CHAPTER 11 Diet and Genotype Interactions
Nutritional Genomics
11.1 INTRODUCTION
Early studies of nutrient requirements typically sought to characterize the biochemical and clinical effects of nutrient depletion and repletion. More recently the focus has shifted towards an understanding of nutrient intake profiles associated with optimum health. What is clear is that individuals respond differently to the same intake of specific nutrients and that what constitutes adequacy in one person may be inadequate in another. Additionally, dietary intake profiles considered to increase risk of chronic disease are associated with different levels of risk in different individuals. Genetic factors are central to these inter-individual differences. Developments in molecular biology techniques have led to great progress in our understanding of the role of genetic constitution (genotype) in determining individual responses to food. They have also helped us to understand how nutrients affect the way in which the information in genes is used to produce specific proteins, in other words how the genes are expressed (phenotype). The term nutritional genomics is used to describe the study of nutrient–gene interactions.
11.2 A MOLECULAR APPROACH TO UNDERSTANDING NUTRIENT–GENE INTERACTIONS
The principles of gene expression
In order to understand the way in which proteins can be encoded by DNA it may be useful to refer again to the structure of DNA and RNA, described in section 4.4, under Protein synthesis. Briefly, DNA is a double helical structure of two polymers of nucleotides, each comprising a sugar, a phosphate and one of four bases (adenine, guanine, cytosine and thymine). The order of bases in a length of DNA codes for the order of amino acids to be incorporated into a protein. This code (the genetic code) is read in groups of three bases (codons). In RNA the sugar is ribose, rather than deoxyribose, and RNA contains the base uracil instead of the thymine in DNA. In the cell nucleus the synthesis of a particular protein is directed by the sequence of bases on a specific region of DNA which acts as a template to transcribe the sequence of bases into a complementary strand of messenger RNA (mRNA). This is possible because pairs of bases can form specific bonds with one another, namely adenine with thymine and cytosine with guanine (Fig 11.1). Transcription produces a molecule of mRNA, which is transferred from the nucleus to the cytoplasm. The information in the mRNA is then translated (used to specify the sequence of amino acids) into a polypeptide, a process that takes place on ribosomes. Once the polypeptide chain of a protein has been synthesized it will fold into its three-dimensional structure and may undergo further post-translational modifications to the fully functional protein (Fig 11.2).
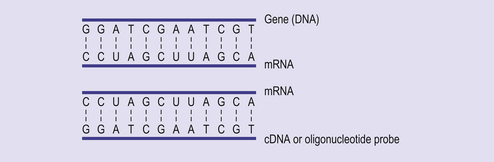
Figure 11.1 Coding of mRNA from DNA and complementarity of cDNA, or oligonucleotide, probes in the detection of mRNAs.
Regulation of gene expression
Regulation of gene expression by nutrients and other factors can, in principle, occur at any level from the initial transcription of genomic DNA through to the final modifications of the finished protein product.
Transcriptional and post-transcriptional regulation
Retinol (vitamin A) provides a good example of how a nutrient can influence transcription. Once in its target cell, retinol is converted to isoforms of retinoic acid which are transferred to the nucleus and act as ligands for the transcription factors, retinoic acid receptor (RAR) and retinoic X receptor (RXR). These transcription factors bind to a part of DNA called the retinoic acid response element (RARE), found in a number of genes, and thereby increase transcription of these genes (see chapter 5, section 5.3).
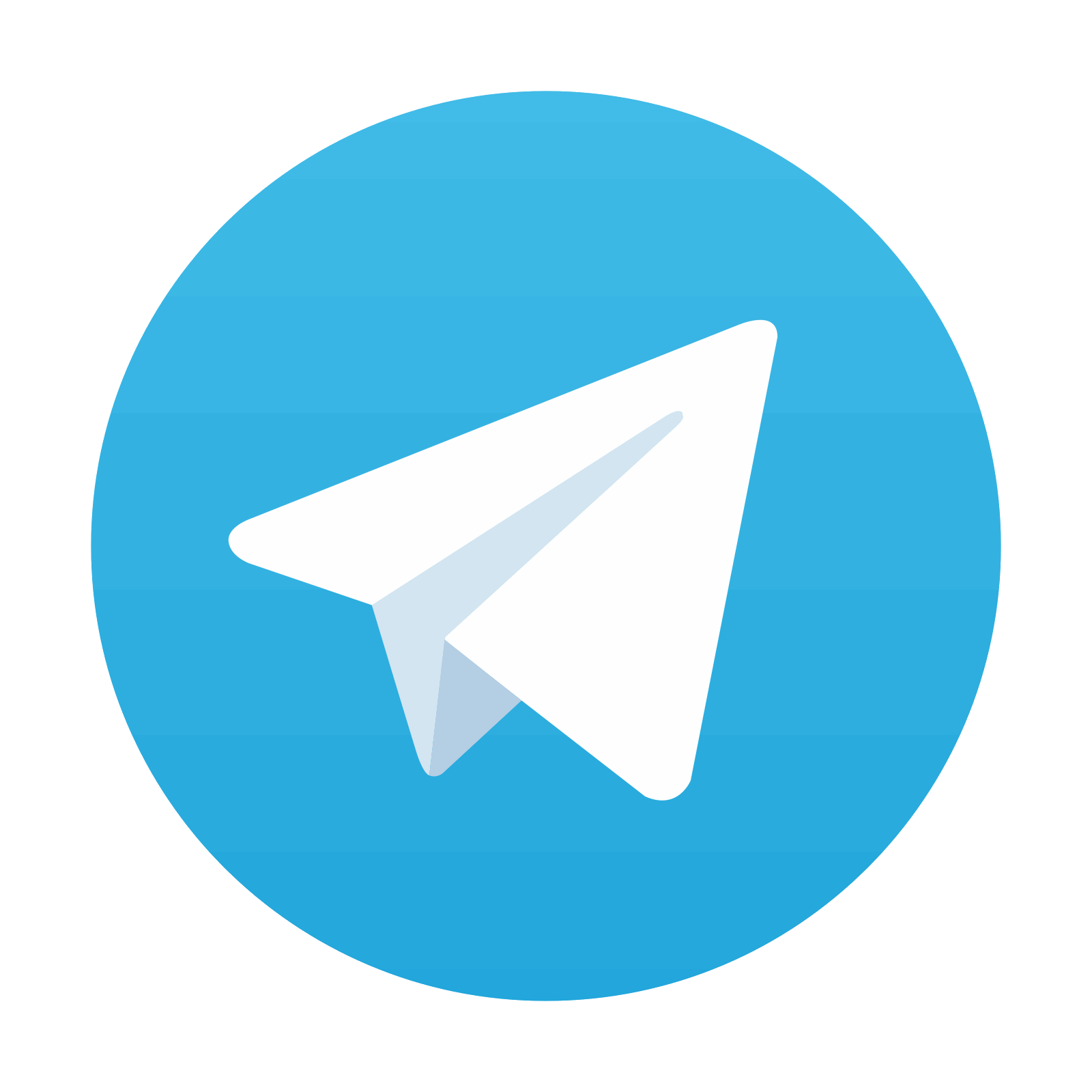
Stay updated, free articles. Join our Telegram channel

Full access? Get Clinical Tree
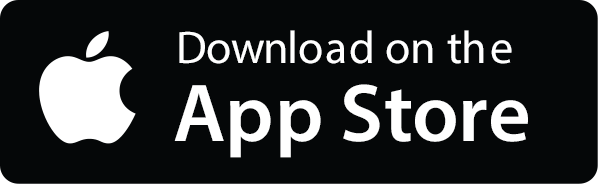
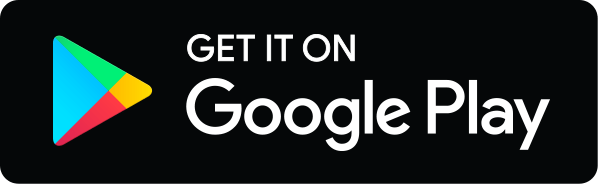