This review presents the indications and contraindications (pros and cons) for the potential use of erythropoietin (Epo) as a treatment in β-thalassemia and sickle cell anemia (SCA). Its high cost and route of administration (by injection) are obvious obstacles, especially in underdeveloped countries, where thalassemia is prevalent. We believe that from the data summarized in this review, the time has come to define, by studying in vitro and in vivo models, as well as by controlled clinical trials, the rationale for treating patients with various forms of thalassemia and SCA with Epo alone or in combination with other medications.
Key points
- •
The clinical experience with erythropoietin (Epo) treatment in β-hemoglobinopathies is limited, and well-controlled clinical trials have not been performed.
- •
Its high cost and route of administration (by injection) are obvious obstacles, especially in underdeveloped countries, where these diseases are prevalent.
- •
We believe that patients with non–transfusion-dependent thalassemia, with low hemoglobin (Hb) levels (<7.0 g/dL) or patients with thalassemia minor, in whom precipitating factors such as infections, operations, pregnancy, and so forth can significantly decrease their Hb levels, might benefit from Epo treatment, at least temporarily.
- •
We suggest that from the data summarized in this review, the time has come to define, by studying in vitro and in vivo models, as well as by controlled clinical trials, the rationale for treating patients with various forms of thalassemia and sickle cell anemia with Epo alone or in combination with other medications.
Erythropoietin (Epo), a hormone released in response to hypoxia mainly in the kidneys, is the major regulator of red blood cell (RBC) production (erythropoiesis). By binding to a surface receptor, it stimulates proliferation and inhibits apoptosis of erythroid progenitors and precursors in the bone marrow. The clinically approved, commercially available, recombinant human Epo preparations include epoetin-α, epoetin-β, and the long-acting darbepoetin-α, all effective stimulators of erythropoiesis. They are widely used for the treatment of chronic anemia of different causes (eg, in patients treated by chemotherapy or hemodialysis as well as in patients with myelodysplastic syndrome).
The β-hemoglobinopathies, β-thalassemia and sickle cell anemia (SCA), are hereditary hemolytic anemias caused by mutations in the β-globin cluster. In β-thalassemia, the mutations reduce (β + ) or abolish (β o ) the synthesis of β-globin chain and consequently the production of hemoglobin (Hb) A (α 2 β 2 ). In SCA, the mutation causes a structural change in the β-globin (β S ), leading to production of an abnormal Hb (HbS). Despite the state of chronic anemia, the level of serum Epo in thalassemia, and to some extent in SCA, is low relative to the degree of anemia, probably because of its increased use and consumption. Several studies reported improvement of the anemia in these patients after treatment with Epo. For example, darbepoetin-α was shown to substantially increase Hb levels in patients with HbE–β-thalassemia.
Although the therapeutic effect of Epo is mainly related to stimulation of erythropoiesis, since Epo is a pleiotropic cytokine, its administration into patients with β-hemoglobinopathies can be associated with a variety of beneficial and deleterious effects. This review presents the indications and contraindications (pros and cons) for the potential role of Epo as a treatment in such patients.
Epo and erythropoiesis
The main effect of Epo is on erythropoiesis. Epo is a 30.4-kDa glycoprotein produced primarily in the adult kidney under the control of an oxygen-sensing mechanism. Low tissue oxygen tension induces EPO gene expression through both transcriptional activation and messenger RNA stabilization. The mechanism involves activation of hypoxia inducible factor 1, a transcription factor that binds to a hypoxia responsive element in the 3′ flanking region of the EPO gene. The erythropoietic effect of Epo is mediated by a surface homodimeric Epo receptor (EpoR), a class 1 cytokine receptor, which is present on erythroid progenitors and precursors. After binding to the receptor, a signal transduction cascade is initiated, which involves the activation of a cytoplasmic, nonreceptor protein tyrosine kinase, Jak2, and the downstream signaling molecule Stat5, a cryptic cytoplasmic transcription factor.
In the β-hemoglobinopathies, erythropoiesis is increased in the bone marrow and often in extramedullary sites. However, it is mostly ineffective; it does not result in mature RBC because of increased apoptosis. Because Epo affects erythropoiesis by stimulating proliferation and by preventing apoptosis, it can be speculated that Epo treatment would reduce ineffective erythropoiesis. Although all erythroid precursors in the β-hemoglobinopathies carry the mutated genotype, their abnormal phenotypic expression varies: in SCA, most precursors produce β S -globin, and in β-thalassemia, low or no β-globin chains (depending on the genotype) and, therefore, have an excess of α-globin chains, the main cause for membrane damage and, consequently, short survival. In both diseases, some cells produce β-like δ-globin and γ-globin chains. By binding to α-chains, these β-like chains form HbA 2 (α 2 δ 2 ) and fetal Hb (HbF) (α 2 γ 2 ) and thereby reduce the α-chain excess in β-thalassemia and the HbS in SCA. Consequently, these cells are less damaged and survive longer. Epo stimulates both types of precursors, thereby increasing the total erythroid mass of both the pathologic and the less pathologic RBCs.
Epo and erythropoiesis
The main effect of Epo is on erythropoiesis. Epo is a 30.4-kDa glycoprotein produced primarily in the adult kidney under the control of an oxygen-sensing mechanism. Low tissue oxygen tension induces EPO gene expression through both transcriptional activation and messenger RNA stabilization. The mechanism involves activation of hypoxia inducible factor 1, a transcription factor that binds to a hypoxia responsive element in the 3′ flanking region of the EPO gene. The erythropoietic effect of Epo is mediated by a surface homodimeric Epo receptor (EpoR), a class 1 cytokine receptor, which is present on erythroid progenitors and precursors. After binding to the receptor, a signal transduction cascade is initiated, which involves the activation of a cytoplasmic, nonreceptor protein tyrosine kinase, Jak2, and the downstream signaling molecule Stat5, a cryptic cytoplasmic transcription factor.
In the β-hemoglobinopathies, erythropoiesis is increased in the bone marrow and often in extramedullary sites. However, it is mostly ineffective; it does not result in mature RBC because of increased apoptosis. Because Epo affects erythropoiesis by stimulating proliferation and by preventing apoptosis, it can be speculated that Epo treatment would reduce ineffective erythropoiesis. Although all erythroid precursors in the β-hemoglobinopathies carry the mutated genotype, their abnormal phenotypic expression varies: in SCA, most precursors produce β S -globin, and in β-thalassemia, low or no β-globin chains (depending on the genotype) and, therefore, have an excess of α-globin chains, the main cause for membrane damage and, consequently, short survival. In both diseases, some cells produce β-like δ-globin and γ-globin chains. By binding to α-chains, these β-like chains form HbA 2 (α 2 δ 2 ) and fetal Hb (HbF) (α 2 γ 2 ) and thereby reduce the α-chain excess in β-thalassemia and the HbS in SCA. Consequently, these cells are less damaged and survive longer. Epo stimulates both types of precursors, thereby increasing the total erythroid mass of both the pathologic and the less pathologic RBCs.
Epo and HbF
HbF plays an important role in the pathophysiology of thalassemia and SCA ( Fig. 1 ). Epidemiologic studies have indicated that increased HbF ameliorates the clinical symptoms of the underlying disease. HbF production can be specifically stimulated by a variety of pharmacologic agents, including hydroxyurea which is the only clinically approved drug, mainly in SCA. The mechanism of hydroxyurea stimulation of γ-globin synthesis and HbF production is still unclear; it has been suggested that it involves nitric oxide generation.
In addition to its effect on total Hb by increasing the production of RBC, Epo treatment can specifically stimulate HbF, by stimulating γ-globin gene expression. However, the effect of Epo is not straightforward. Using a culture system for growing human erythroid precursors, we found that a constant high level of Epo significantly increased the yield of erythroid cells and total Hb, but it had no effect on the proportion of HbF. However, when Epo concentration was reduced during the culture period, the proportion of HbF increased compared with cultures treated with a fixed, high or low, Epo concentration. The possible mechanism of this effect involves changes in the intensity of the stimulus that Epo exerts on Hb-producing precursors ( Fig. 2 ). This stimulus is influenced by the availability of surface EpoR and by the concentration of Epo. EpoR is decreased during cell maturation, resulting in a decrease in HbF production content from high in early precursors to low, when HbA takes over in mature RBC. Epo concentration decreases after Epo treatment of anemic patients, in whom serum Epo level fluctuates from high (after administration) to low (because of its short survival in vivo). When high HbF-containing erythroid precursors are shifted from high to low Epo, their maturation is synchronized and accelerated, shortening the period of HbA production, leaving a relatively high proportion of HbF. Changes in Epo levels, and thereby in Epo stimulus, also occur in stress erythropoiesis, which is associated with increased HbF. Attempts to increase HbF in vivo, either by Epo alone or in combination with hydroxyurea, produced contradictory results. This situation could be because of the different timing, dose, and frequency of Epo administration similar to the results in cultured cells mentioned earlier.
Epo and iron overload
Iron overload (IO) is a major problem in patients with thalassemia and to some extent, also in SCA, affecting both morbidity and mortality, mainly in older patients. The major cause of IO in thalassemia is repeated blood transfusions, the main therapeutic modality in the severe forms of the disease, which introduce iron (in the form of Hb in the transfused RBC) beyond the capacity of the body to dispose of it. Another cause of IO, which exists even in nontransfused patients, is increased iron uptake from the gastrointestinal tract, which is mediated by reduced production of hepcidin, the master regulator of iron homeostasis. Hepcidin inhibits iron transport across the gut mucosa, thereby preventing excess iron absorption and inhibits transport of iron out of macrophages (where iron is stored), thus lowering its mobilization. Hepcidin functions by binding to the iron export channel ferroportin, which is located on the basolateral surface of the enterocytes and the plasma membrane of reticuloendothelial macrophages. Binding to ferroportin leads to its intracellular degradation and consequently reduces iron uptake and mobilization. Hepcidin is produced in the liver, and its production is regulated by multiple factors, including iron and cytokines such as interleukin 6. In thalassemia, hepcidin production is reduced, leading to increased iron absorption. The main cause for the low levels of hepcidin in thalassemia is believed to be augmented erythropoiesis, in which the body attempts, ineffectively, to overcome the anemia. The mechanism in not clear, but it was suggested to involve an increase in the synthesis of the growth differentiation factor 15 (GDF-15).
Iron is transported in the circulation bound to transferrin and is taken up by cells through a transferrin receptor. Inside the cells, most of the iron is bound, in a redox-inactive form, to various components such as Hb, heme, and cytochrome C, and the excess is stored in ferritin. When serum iron exceeds the binding capacity of transferrin, it is present in the form of non–transferrin-bound iron. This form of iron enters cells through transferrin-independent pathways to form labile iron pool (LIP), which was suggested as a transitory intermediate between the cellular iron pools. LIP is redox active and participates, through the Fenton and Haber-Weiss reactions, in the generation of reactive oxygen species (ROS), which, when present in excess, are cytotoxic. It is postulated that this is the main reason of morbidity and mortality caused by IO in major organs of patients with thalassemia. The administration of Epo can affect IO in 2 different indirect ways through its effect on erythropoiesis: on the one hand, it increases the use of iron, with a decrease in iron levels, but on the other hand, it may increase iron uptake by reducing hepcidin production, via GDF-15, with an increase in iron levels ( Fig. 3 ).
Administration of Epo is usually supplemented by iron. This factor may be crucial for anemia associated with iron deficiency, when iron supply is a limiting factor of erythropoiesis, but it may not be required when iron supply is abundant. We studied the effect of iron supplementation in patients with chronic renal failure on dialysis, in whom the kidneys fail to produce Epo, and consequently, the patients suffer from anemia. These patients are treated by weekly injections of Epo, which is usually supplemented with intravenous iron. By using T2-weighted magnetic resonance imaging, we have shown iron deposition in the liver, spleen, and pancreas. Stopping iron supplementation for up to 1 year reduced IO (monitored by decrease in serum ferritin and transferrin saturation levels), without interfering with the Hb-stimulating effect of Epo, indicating that under these conditions, the stored iron could be mobilized for erythropoiesis. These results raise the question of the minimal iron supplementation required in order to support erythropoiesis during Epo treatment and suggest that in patients with IO, such as in thalassemia, Epo may be given to increase the Hb level without iron supplementation. It is possible that such iron-free Epo treatment may reduce the consequences of IO by mobilizing iron not only from macrophages but also from parenchymal cells of vital organs (heart, liver), which are the main target of IO damage.
Epo and oxidative stress
In β-thalassemia, as well as in SCA, although the primary lesion is mutations in the β-globin gene, the damage to the RBC and other cells is mediated in part by oxidative stress. The homeostasis of the cellular oxidative status is maintained by the balance between oxidants, such as the ROS and antioxidants, such as reduced glutathione (GSH). When the balance is tilted by an increase in oxidants or a decrease in antioxidants, oxidative stress ensues. It has been shown that in thalassemia and SCA, RBC are under oxidative stress, caused mainly by IO, as discussed earlier.
Another contributor to oxidative stress in thalassemic RBC is Hb instability. Excess α-globin chains form unstable tetramers that dissociate into monomers, which, after a change in their tertiary structure, are oxidized first to methemoglobin and then to hemichromes. The following steps of the subunit disintegration are release of heme and iron while the protein precipitates both in the cytosol and the plasma membrane. The outcome of this chain of events is increased formation of ROS, which is catalyzed by free iron, with deleterious effects on the membrane lipids and proteins, including oxidation of membrane protein band 4.1 and a decrease in spectrin/band 3 ratio.
Using flow cytometry, we showed increased generation of ROS with a concurrent decreased content of GSH, in thalassemic RBC compared with normal RBC at basal level, as well as after an oxidative insult, such as treatment of the cells with H 2 O 2 . These effects were associated with RBC membrane changes, including lipid peroxidation and externalization of phosphatidylserine, a marker of cell senescence. The changes in the RBC membranes resulted in increased susceptibility to hemolysis and to phagocytosis by macrophages. Oxidative stress was also found in the polymorphonuclears and platelets of these patients, explaining, in part, their propensity for recurrent infections and thromboembolic complications.
Several studies suggested that Epo reduces oxidative stress: starvation of rats, which reduces endogenous Epo production, was found to increase lipid peroxidation of the RBC membrane, whereas administration of Epo reversed the effect. Improved antioxidant status was also found after Epo treatment of newborn rabbits. This situation could be caused by use of excess iron for developing erythroid precursors, as discussed earlier, thus making it unavailable for ROS generation.
The antioxidant effect of Epo treatment was first suggested for patients with chronic renal failure on dialysis. RBC in these patients are under oxidative stress, which results in externalization of phosphatidylserine, a marker of senescence, which tags RBC for elimination by phagocytosis. Epo treatment of these patients resulted in reduced lipid peroxidation concomitant with an increase in superoxide dismutase, catalase, and other antioxidant activities. It also caused, within 4 hours, a decrease in the number of RBC showing surface phosphatidylserine. Thus, although the main effect of Epo is related to stimulation of erythropoiesis and improvement in the anemia, as discussed earlier, it was suggested that in these patients, the Epo effect may also be associated with prolonging the life span of mature RBC.
These reports raise the question: can Epo reduce oxidative stress in thalassemia and SCA? In an attempt to answer this question, we investigated the effect of Epo on the oxidative status on normal and β-thalassemic RBC and platelets. When diluted human blood samples were incubated with Epo, ROS levels were decreased and GSH levels were concurrently increased in RBC and platelets from both normal and thalassemic donors compared with untreated cells. These effects were time dependent, starting within 10 to 15 minutes after exposure to Epo. The effect was noted in both unstimulated and H 2 O 2 -stimulated cells, indicating that Epo decreased the basal ROS of the cells as well as their ability to generate ROS in response to oxidative stress. We further correlated the effects of Epo on oxidative stress, senescence markers, and susceptibility to undergo hemolysis and phagocytosis in thalassemic RBC incubated in their autologous plasma for 3 days with or without Epo. The results showed that concomitant with the decrease in ROS and the increase in GSH, Epo treatment increased their staining with calcein and decreased their exposure of PS, markers of cell senescence, as well as hemolysis and phagocytosis.
The in vivo effects of Epo were shown 2 hours after its intraperitoneal injection (5000 U/kg) to heterozygous (Hbb th3/ + ) β-thalassemic mice. The ROS and lipid peroxides of their RBC were significantly reduced with a concomitant increase in GSH levels compared with control mice.
Although in these studies, the effects on oxidative stress required Epo concentrations Epo (1–2 U/mL) higher than normal serum levels, continuous, accumulative subthreshold effects, which could not be detected by the methodology used, at physiologic Epo concentrations are possible. Epo is obviously not a practical antioxidant drug, because it is less potent and more expensive than other antioxidants, and, in addition, has to be administrated by injection. Nevertheless, under conditions at which Epo levels are very high, such as during severe anemia (eg, after massive bleeding or in aplastic anemia), or after its administration to patients on hemodialysis or after chemotherapy, its protective effect as an antioxidant on RBC and platelet survival should also be considered.
The mechanism underlying the short-term effect of Epo on the oxidative stress of mature RBC is not clear. Epo affects erythroid cells through their surface EpoR, but mature RBC (and reticulocytes) seem to lack EpoR. However, Myssina and colleagues reported binding of radiolabeled Epo to a low, albeit detectable, number of high-affinity EpoR on mature RBC. Mihov and colleagues reported that not only can recombinant human Epo bind specifically to mouse peripheral blood RBC but it can also upregulate their nitric oxide production. Interaction of Epo with the mature RBC membrane was also reported by Baciu and Ivanof. To probe the possibility that EpoR is involved in mediating the effect of Epo on mature RBC, we inhibited Jak-2, a crucial step in the signal transduction pathway by EpoR, by Jak inhibitor I. Treatment of thalassemic RBC with this inhibitor did not inhibit the antioxidant effect of Epo.
Alternatively, the protective effect of Epo may be mediated through scavenging activity in the extracellular milieu; Epo contains more basic than acidic amino acids and many charged residues that may act as a “sink” for ROS (eg, hydroxyl radicals). Moreover, Epo is a highly sialidated glycoprotein. It has been reported that mucin, a typical sialic acid containing high-molecular-weight glycoprotein, is an antioxidant and that sialic acid is crucial for this activity. Mucin was also shown to enhance the antioxidant activity of polyphenols, probably by increasing their solubility. Sialic acid may also function intracellularly; Oetke and colleagues, using human hematopoietic cell lines, which are hyposialylated because of a deficiency in de novo sialic acid biosynthesis, reported efficient uptake and incorporation of free sialic acid. Other studies reported that thalassemic RBC have a lower content of sialic acid than normal RBC, and that sialic acid can be taken up by human RBC. In our experiments, using several methodologies, Epo, at the concentrations tested, did not show any ROS scavenging activity in a cell-free system.
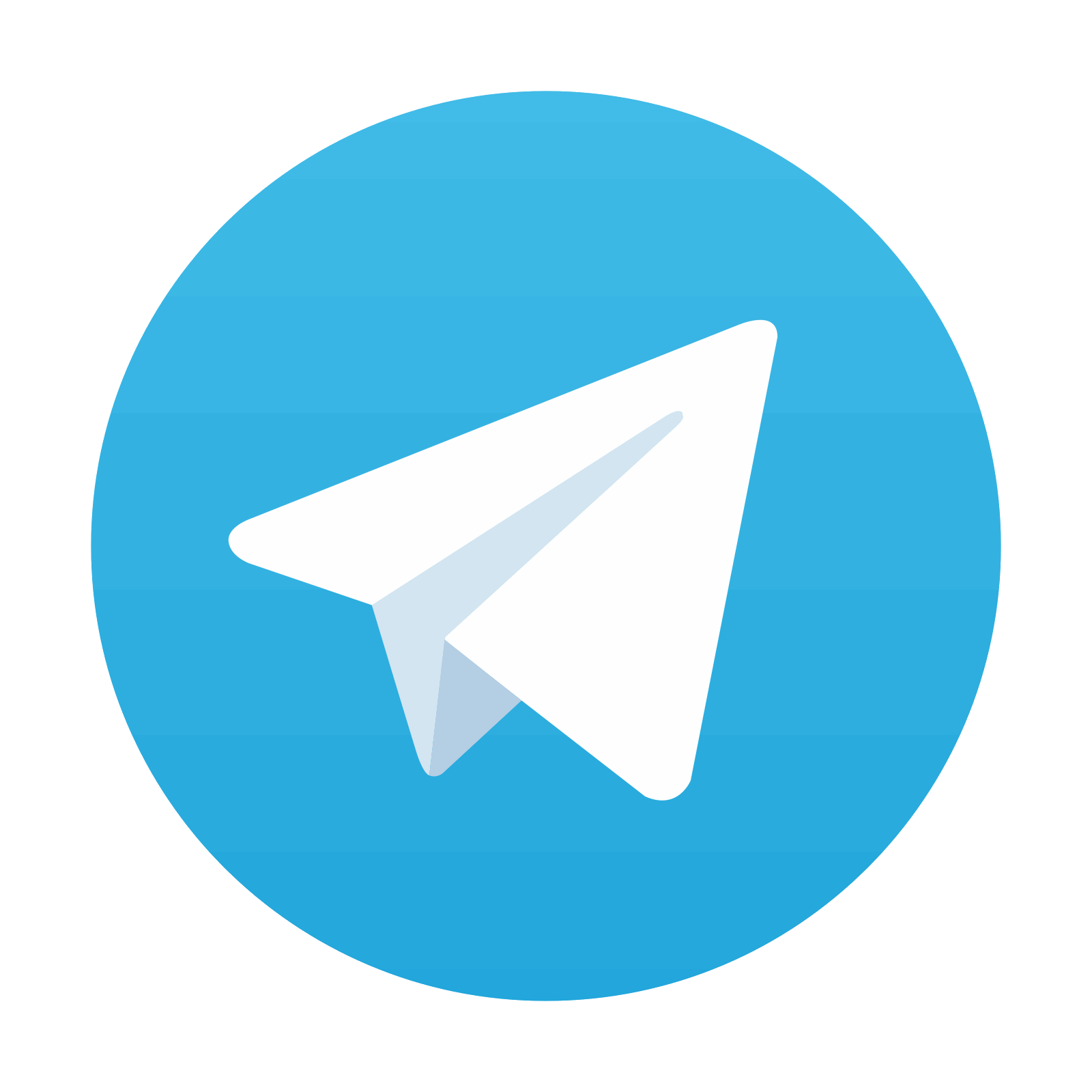
Stay updated, free articles. Join our Telegram channel

Full access? Get Clinical Tree
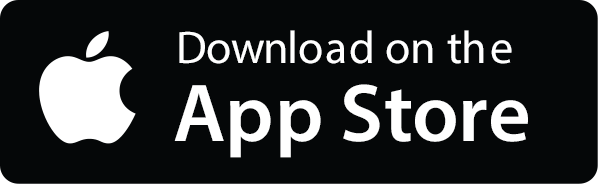
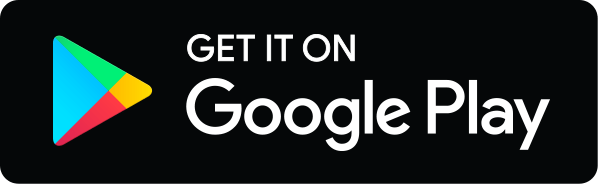