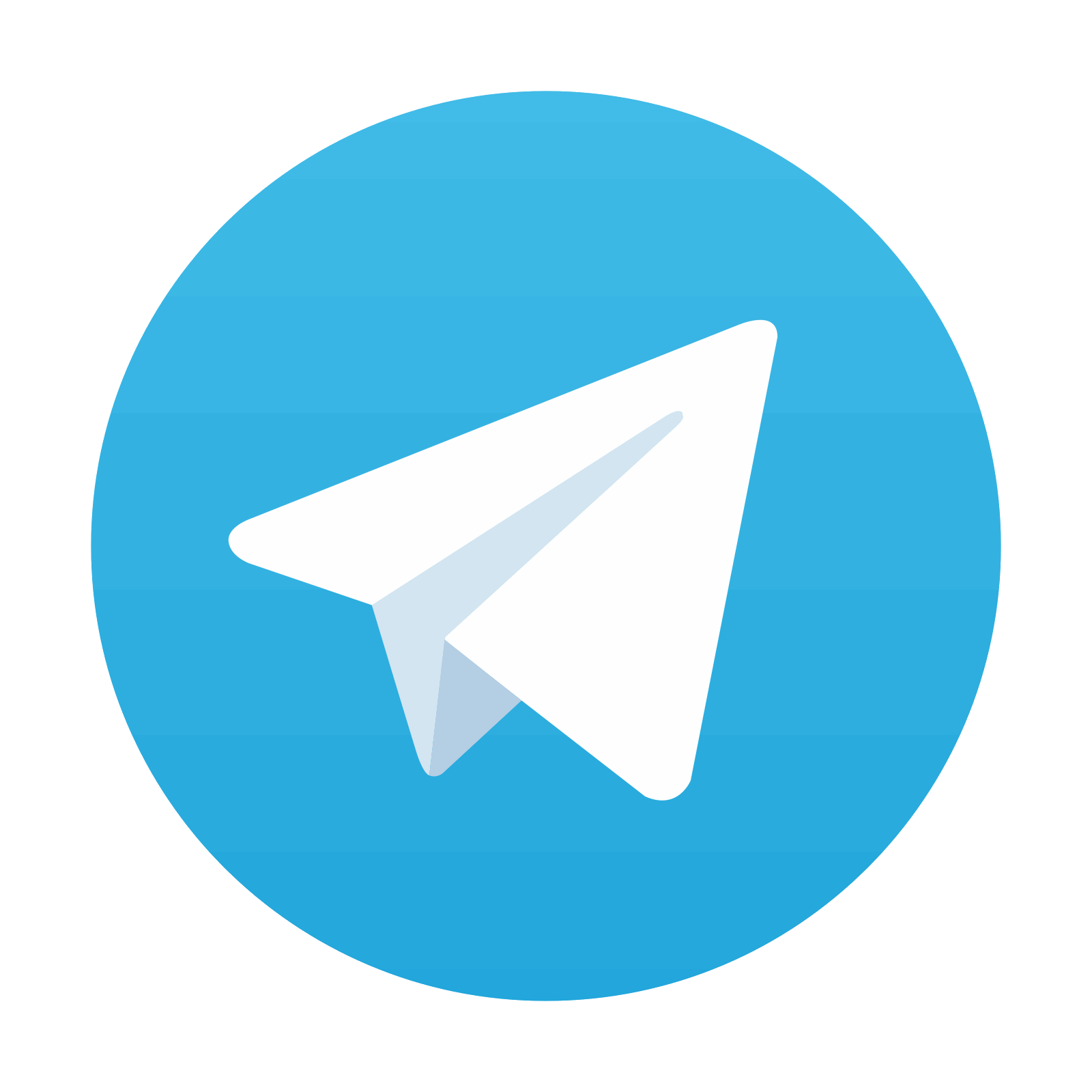
Stay updated, free articles. Join our Telegram channel

Full access? Get Clinical Tree
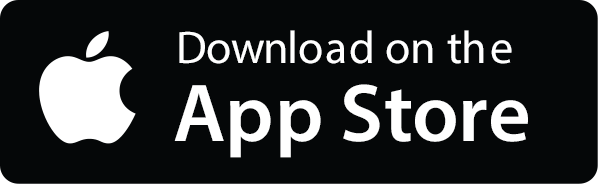
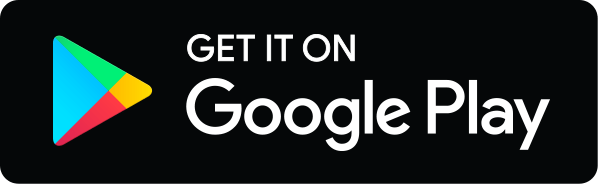