Chapter Outline
SPECIAL CONSIDERATIONS FOR THE HEMATOPOIETIC STEM CELL TRANSPLANT SURVIVOR
FOLLOW-UP CARE OF CHILDHOOD CANCER SURVIVORS
With ongoing advances in the diagnosis and treatment of childhood cancers, increasing numbers of children and adolescents diagnosed with cancer are becoming long-term survivors. However, even as higher cure rates are achieved, there is increasing recognition that the diagnosis and treatment of cancer during childhood and adolescence can have lifelong implications for health and well-being. Cancer therapy administered during critical periods of growth and development render childhood cancer survivors vulnerable to a variety of physical and emotional disorders often referred to as “late effects.” Some late effects can be diagnosed soon after completion of cancer-directed therapy, and others may not manifest until decades later. The severity of late effects ranges from minor disabilities to chronic conditions and even life-threatening morbidities, including second cancers, cardiac disease, and pulmonary dysfunction. Severe late effects can reduce a survivor’s life expectancy.
Although remarkable progress has been made in advancing our understanding of the adverse health outcomes associated with treatment of childhood cancer, survivors continue to experience significant long-term morbidity related to late effects. Increasingly it is recognized that the care of the child or adolescent diagnosed with cancer does not end with the completion of therapy but continues on the path into adulthood. Clinical focus during survivorship care shifts from treatment of the primary cancer and screening for its recurrence to the prevention, detection, and management of adverse consequences of prior therapy. Ultimately, ongoing research may result in the reduction or even elimination of late effects and in improved quality of life for childhood cancer survivors.
History and Overview
Prior to 1950, children and adolescents who developed cancer rarely survived. The first generation of survivors primarily consisted of children with Hodgkin lymphoma, sarcoma, and Wilms tumor who were successfully treated with surgery and high-dose, large-field radiation therapy. The widespread use of multiagent chemotherapy, beginning in the 1970s, resulted in dramatic increases in survival for the most common childhood cancer, acute leukemia, and continued improvement in survival for other pediatric cancers as well. By the mid-1970s, many forms of childhood cancer became treatable, and potentially curable. Continuing advances in treatment options for childhood cancer have continued to improved survival. Children with cancer have also benefited from improvements in diagnostic testing, resulting in earlier and more precise diagnosis and more timely delivery of necessary treatment. Enhanced supportive care including advances in intensive care, blood banking, and management of infectious disease and acute toxicities of therapy also contributed to successful outcomes. Currently, the overall 5-year survival for childhood cancer exceeds 80%, and for some cancer diagnoses 5-year survival is above 95% ( Fig. 72-1 ). There are estimated to be more than 300,000 adult survivors of childhood cancer in the U.S. population, and this number continues to grow by approximately 10,000 survivors each year.

Recognition of Late Effects
Along with these remarkable advances in survival there is a growing recognition that treatment for childhood cancer is associated with long-term complications or “late effects.” Late effects can be defined as adverse health outcomes experienced by childhood cancer survivors associated with previous cancer–directed therapy; these outcomes reduce survival or affect the “quality” of survival. Some late effects can be life threatening and contribute to the increased risk for early mortality observed in adults who are long-term survivors of childhood cancer. Other late effects, such as neurocognitive impairment, physical deformity, and infertility, can limit survivors’ social or economic opportunities and impair their overall quality of life. In contrast, some late effects may be “positive” effects, such as increase in psychological resiliency and decrease in smoking rates and the rates of other high-risk behaviors compared with those in the rest of the population.
Second cancers, cardiopulmonary disease, and infertility were among the first treatment-associated late effects recognized in childhood cancer survivors. These first descriptions were based on clinical observations in small numbers of the earliest survivors of childhood cancer treated primarily with surgery and irradiation in the 1960s. Early clinical observations created awareness about late effects and identified the need for further research defining treatment-related health outcomes in the growing numbers of survivors of childhood cancer.
Early Mortality
Follow-up studies of the earliest cohorts led to the observation that childhood cancer survivors are at risk for early mortality. These studies showed that late recurrence of the primary cancer and treatment-associated complications resulted in decreased long-term survival, 80% at 20 years after diagnosis compared with 97% expected survival in the age-matched general population. The majority of premature deaths observed in survivors in these early studies were attributed to late relapse, whereas treatment-associated second cancers and major organ toxicity accounted for 30% of deaths. It was also recognized that the risk for dying of a treatment-related cause increased with increasing intensity of therapy. Although late recurrence accounts for the majority of premature deaths observed in childhood cancer survivors, the risk for relapse diminishes over time while the risk for therapy-related mortality persists beyond 25 years of follow-up. Figure 72-2 depicts all-cause mortality in 5-year survivors of childhood cancer, and Figure 72-3 demonstrates all-cause mortality by underlying cancer diagnosis. It remains to be determined if current treatment protocols that are specifically designed to reduce late effects will continue to improve very long-term survival by reducing the risk for therapy-related mortality.


Identifying Risk Factors for Late Effects
After the early descriptive studies, registry-based investigations of larger groups of childhood cancer survivors were undertaken with the aim of identifying specific risk factors for late effects. These included the National Cancer Institute’s “Five Center Study,” a registry-based cohort that enrolled 2490 adult 5-year survivors diagnosed with cancer between 1945 and 1975 at age younger than 20 years who were followed prospectively for reproductive outcomes, second cancers, and mortality ; the “Late Effects Study Group,” a multiinstitutional cohort that analyzed the occurrence of second cancers in 9170 two-year survivors of childhood cancer diagnosed between 1936 and 1979 ; and cancer registry–based studies in the United Kingdom, the Netherlands, and the Nordic countries (Denmark, Finland, Iceland, Norway, Sweden) from the same early treatment era and beyond. These epidemiologic studies analyzed the association between specific treatments and adverse health outcomes of interest. Relative risks were calculated to compare rates of outcomes under study in cancer survivors with rates in a control population. Collectively these investigations made significant contributions to the understanding of late effects. Limitations of such studies of late-occurring outcomes included that recruitment was often not complete, resulting in a possible overrepresentation of childhood cancer survivors who were experiencing health conditions related to their prior therapy. In addition, some of the late effects observed in these cohorts were associated with historic treatments that have been replaced by more modern modalities. However, despite such limitations, these studies identified the relationships, now well accepted, between cancer therapy and early mortality, radiation therapy and secondary cancers, and alkylating agents and gonadal dysfunction.
To address the limitations of prior studies, the development of “survivor” cohorts to be followed prospectively was undertaken by investigators in North America and Europe. In North America, a multiinstitutional follow-up study of a cohort of over 14,000 childhood cancer survivors (the Childhood Cancer Survivor Study [CCSS]) was created, evaluating late effects in survivors diagnosed from 1970 to1986, at age younger than 21 years, and surviving at least 5 years from diagnosis. A recent expansion of the CCSS to include survivors treated between 1986 and 1999 will yield important analyses of the effects of more “modern” therapies, as well as reflect a more ethnically and racially diverse population in North America. The CCSS uses self-reported questionnaires to obtain health outcomes and analyzes the association between outcome and treatment variables documented in abstracted medical records. The primary end points investigated by the CCSS included second cancers, mortality, and heart disease. Large registry-based cohort studies of childhood cancer survivors treated in Great Britain, France, the Netherlands, and the Nordic countries are ongoing and continue to contribute to our understanding of survivorship. Within these cohort studies, the prevalence of and the risk factors for the development of late effects after childhood cancer have been better elucidated.
Interpreting Late Effects Research
Advances continue to be made in recognizing and categorizing adverse health outcomes in childhood cancer survivors. However, the study of late effects in cancer survivors has inherent challenges that must be considered when interpreting research findings. First, there is not universal implementation of a definition for “cancer survivor.” The definition chosen for a particular investigation determines eligibility for studies and dictates how results can be interpreted and generalized to specific populations of survivors. The Office of Cancer Survivorship at the U.S. National Cancer Institute considers an individual a cancer survivor from the moment of cancer diagnosis through the balance of his or her life regardless of degree of cancer control. Although this definition is meaningful, particularly from an advocacy perspective, it is not effective for the purposes of understanding late outcomes in a cured population. Survival 5 years beyond cancer diagnosis is the definition of cancer survivor that is often, but not universally, used for the purpose of research on late effects.
Another consideration is how investigators measure the late effect or outcome of interest. Self-report is frequently used to measure outcomes in epidemiologic studies of late effects. Although it is cost-effective, self-report can lead to reporting bias that results in inaccurate assessments of the incidence or severity of outcomes. Studies of smaller groups of survivors utilizing direct observation to delineate outcomes help to confirm self-reported outcomes, refine our understanding of these outcomes, and allow direct measurement of asymptomatic or early morbidity not evident by self-report. The disadvantages of this type of clinical studies are cost, complexity, and small sample size, which can limit interpretation of results.
The timing of assessments can also influence the results and interpretation of late effects research. Although it can be efficient to recruit survivors for follow-up studies during or soon after treatment, some late effects are not observed until decades after initial therapy. Studies with inadequate periods of follow-up might yield results that underestimate or overestimate late effects. Retaining survivors in observational cohorts over long periods of follow-up is challenging, and results may be biased toward retention of patients experiencing the outcomes under investigation. Consideration of the duration of follow-up is particularly important when applying results to populations of pediatric survivors, in whom late effects may emerge in the course of developmental change.
Another challenge when interpreting the results of late effect studies is that most results are reported as relative risks, the rate of an outcome observed in a cohort of survivors relative to the rate of the outcome observed in a control population. Elevated relative risks must be interpreted and communicated with caution, because even the smallest increase in an otherwise very rare outcome results in a very large relative risk; however, even a small or statistically insignificant increase in relative risk might have important clinical implications.
Finally, an inherent difficulty with studying late effects is that treatments for cancer continue to evolve; therefore the health outcomes of interest in follow-up studies are often reflective of historic or outdated treatments. Methodology does not exist to address this issue, so treatment era must be taken into consideration when interpreting the results of these investigations. Duration of follow-up, treatment era, cohort eligibility, and outcome measures are all aspects of study design that merit careful consideration when interpreting results of late effects research studies.
General Principles of Late Effects
Epidemiologic studies investigating the association between treatment and risk for adverse health outcomes have led to general principles that contribute to our thinking about an individual survivor’s potential for developing late effects ( Box 72-1 ). A childhood cancer survivor’s risk for developing late effects depends on the nature of prior treatment as well as individual characteristics, such as age at exposure, gender, or time since exposure.
First, the dose and intensity of the treatment is frequently correlated with risk for late effects. In general, survivors exposed to larger doses of therapy have worse outcomes. For example, risk for male infertility after treatment with gonadotoxic chemotherapy is directly related to dose; males exposed to the highest cumulative doses have the greatest risk for future infertility. In addition, a survivor’s likelihood for late effects is increased if there is exposure to more than one modality with related toxicities. For example, survivors treated concurrently with mediastinal radiation and cardiotoxic chemotherapy are at greater risk for cardiac late effects than those treated with either of those modalities alone.
Organ toxicity associated with a single treatment exposure can manifest clinically as different late effects depending on the timing of the clinical assessment. For example, consider a postmenarchal female adolescent treated with gonadotoxic chemotherapy. During treatment she might experience acute reversible amenorrhea. Years later, after resolution of acute menorrhea, the woman might experience infertility secondary to premature menopause. Over time, the same woman might have osteoporosis as a secondary late effect of chronic estrogen deficiency. Neurocognitive impairment after central nervous system (CNS) therapy provides another example of how the timing of assessment influences detection of late effects. Children treated with CNS therapy might not manifest treatment-related neurocognitive deficits until they reach a time when school work, employment responsibilities, or social interactions require more complex cognitive function. The clinical manifestations of a single treatment exposure can change over time and are influenced by the physical, emotional, and social needs of the individual survivor.
Duration of follow-up also influences the potential for late effects. Some irradiation-associated late effects, especially secondary malignancies, have long latency periods, with increases in cumulative incidence over time. In contrast, other treatment-related toxicities can be acute and resolve (bone marrow suppression) or occur late and be progressive (pulmonary fibrosis). The leukemogenic potentials of specific forms of chemotherapy appear to be associated with fairly discrete time frames after exposures: topoisomerase inhibiters are associated with occurrence of secondary leukemias within months of exposure and rarely beyond 5 years, whereas alkylating agents are associated with a later onset and a later, but still predictable plateau in cumulative incidence at 10 years after exposure. In addition, acute chemotherapy organ toxicity can predict late toxicity. For example, chemotherapy-associated renal toxicity diagnosed during therapy can persist as a chronic condition or resolve and be diagnosed again as chronic renal disease decades after treatment. Although treatment exposures are the largest determinants of future late effects, a survivor’s age or developmental stage during cancer treatment and his or her sex, family history, existing comorbidities, and health behaviors can all modulate risk for the development of late effects. In general, younger age at treatment is associated with a higher risk and/or greater severity for late effects. Children treated with cranial irradiation during the critical period of rapid brain development (<2 years old) are at greater risk for neurocognitive late effects than children treated when they are older. In addition, female survivors are at greater risk than males for some late effects, including chemotherapy-associated cardiomyopathy, neurocognitive impairment, and second cancers, although the explanation for this gender difference is not clear.
Underlying genetic predisposition for cancer (contributing etiologically to the primary cancer occurrence) will influence the risk for secondary cancers, as has been observed in hereditary retinoblastoma and nevoid basal cell carcinoma syndrome. In some patients, the only marker of this predisposition will be family history or a feature of the primary tumor itself (young age at occurrence, occurrence in paired organs). Predisposition to late effects based on genetic background is an area of great interest in survivorship research. Those survivors with a genetic predisposition to chronic medical conditions, such as heart disease, may be at greater risk for treatment-associated chronic conditions, such as radiation-associated coronary artery disease. Recent genome-wide association studies have identified risk-specific polymorphisms that may become clinically relevant for predicting the risk for late effects and allow for potential tailoring of primary cancer therapy or follow-up. Most associations that have been described—for example, for ototoxicity after cisplatin exposure or second cancer after irradiation —await further validation. In addition, common medical comorbidities associated with aging, such as hypertension or hypercholesterolemia, may contribute to a survivor’s risk for a variety of medical late effects, including chronic renal disease, heart disease, and cerebrovascular disease. Modifiable health behaviors such as diet, exercise, smoking, and sun exposure may also modulate a survivor’s risk for late effects.
High rates of chronic health conditions have been described in adult survivors of childhood cancer. In the CCSS cohort, the relative risk for a severe or life-threatening chronic condition in survivors when compared with siblings was 8.2 (95% confidence interval [CI], 6.9 to 9.7). In the St. Jude Lifetime Cohort Study, the cumulative prevalence of a chronic condition was 95.5% (9%% CI, 94.8% to 98.6%) by age 45 years and 80.5% (95% CI, 73.0% to 86.6) for a severe or life-threatening or disabling condition. The following section provides an overview of late effects experienced by survivors of childhood cancer. A summary of selected treatment-associated late effects is presented in Table 72-1 .
Treatment | Late Effect | Comments | Reference |
---|---|---|---|
Alkylating agents (busulfan, carmustine, lomustine, mechlorethamine, procarbazine, cyclophosphamide, ifosfamide, dacarbazine, melphalan) | Secondary leukemia/ myelodysplasia | Melphalan and mechlorethamine more leukemogenic than cyclophosphamide Median latency 4-6 years Dose-response relationship | |
Premature menopause | Risk factors: increasing cumulative total dose, concurrent pelvic radiation, increasing age, older age at treatment Myeloablative doses are associated with ovarian failure. Cryopreservation of oocytes or embryos are interventions to preserve fertility. | ||
Male infertility | Risk of azoospermia increases with increasing cumulative dose; spermatogenesis may recover over time. Highest dose is associated with impaired testosterone production. Pretreatment semen cryopreservation and testicular sperm extraction are interventions to preserve fertility. | ||
Anthracyclines (daunorubicin, doxorubicin, mitoxantrone, idarubicin) | Clinical heart failure | Risk factors: female gender, age <5 yr, cumulative doxorubicin equivalent dose >250-300 mg/m 2 , concurrent radiation | |
Bleomycin, carmustine, busulfan (pulmonary toxic chemotherapies) | Pulmonary fibrosis | No plateau in incidence observed Radiation exposure an additional risk factor | |
Platinum compounds (cisplatin, carboplatin) | High-frequency hearing loss | Cumulative dose cisplatin >450 mg/m 2 Risk factors: dose, age <5 yr Carboplatin ototoxic at myeloablative dose | |
Speech frequency hearing loss | Cumulative dose cisplatin >720 mg/m 2 Rarely observed cumulative dose cisplatin <360 mg/m 2 Risk factors: dose, age <5 yr Carboplatin ototoxic at myeloablative dose | ||
Topoisomerase II inhibitors (epipodophyllotoxins and anthracyclines) | Secondary leukemia | Median latency 1-3 yr Frequent administration increases risk (weekly/twice weekly). | |
Any irradiation | Secondary sarcoma | Risk factors: increasing radiation dose, concurrent alkylating agent or anthracycline chemotherapy, young age at diagnosis, primary diagnosis of sarcoma or retinoblastoma, family history of sarcoma | |
Nonmelanomatous skin cancer | Fifty percent of survivors will have multiple lesions. Basal cell carcinoma—linear dose-response | ||
Melanoma | Risk factors: radiation dose >15 Gy, prior history melanoma, retinoblastoma, familial melanoma | ||
Cranial and head/neck irradiation (including TBI) | Growth hormone deficiency | High prevalence (85%-100%) with median dose 45-55 Gy Observed at 18-24 Gy, with lower prevalence and longer latency | |
Obesity | Risk factors: ≥20 Gy cranial radiation, female gender | ||
Hypothyroidism | Primary—direct neck radiation ≥10 Gy Central hypothyroidism—cranial dose >35 Gy Dose-response relationship with no plateau in incidence observed after neck radiation | ||
Thyroid cancer | Risk factors: radiation dose 15-30 Gy, young age at treatment, female gender | ||
Brain tumors | The most common pathologic processes are meningioma and glioma. Risk increases with increasing dose. | ||
Head and neck carcinoma | Parotid gland carcinoma most common; secondary head and neck carcinoma Risk of salivary gland carcinoma increases linearly with dose. | ||
Stroke | Mean cranial radiation dose ≥30 Gy increased risk for both leukemia and brain tumor survivors. Highest risk after cranial dose ≥50 Gy | ||
Dental abnormalities | Risk factors: age at diagnosis <5 yr, higher-dose radiation | ||
Chest irradiation (chest, mantle, lung, mediastinal, TBI) | Coronary artery disease | Increased risk with high-dose exposure | |
Cardiac valve disease | Increased risk with high-dose exposure | ||
Breast cancer | Risk factors: increasing radiation dose, age 10-30 years at diagnosis Latency: 10-15 years Ovarian failure reduces risk. | ||
Lung fibrosis | Pulmonary—toxic chemotherapy an additional risk factor | ||
Lung cancer | Greatest risk in Hodgkin lymphoma survivors Increased risk in smokers | ||
Thyroid cancer | Risk factors: radiation dose 15-30 Gy, young age at treatment, female gender | ||
Hypothyroidism | Risk factors: increasing radiation dose, older age at diagnosis, female gender No plateau in incidence observed over time | ||
Abdominal irradiation (abdominal, periaortic, flank) | Gastrointestinal cancer | Risk factors: dose >30 Gy; concurrent treatment with alkylating agents; increasing radiation dose and increasing radiation volume | |
Pelvic irradiation (pelvic, inverted “Y,” TBI) | Genitourinary cancer | Modestly increased risk overall; greatest risk for cancers of the female genital tract, bladder cancer, and renal cell carcinoma Risk factors: renal cell carcinoma renal irradiation, platinum-based chemotherapy, primary diagnosis of neuroblastoma | |
Premature menopause | Risk factors: dose (≥1 to ≤20 Gy) and concurrent alkylating agent chemotherapy Pelvic radiation >10 Gy associated with ovarian failure Cryopreservation of oocytes or embryos is intervention to preserve fertility. | ||
Male hypogonadism | Risk of irreversible azoospermia >2 Gy Higher dose associated with impaired testosterone production, >20 Gy Pretreatment semen cryopreservation and testicular sperm extraction are interventions to preserve fertility. |
Second Cancers
Second cancers are the leading cause of treatment-related mortality in 5-year survivors. In the CCSS cohort, second cancers accounted for 13% of deaths overall and for 60% of deaths defined as treatment related. Most often, a new cancer diagnosed in a survivor can be associated with a prior treatment exposure, such as radiation therapy or alkylating agent chemotherapy. Genetic cancer predisposition may also contribute to a survivor’s risk for subsequent cancers. In addition, survivors, like all aging individuals, develop cancers that are not related to prior treatment or known genetic predisposition and these cancers will become more prevalent as the childhood cancer survivor population ages. To date, there are no data that address the comparative morbidity and mortality from these adult-onset cancers and whether survivors will be compromised in their ability to receive or respond to known effective therapies.
Overall, the likelihood of a childhood cancer survivor being diagnosed with a second primary cancer is three to six times greater than the risk in the aged-matched general population. At 20 years after diagnosis, the cumulative incidence of second cancers in childhood cancer survivors is estimated at 3.2% to 4.2%. In the British Survivorship Cohort, 5% of survivors had developed a secondary cancer by 38 years of age; this cumulative incidence of cancer would be expected at age 54 in a comparable, nonsurvivor population. Clinical and demographic factors that increase the risk for second cancers include primary diagnosis, exposure to radiation, attained age, and chemotherapy with alkylating agents or epipodophyllotoxins. Diagnoses that confer the greatest risk for subsequent cancers include Hodgkin lymphoma (standardized incidence ratio [SIR], 8.7), Ewing sarcoma (SIR, 8.5), and retinoblastoma (relative rate [RR], 15).
Genetic predisposition for second cancers results from an inherited or spontaneous germline mutation in a known or presumed cancer susceptibility gene. Characteristic findings in family cancer predisposition syndromes are younger age at cancer diagnosis, cancer in two or more generations, two or more cancers in an individual, and rare forms of cancer in combination. An example of a rare cancer predisposition syndrome that is associated with pediatric malignancies is Li-Fraumeni syndrome, which is associated with germline mutations in the tumor suppressor gene TP53. It is inherited as an autosomal dominant trait and has a high penetrance with cancer risk of 50% by age 40 years. The cancers most commonly observed in Li-Fraumeni syndrome are soft tissue sarcomas, breast cancer, leukemia, osteosarcoma, adrenocortical cancer, and brain tumors.
Survivors with an underlying genetic predisposition for cancer are more susceptible to treatment-associated secondary malignancies. This is demonstrated in survivors of retinoblastoma who carry a mutation in the RB1 gene. Those with hereditary retinoblastoma treated with irradiation for their primary cancer have a cumulative risk for second cancers of 58% at 50 years, compared with 26% if not treated with irradiation. There are other rare genetic syndromes that are associated with an increased susceptibility to pediatric cancers and subsequent adult-onset secondary cancers, and increasing evidence suggests that a substantial percentage of children with cancer may have an underlying cancer predisposition syndrome that might inform risk for subsequent cancers. Genome-wide association studies of patients with and without secondary cancers may reveal polymorphisms associated with increased risk; for example, a study of Hodgkin lymphoma survivors who received irradiation identified two variants at genetic locus 6q21 that are associated with increased risk for a subsequent cancer. Further work in this field may determine which survivors should undergo more intensive surveillance or prophylaxis for secondary cancer. All survivors of childhood cancer should be assessed for risk for secondary cancer based on a possible cancer predisposition, with attention to the family history as it evolves, development of benign disorders (e.g., polyps or hamartomatous lesions), as well as assessment of the pediatric history, including the primary diagnosis and age at diagnosis. Referral for genetic counseling for survivors of childhood cancer is increasingly important, because recent studies suggest that early genetic diagnosis and subsequent tumor surveillance may result in early diagnosis of secondary cancers in patients with known cancer predisposition syndromes ( Box 72-2 ).
Family History
- •
Cancer in first-degree relatives
- •
Relatives with cancer at early age
- •
Cancer in two or more generations
- •
Individual with two or more cancers
- •
Rare cancers in combination
- •
Cancer in paired organs
Primary Childhood Cancer Diagnosis
- •
Adrenal cortical carcinoma
- •
Choroid plexus carcinoma
- •
Melanoma
- •
Pleuropulmonary blastoma
- •
Renal cell carcinoma
- •
Retinoblastoma
- •
Rhabdoid tumor
- •
Sarcoma
Medical History
Immunodeficiency Syndrome
- •
X-linked lymphoproliferative syndrome
- •
Wiskott-Aldrich syndrome
Genetic Syndrome
- •
Beckwith-Weidemann syndrome
- •
WAGR/Denys-Drash syndrome
- •
Hereditary leiomyomatosis and renal cell carcinoma
- •
Familial adenomatous polyposis
- •
Juvenile polyposis syndrome
- •
Peutz-Jeghers syndrome
- •
Cowden syndrome
- •
Bannayan-Riley-Ruvalcaba syndrome
- •
Von Hippel-Lindau disease
- •
Hereditary paraganglioma–pheochromocytoma
- •
Nevoid basal cell carcinoma
- •
Noonan syndrome
- •
Blooms syndrome
- •
Diamond-Blackfan anemia
- •
Fanconi anemia
- •
Ataxia-telangiectasia
- •
Neurofibromatosis
- •
Tuberous sclerosis
- •
Carney complex
- •
Xeroderma pigmentosum
- •
Turcot syndrome
- •
Rubenstein-Taybi syndrome
- •
Simpson-Golabi-Behmel syndrome
- •
Costello syndrome
Known Carrier of Cancer Predisposition Gene Mutation
- •
ATM
- •
TP53
- •
APC
- •
RB1
- •
WT1
- •
PTCH
- •
MLH1, MLH2
- •
BRCA1, BRCA2
- •
NF1, NF2
- •
MEN1, MEN2
- •
DICER-1
- •
PTEN
- •
VHL
Physical Findings
- •
Dysmorphic features
- •
Skin lesions
- •
Hemihypertrophy
Secondary Solid Tumors
Cancer that develops in a prior radiation field is the most common type of second cancer observed in survivors. Risk estimates for second cancers have been reported from populations of survivors treated with irradiation during historic treatment eras when the modes of radiation therapy routinely used in clinical practice were different than current practices. In current oncology practice, orthovoltage radiation has been eliminated, thus reducing radiation doses to nontumor tissues such as skin, bones, and soft tissue. Large-dose extended-field radiation therapy is increasingly being replaced by involved-field lower-dose radiation therapy or conformal radiation therapy, often in combination with chemotherapy. It is assumed that this change in clinical practice aimed at reducing exposure to radiation will result in a lower incidence of radiogenic cancer; however, no “safe” dose of therapeutic radiation has been established and irradiation-associated cancers have been observed at what would be considered the lowest treatment doses. In general, risk factors for irradiation-associated second cancers include treatment with radiation doses greater than 30 Gy, radiation in combination with alkylating agent chemotherapy, young age at exposure, more than 10 years beyond treatment, and the presence of a cancer predisposition syndrome. Of particular concern is the observation that the cumulative incidence of irradiation-associated cancers does not plateau in groups of survivors followed for up to 50 years after their therapy for childhood cancer. Second cancer risk is modulated by lifestyle choices; for example, adult survivors of Hodgkin lymphoma who smoke after exposure to chest irradiation have a 20-fold greater risk for secondary lung cancer compared with survivors who are nonsmokers.
All tissues and organs are vulnerable to cancer induction by exposure to radiation. However, some tissues, such as thyroid and breast tissue, have greater sensitivity to radiation. Excluding low-grade nonmelanomatous skin cancer and (benign) meningiomas, the most frequently reported irradiation-associated second cancers in 5-year survivors of childhood cancer followed in the CCSS are, in order, breast cancer, thyroid cancer, CNS cancers, soft tissue sarcomas, and bone sarcomas. Additional irradiation-associated second cancers reported in survivors, especially with longer follow-up intervals, include carcinomas of the head and neck (primarily parotid gland), colon cancer and other gastrointestinal cancers, lung cancer, and carcinomas of the genitourinary tract. The most common second cancers observed in childhood cancer survivors are described next.
Breast Cancer
Breast cancer is one of the most frequently diagnosed irradiation-associated second cancers in childhood cancer survivors. Women treated with mantle, mediastinal, chest, spinal, whole-lung, and total-body irradiation during childhood or adolescents are at risk for secondary breast cancer. Large cohort studies of childhood Hodgkin lymphoma survivors report a fifteen- to thirtyfold increased risk for irradiation-associated secondary breast cancer compared with the age-matched general population. Risk estimates vary by characteristics of the cohort, including radiation dose, age at therapy, and duration of follow-up. The cumulative incidence of secondary breast cancer is as high as 12% to 35% at 20 years after therapy for Hodgkin lymphoma, and the incidence does not plateau with longer duration of follow-up.
Female survivors at greatest risk for secondary breast cancer are those treated between the age of 10 and 30 years with chest radiation doses greater than 20 Gy. Studies have demonstrated a linear dose-response relationship between radiation dose and risk for secondary breast cancer. The typical latency of irradiation-associated breast cancer is 10 to 15 years after therapy; however, secondary breast cancer has been reported in women as young as 20 years of age and as soon as 4 years after primary diagnosis. Secondary breast cancer is most often associated with chest irradiation for Hodgkin lymphoma; however, it is important to recognize that women treated with chest irradiation for any childhood cancer diagnosis have increased risk for subsequent breast cancer, as illustrated in Figure 72-4 . Survivors of primary sarcomas not treated with chest irradiation also have an increased risk for secondary breast cancer (RR, 7). Interestingly, survivors with therapy-induced ovarian dysfunction have a relative decreased risk for irradiation-associated breast cancers compared with survivors with normal ovarian function. Although very rare, sporadic cases of male breast cancer have been reported in men treated with whole-lung irradiation as children.

The most common type of breast cancer diagnosed in survivors is invasive ductal carcinoma; adverse biologic features (e.g., hormone-receptor negativity) may be more common in these childhood cancer survivors than in the general population. Similar to findings in the general population, secondary breast cancer is detectable by radiographic imaging and has an excellent prognosis when treated in the earliest stages. Breast cancer treatment options for survivors, however, can be limited by the therapy received for their childhood cancer. Specifically, prior anthracycline exposure and concern for potential cardiotoxicity may limit the use of adjuvant systemic chemotherapy with doxorubicin and the concern for tissue necrosis with repeated exposure to chest irradiation limits the option of breast-conserving surgery for some women.
Current screening recommendations for childhood cancer survivors treated with chest radiation published by the Children’s Oncology Group include monthly self-breast examination, annual clinical breast examination, and annual breast magnetic resonance imaging (MRI) and mammography starting 8 years after treatment or age 25 whichever comes last. Guidelines published by the American Cancer Society also advocate mammography and annual breast MRI, but suggest MRI only for women treated with chest irradiation between the ages of 10 and 30 years. The addition of MRI to mammography has been reported to increase the sensitivity of breast cancer screening, although an impact on breast cancer outcomes has not been described ( Table 72-2 ).
Treatment Risk Factors | Clinical Evaluation | Special Considerations | |
---|---|---|---|
Breast Cancer | Any chest irradiation Spinal irradiation TBI | Monthly breast self-examination Biannual clinical breast examination Breast MRI Mammogram | Current recommendations are to begin annual radiologic screening 8 years after irradiation or age 25 years, whichever occurs last. |
CNS Tumor | Cranial irradiation Head /neck irradiation TBI | Annual neurologic examination Head MRI | Imaging studies are indicated for new or persistent neurologic symptoms. |
Colorectal Cancer | Any abdominal irradiation Pelvic irradiation Spinal irradiation TBI | Colonoscopy Computed tomographic colonoscopy (CTC) | Current recommendations are to begin colonoscopy 10 years after radiation or age 35, whichever occurs last. CTC is not universally recommended as an alternative to colonoscopy in high-risk populations. |
Thyroid Cancer | Neck irradiation Chest irradiation TBI MIBG | Annual clinical thyroid examination Thyroid ultrasound evaluation | Routine ultrasound screening is not currently recommended but should be considered for survivors with difficult clinical examination. |
Skin Cancer | Any irradiation | Annual skin cancer screening Monthly skin self-examination | Practicing skin cancer prevention has potential to reduce risk. |
Thyroid Cancer
Irradiation of the head and neck and upper thorax, total-body irradiation, and treatment with radioactive iodine (e.g., with 131 I-labeled-metaiodobenzylguanidine [MIBG]) place childhood cancer survivors at risk for subsequent thyroid cancers. Large cohort studies of childhood cancer survivors estimate relative risks for secondary thyroid cancer of between 4 and 36, with a cumulative incidence of 1.3% at 30 years reported for female survivors and 4.4% in Hodgkin disease survivors. Well-differentiated papillary carcinoma is the most common pathologic process (63% to 80%); however, follicular carcinomas are also observed. The minimum observed latency period for secondary thyroid carcinoma is 5 years, although cases have been reported closer to the time of original diagnosis. At 20 years of follow-up, the relative risk for thyroid carcinoma remains increased and a plateau in incidence has not been observed beyond 40 years of follow-up. The risk for thyroid cancer is related to radiation dose; but unlike most other radiation-induced second cancers, the risk for thyroid cancer is greatest at lower doses of 15 to 30 Gy and beyond 30 Gy there is a decline in risk. This finding demonstrates that thyroid tissue has enhanced sensitivity to radiation toxicity and supports the “cell kill” hypothesis that suggests higher doses of radiation cause cell death, which reduces the initiation of carcinogenesis observed at lower doses. Although there is conflicting evidence, chemotherapy does appear to modify risk for irradiation-associated secondary thyroid cancer when the radiation dose is less than 20 Gy. Female gender and young age at treatment are independent risk factors for secondary thyroid cancer. Survivors of neuroblastoma treated with 131 I-MIBG are also at risk for subsequent thyroid cancer. In a single-institution case series of 16 neuroblastoma survivors treated with 131 I-MIBG and no external-beam irradiation, followed for a median of 15 years, 9 of 16 had nonpalpable thyroid nodules detected on thyroid ultrasonography, 6 of the 9 went on to biopsy, and 2 of those 6 were diagnosed with papillary thyroid carcinoma.
Radiation-induced thyroid cancer in young survivors has an excellent prognosis; therefore many experts have recommended clinical screening. Appropriate screening schedules for secondary thyroid cancers and recommended modality for screening are controversial. At a minimum, annual palpation of the thyroid for nodules by an experienced examiner is recommended. Routine screening to detect subclinical thyroid nodules with high-resolution ultrasonography has been recommended by some investigators but remains controversial. Ultrasound screening has the benefit of detecting smaller nonpalpable nodules but has the consequence of false-positive findings and potential for unnecessary biopsies. Thyroid nodules do have ultrasound characteristics that are of concern for malignancy (hypoechogenicity, irregular margins, microcalcification). However, these findings are not diagnostic and fine-needle aspiration of nodules larger than 1.0 cm or with suspicious features is usually necessary to evaluate for malignancy. Serial ultrasonography is then recommended to monitor thyroid nodules that are small or benign on biopsy. Although clinical practice is moving toward using ultrasound to screen asymptomatic high-risk survivors for thyroid cancer, screening should be considered only after a discussion of the potential risks and benefits (see Table 72-2 ).
In general, irradiation-associated thyroid cancer is treated the same as other thyroid cancers and has an excellent prognosis. Thyroid surgery is indicated for nodules that are enlarging, are of indeterminate pathology on fine-needle aspiration, or are positive on fine-needle aspiration for malignancy. Total thyroidectomy is often recommended to survivors because irradiation-associated thyroid cancers can be multicentric and because benign nodules can accompany clinically undetectable malignant lesions. Finally, there is indirect evidence to support that exogenous thyroid hormone use reduces the risk for secondary thyroid cancer by suppressing thyroid stimulating hormone (TSH)-induced proliferation of thyroid nodules. Although not proven to reduce risk for thyroid cancer, the use of exogenous thyroid hormone to suppress thyroid proliferation is supported by data that show a decrease in incidence of new thyroid nodules in patients treated with exogenous thyroid hormone after partial thyroidectomy (34% vs. 14%).
Sarcomas
Survivors treated with radiation are at increased risk for secondary cancer of the bone and soft tissue in the radiation field. Although secondary sarcomas are most often associated with irradiation, survivors of primary sarcoma and hereditary retinoblastoma who have not had prior radiation therapy also have an increased risk, suggesting a genetic predisposition. Risk estimates for secondary sarcoma vary by type of sarcoma and composition of the study cohort. The CCSS cohort, which did not include survivors of retinoblastoma, reports an overall ninefold increased risk for secondary sarcomas and an absolute excess risk of 32.5 per 100,000 person-years. Secondary sarcomas occurring after childhood cancer include multiple histologic subtypes such as osteosarcoma, nonrhabdomyosarcoma soft tissue sarcomas, primitive neuroectodermal tumors (Ewing sarcoma), and malignant peripheral nerve sheath tumors, as well as rarer sarcoma subtypes. The median latency for secondary sarcomas is 11 years, with increased risk persisting beyond 20 years. Reported risk factors for subsequent sarcomas include higher doses of radiation, primary diagnosis of sarcoma or hereditary retinoblastoma, younger age at diagnosis, alkylating agent exposure, anthracycline exposure, and family history of sarcoma. A nested case-control study of secondary sarcomas in the CCSS cohort identified a dose-response relationship between radiation dose and risk for secondary sarcomas, with elevations in risk ratios with higher radiation doses: 10 to 29.9 Gy (odds ratio [OR], 15.6; 95% CI, 4.5 to 53.9), 30 to 49.9 Gy (OR, 16.0; 95% CI, 3.8 to 67.8), and more than 50 Gy (OR, 114.1; 95% CI, 13.5 to 964.8). Risk factors other than irradiation associated with increases in risk for secondary sarcoma in this study included primary cancer diagnosis of sarcoma or Hodgkin lymphoma and anthracycline exposure.
For survivors of hereditary retinoblastoma treated with irradiation, the risk for subsequent bone sarcomas is as high as 400-fold with a cumulative incidence at 20 years of 14.1% and the relative risk for soft tissue sarcomas is 140 with 50-year cumulative incidence of 13%. Radiation exposure and underlying genetic predisposition both contribute to risk for secondary sarcomas in retinoblastoma survivors.
Routine radiographic screening for secondary sarcomas is currently not recommended. Survivors at risk for secondary sarcomas should be counseled to report new pain, lesions, or masses in the radiation field and should be promptly evaluated for any signs or symptoms suggestive of a secondary sarcoma. As survivors enter follow-up care, providers should consider obtaining baseline imaging of any previously irradiated site, so these studies are then available for comparison should symptoms suggestive of a new malignancy develop. There is no established role for secondary sarcoma surveillance for survivors of hereditary sarcoma predisposition syndromes (Li-Fraumeni syndrome, hereditary retinoblastoma), although the role of whole-body MRI is currently under investigation.
Skin Cancer
Melanoma and nonmelanomatous skin cancers are reported with increased incidence in survivors. The relative risk for melanoma is 2.5 to 9.0 in childhood cancer survivors, with a median latency of 15 to 18 years. Survivors of hereditary retinoblastoma, familial melanoma syndromes, and those with a prior history of melanoma all have an increased risk for subsequent melanoma independent of irradiation. Although the association between therapeutic radiation and melanoma is not firmly established, a case-control study of 16 survivors with secondary melanoma in a European cohort of 4000 childhood cancer survivors found an association between secondary melanoma and exposure to more than 15 Gy of radiation. In contrast, basal cell carcinomas and squamous cell carcinomas are known to be more common in radiation-exposed survivors (RR, 6.3), with 90% of all nonmelanomatous skin cancers diagnosed in previously irradiated fields. A linear dose-response relationship is described for basal cell carcinomas, with an excess odds ratio of 1.09 per Gy of radiation. Also of note, these low-grade skin cancers can be recurrent, and almost half of survivors diagnosed will have multiple lesions. The prognosis for secondary skin cancers is excellent with early diagnosis; therefore routine screening with skin examinations is recommended. Because ultraviolet radiation from the sun is known to contribute to skin cancer risk; patients should be routinely educated about preventive strategies such as aggressive sun exposure protection (see Table 72-2 ).
Central Nervous System Tumors
Past exposure to cranial irradiation is considered the most important risk factor for the development of subsequent CNS tumors in survivors of childhood cancer. The most common pathologic processes include meningioma ( Fig. 72-5 ) and glioma; however, other tumors such as primitive neuroectodermal tumor or medulloblastoma have been reported. In a report from the British Childhood Cancer Survivor Study, the standardized incidence ratio for development of a subsequent glioma was 10.8 (95% CI, 8.5 to 13.6), and in the CCSS cohort it was 8.7 (95% CI, 6.2 to 11.6). Higher standardized incidence ratios have been reported for meningioma, and meningioma is the most common subsequent CNS tumor 10 years or more after a childhood cancer diagnosis. There is an established dose-response relationship between cranial radiation dose and incidence of secondary CNS tumors. In the CCSS cohort, Neglia and colleagues reported an excess relative risk of 1.06 per Gy of cranial irradiation for meningiomas and 0.3 per Gy for gliomas. In the British Childhood Cancer Survivor Study cohort, Taylor and colleagues reported that the risk for glioma and for meningioma each increased linearly with increasing radiation dose. Several studies have suggested that the increased risk for development of meningioma does not appear to plateau. In a report from the CCSS, among survivors with no previous diagnosis of meningioma at 25 years after a primary CNS tumor, the cumulative incidence of meningioma at 30 years was 3.5% (95% CI, 0.9% to 6.1%). The contribution of chemotherapy to the risk for CNS malignancies is controversial. Laboratory investigations have suggested an association between radiation-induced brain tumors and thiopurine-based chemotherapy related to a deficiency in the enzyme thiopurine methyltransferase (TPMT). In the British Childhood Cancer Survivor Study cohort, a higher cumulative dose of intrathecal methotrexate was associated with the development of meningioma. In the CCSS cohort, after adjusting for radiation dose, chemotherapy exposure did not alter the survivor’s secondary brain tumor risk.

The role of routine surveillance for secondary CNS tumors with neuroimaging in asymptomatic childhood cancer survivors requires further investigation. Single-institution series of neuroimaging screening have reported high rates of meningioma. For example, in a prospective study of 76 survivors of acute lymphoblastic leukemia that was treated with cranial irradiation and who were screened with MRI every 3 to 6 years for 20 years, Goshen and colleagues detected 16 meningiomas (15 asymptomatic) and one glioma. Cumulative incidence in this screened population was 14.8% at 20 years. Surgical resection of these secondary CNS tumors was recommended for 12 of the 16 survivors, with one surgical complication reported. Whether early detection of meningiomas in asymptomatic survivors might reduce morbidity and minimize neurologic complications by facilitating surgical intervention remains to be determined. Survivors with a prior history of cranial irradiation should be educated about the potential for late-occurring CNS tumors and instructed to report new or persistent neurologic signs or symptoms (see Table 72-2 ).
Other Cancers
Secondary carcinomas of the gastrointestinal tract, genitourinary organs, lung, and head and neck region are observed in survivors of childhood cancer and are associated with radiation exposure and longer follow-up times. In general, irradiation-associated carcinomas (like colon cancers) occur at an earlier age than is seen in the general population, thereby supporting recommendations for early initiation of cancer screening and aggressive evaluation of symptoms suggestive of possible secondary malignancy.
Large cohorts of childhood cancer survivors report relative risks for gastrointestinal cancers between 4.6 and 7.0. A European cooperative cohort study found the risk for secondary gastrointestinal malignancies greatest after abdominal radiation dose greater than 30 Gy (SIR, 9.7); however, lower-dose radiation was also associated with increased risk (10 to 29 Gy, SIR 5.2). Alkylating agent chemotherapy is consistently found to be independently associated with an increased risk for gastrointestinal cancer. The most common irradiation-associated secondary gastrointestinal malignancy is colorectal cancer, with a standardized incidence ratio of 3.6 to 13 and as high as 63.4 (95% CI, 15.7 to 71.8) reported in a cohort of Hodgkin lymphoma survivors. In a British cohort of 18,000 childhood cancer survivors, the 50-year cumulative incidence of colorectal cancer for those treated with direct abdominal radiation was 1.4% (95% CI, 0.7 to 2.6). The St. Jude Children’s Research Hospital cohort of over 13,000 childhood cancer survivors found a 40-year cumulative incidence of secondary colorectal cancer of 1.4% ± 0.53%). In this study, the risk for colorectal cancer increased by 70% with each 10-Gy increase in radiation dose and increasing radiation volume also significantly increased risk. Current recommendations for screening of survivors treated with abdominal radiation therapy are to begin colon cancer screening earlier than what is recommended for the general population (10 years after completing radiation therapy or age 35 years, whichever comes last), although the benefit of early surveillance has not be established in this high-risk population (see Table 72-2 ).
Of note, gastric cancers and esophageal cancers are also observed in greater numbers than expected for age in survivors of pediatric Hodgkin lymphoma. Common symptoms such as dysphagia and dyspepsia should be evaluated aggressively, with a low threshold for utilizing endoscopy or imaging to rule out malignant or premalignant conditions in exposed survivors.
Overall, the relative risk for genitourinary cancer is modestly elevated (SIR, 1.9; 95% CI, 1.6 to 2.4), with elevated relative risks for secondary malignancies of the female genital tract (SIR, 2.1; 95% CI, 1.4 to 3.2), secondary renal cell carcinoma (SIR, 8.0; 95% CI, 5.2 to 11.7), and secondary bladder cancer (SIR, 5.1; 95% CI, 2.1 to 12.4). Data from the CCSS show the risks for secondary renal carcinoma are primary diagnosis of neuroblastoma (SIR, 85.8; 95% CI, 38.4% to 175.2%), renal-directed radiation therapy of 5 Gy or greater (RR, 3.8; 95% CI, 1.6 to 9.3), and platinum-based chemotherapy (RR, 3.5; 95% CI, 1.0 to 11.2). Secondary urinary bladder cancer is associated with both radiation therapy and cyclophosphamide chemotherapy, with the risk dependent on dose and duration of therapy. An adult series of more than 6000 non-Hodgkin lymphoma survivors treated with cyclophosphamide reported 31 cases of bladder cancer (RR, 4), and no significantly increased risk was observed at doses less than 20 g/m 2 . In a British cohort of 18,000 childhood cancer survivors, 17 were diagnosed with bladder cancer (RR, 4); risk factors were diagnosis of hereditary retinoblastoma, treatment with chemotherapy, male gender, and longer follow-up. In another series of adults treated with prolonged cyclophosphamide for nonmalignant disease, 5% developed secondary bladder cancer at a median of 8.5 years and all diagnoses were preceded by symptoms of microscopic hematuria. Although the absolute excess risk for bladder cancer in childhood cancer survivors is low, routine monitoring with urinalysis of survivors exposed to pelvic radiation, large cumulative dose of cyclophosphamide, and those with hereditary retinoblastoma should be considered.
Cancer of lung and bronchus is diagnosed more commonly in childhood cancer survivors than the general population (SIR, 3.4; 95% CI, 1.9 to 6.1), with greater risk observed in cohorts of pediatric Hodgkin lymphoma survivors (SIR, 5.1 to 27.2). In a meta-analysis of secondary lung cancer in Hodgkin lymphoma survivors, the largest risk for secondary lung cancer was observed among patients diagnosed age 15 to 24 years (RR, 8.76; 95% CI, 4.55 to 16.89). Treatment with alkylating agents and irradiation are both associated with increased lung cancer risk. In addition, studies of adult survivors of Hodgkin lymphoma have shown that smoking increased lung cancer risk more than twentyfold. Aggressive smoking cessation interventions and routine surveillance with radiographic imaging have been suggested for this high-risk population of survivors, although the use of spiral computed tomography has not been studied in the survivors who had chest irradiation.
Survivors are also at increased risk for secondary head and neck carcinomas (SIR, 10.8 to 14.1), with mucoepidermoid carcinoma of the parotid gland the most frequently observed diagnosis. In a CCSS analysis of secondary salivary gland carcinomas, the incidence was thirty-ninefold higher in survivors compared with the general population (95% CI, 25.4 to 57.8). The analysis also demonstrated that risk increased linearly with radiation dose (excess RR, 0.36/Gy; 95% CI, 0.06 to 2.5). Interestingly, modifiable health behaviors including cigarette smoking and alcohol intake were not associated with increased risk for head and neck cancers in childhood cancer survivors.
Hematologic Malignancies
Childhood cancer survivors are at an increased risk for developing subsequent hematologic malignancies, particularly secondary acute myelogenous leukemia. Overall, the relative risk for secondary acute leukemia in childhood cancer survivors is reported from 7 to 20. An increased risk for secondary leukemia is described after treatment of a primary solid tumor and after treatment of acute lymphoblastic leukemia, particularly with exposure to a topoisomerase II inhibitor. In an analysis of survivors of cancer diagnosed when younger than 20 years of age, utilizing the Surveillance, Epidemiology, and End Results (SEER)-9 database, the cumulative incidence of secondary hematologic malignancy at 5 years was 0.2% (± 0.03%) and at 10 years it was 0.4% (± 0.04). In contrast to irradiation-associated malignancies, the latency for leukemogenesis associated with chemotherapy is within the first 10 years after treatment, with the greatest risk observed in the first 5 years off therapy. In a study of 4202 survivors of childhood solid tumors, the relative risk of leukemia was 20 at 3 to 5 years and then decreased to 2.2 by 10 years off therapy. Of interest, in this same study a second peak in secondary leukemia was observed at 20 years or more of follow-up (RR, 14.8); this finding has not been observed in studies with shorter follow-up periods.
Acute leukemias and myelodysplasia are associated with exposure to high cumulative doses of alkylating agents and topoisomerase II inhibitors, including epipodophyllotoxins and anthracyclines. The alkylating agents presenting the greatest concern for secondary leukemia risk are melphalan and mechlorethamine, when compared with cyclophosphamide and ifosfamide. Secondary leukemias induced by exposure to high-dose alkylating agents are classically considered to be preceded by myelodysplasia, have a median latency of 4 to 6 years, are more often diagnosed in older children, and are often associated with losses or deletions of chromosome 5 or 7. Higher cumulative dose of alkylating agents is associated with a greater risk for the development of secondary acute myelogenous leukemia. Interestingly, more recent pediatric studies demonstrated lower overall rates of leukemia after alkylating agent exposure than earlier studies, likely reflecting the trend of treatment with lower cumulative doses of less leukemogenic alkylating agents in more modern cohorts.
Topoisomerase II–induced leukemias, on the other hand, typically do not have an indolent course, have a relatively short latency (median, 1 to 3 years), are diagnosed in younger children, and are associated with a balanced chromosomal translocation 11q23 and, less frequently, 21q22. Some have reported an increased risk for secondary acute myelogenous leukemia with higher cumulative epipodophyllotoxin dose. For example, in a case-control study of 61 cases of secondary leukemia in childhood survivors, Le Deley and colleagues reported a greater than twentyfold increased risk for leukemia after exposure to greater than 6 g/m 2 of epipodophyllotoxins and a sevenfold increased risk after exposure to either 1.2 to 6 g/m 2 epipodophyllotoxins or greater than 170 mg/m 2 of anthracyclines compared with lower doses of these agents. However, other investigators have not demonstrated a relationship between higher cumulative epipodophyllotoxin dose and the development of secondary acute myelogenous leukemia. Schedule of administration of epipodophyllotoxins may contribute to the risk for secondary leukemia, because prolonged infusions (twice weekly, weekly) have been found to be an independent risk factor for development of leukemia.
The contributory role of therapeutic doses of radiation in the development of secondary leukemia is debated. Although some studies find no association between leukemia and exposure to radiation therapy, others studies have documented subsequent leukemia in survivors after exposure to radiation in the absence of chemotherapy. In addition, specific host factors, such as polymorphisms of DNA repair genes, may contribute to susceptibility to secondary acute myelogenous leukemia.
The prognosis of secondary acute myelogenous leukemia is generally considered to be poor. In the SEER-9 analysis cited earlier, the 5-year overall survival for patients with secondary hematologic malignancies was 31% ± 4.7%. In a report of 24 children with secondary myelodysplastic syndrome or acute myelogenous leukemia, those with secondary myelodysplastic syndrome or acute myelogenous leukemia had lower rates of remission with induction therapy and poorer overall and event-free survival when compared with patients with de novo acute myelogenous leukemia. Further investigation is needed to determine the optimum strategies for preventing and treating secondary leukemia.
Organ System Late Effects
Cardiovascular System
Cardiac disease is the leading cause of treatment-related morbidity and noncancer mortality observed in childhood cancer survivors. In an analysis of late mortality among 5-year survivors of childhood cancer from the CCSS cohort, childhood cancer survivors were seven times more likely to die of cardiac-related events compared with expected population-based rates of cardiac-related deaths. Indeed, childhood cancer survivors have been reported to be at significantly increased risk, compared with a sibling cohort, for the development of congestive heart failure (RR, 15.1; 95% CI, 4.8 to 47.9,), coronary artery disease (RR, 10.4; 95% CI, 4.1 to 25.9), and cerebrovascular accidents (RR, 9.3; 95% CI, 4.1 to 21.2). The treatments most often associated with cardiac morbidity and mortality are anthracycline chemotherapy and cardiac irradiation. A recent cohort study assessing the long-term risk for clinically validated, symptomatic cardiac events in 5-year survivors of childhood cancer demonstrated that higher cumulative anthracycline dose or higher cumulative radiation dose was associated with higher risk ratio for cardiac events. Survivors with both anthracycline and cardiac irradiation exposures had the highest cumulative incidence of cardiac events, with nearly one in eight survivors treated with both exposures developing a serious cardiac event. Risk factors for late onset anthracycline cardiotoxicity (occurring 1 year beyond completion of therapy) have been assessed in multiple studies and include higher cumulative anthracycline dose, history of acute cardiac toxicity, combined exposure with other cardiotoxic therapy, female sex, younger age at exposure (<5 years old), and longer duration of follow-up. Irradiation of the heart as a consequence of irradiation to the chest for treatment of childhood cancer can cause both functional and anatomic cardiac disease. Mantle field, whole-lung fields, left- or whole-abdominal irradiation, spinal irradiation, and total-body irradiation all put a survivor at risk for cardiac late effects. Irradiation-associated cardiac late effects include constrictive pericarditis, myocardial fibrosis, cardiomyopathy, coronary artery disease, valvular abnormalities, conduction disturbances, and vascular disease. Similar to other irradiation-associated late effects, cardiac toxicity is observed more frequently in those survivors treated at the youngest age, receiving higher radiation dose volumes to the heart, and followed the longest.
Nonanthracycline chemotherapeutic agents, including cyclophosphamide, cytarabine, cisplatin, and ifosfamide, have also been associated with cardiotoxicity. In addition, children and adolescents are increasingly being treated with newer agents with unclear cardiac risks. For example, tyrosine kinase inhibitors (imatinib mesylate, sorafenib, and sunitinib) have been associated with cardiac toxicity with exposure in adulthood. Cardiac risks with prolonged exposures to newer agents in large cohorts of pediatric patients are yet to be defined.
The spectrum and nature of cardiac late effects experienced by childhood cancer survivors suggest a need for regular screening with one or more cardiac imaging modalities. Risk-based screening recommendations for childhood cancer survivors exposed to cardiotoxic therapies have been published by the Children’s Oncology Group as part of the “Late Effect Guidelines” as well as by other groups. However, these guidelines have not been tested for efficacy in terms of reduction of cardiac mortality or morbidity. Survivors exposed to cardiotoxic therapy should be informed about their risk for cardiac disease and should also be made aware of activities that present potential stressors to already impaired cardiac function, including smoking, pregnancy, and anesthesia.
Cardiomyopathy
Cardiomyopathies diagnosed in childhood cancer survivors after completion of therapy are associated with both anthracycline chemotherapy and cardiac irradiation. Survivors are at risk for both systolic and diastolic left ventricular dysfunction, which can lead to clinical heart failure. Anthracycline-associated cardiotoxicity can be classified as acute, early onset (<1 year after anthracycline exposure), or late onset (>1 year after anthracycline exposure), although it is not clear to what extent these categories reflect differences in underlying pathophysiology. Anthracyclines are considered to be directly toxic to cardiac myocytes, with exposure resulting in reduced myocyte mass and impaired function of cardiac muscle. Subclinical anthracycline cardiotoxicity is measured as diminished left ventricular contractility and a thinning of the left ventricular wall. Thinning of ventricular walls causes elevated wall stress, which increases afterload, and ultimately can progress to symptomatic left ventricular failure. In children, loss of cardiac myocytes at a young age can result in impaired cardiac growth and function relative to somatic growth, which contributes to the observed decrease in left ventricular systolic function. Anthracycline-associated late cardiac toxicity can manifest as asymptomatic cardiac dysfunction detected by echocardiogram or other modalities or symptomatic dysfunction and the classic signs of heart failure. Longitudinal and long-term follow-up data support that cardiac abnormalities diagnosed in childhood cancer survivors treated with anthracyclines are persistent and progressive. In a Dutch cohort of more than 800 anthracycline-exposed childhood survivors, the cumulative incidence of anthracycline-induced clinical heart failure increased with the duration of follow-up; there was a cumulative incidence of 2.6% at 10 years and 5.5% at 20 years, with no evidence of plateau in incidence with longer duration of follow-up. Of further concern, the incidence of asymptomatic left ventricular dysfunction detected by cardiac screening is reported to be as high as 50% to 60%. However, the long-term clinical implications of subtle echocardiographic abnormalities in asymptomatic individuals are not fully determined.
Many risk factors for anthracycline-associated cardiomyopathy have been identified. The major risk factor for anthracycline cardiomyopathy is cumulative dose (“doxorubicin equivalent”). Equivalent dose takes into consideration exposure to different anthracyclines and can be calculated using conversion factors ( Table 72-3 ). Survivors exposed to cumulative doses of doxorubicin equivalents greater than 250 to 300 mg/m 2 appear to be at greatest risk for cardiomyopathy. The dose-dependent relationship between anthracyclines and subsequent congestive heart failure was demonstrated in the Dutch cohort, where the greatest risk for congestive heart failure was at a dose greater than 300 mg/m 2 (RR, 8), and no congestive heart failure was observed at doses less than 150 mg/m 2 . However, others have shown that survivors treated with low cumulative dose (45 mg/m 2 ) also have asymptomatic cardiac abnormalities detectable on an echocardiogram, suggesting that exposure to anthracycline at any dose poses potential risk for late cardiotoxicity.
Anthracycline | Conversion Factor |
---|---|
Doxorubicin | Total dose (mg/m 2 ) × 1 |
Daunorubicin | Total dose (mg/m 2 ) × 1 |
Mitoxantrone | Total dose (mg/m 2 ) × 4 |
Idarubicin | Total dose (mg/m 2 ) × 5 |
Epirubicin | Total dose (mg/m 2 ) × 0.67 |
* Estimation of anthracycline isotoxic dose equivalents for use in calculation of total cumulative anthracycline dose.
Additional reported risk factors for the development of anthracycline-associated cardiomyopathy include history of acute cardiac toxicity during treatment, combined exposure with other cardiotoxic therapy, female sex, younger age at exposure (<5 years of age), and longer duration of follow-up. Cardiotoxicity diagnosed during or within 1 year of completion of therapy is considered a risk factor for late cardiotoxicity. Furthermore, survivors exposed to anthracycline therapy and concurrent mediastinal radiation are considered to be at risk for cardiomyopathy at lower total anthracycline dose. Increasingly, genetic variants that may predispose to anthracycline cardiotoxicity are being explored. A recent evaluation from the Children’s Oncology Group demonstrated that patients with CBR3 V244M homozygous G genotype with low- to moderate-dose anthracycline exposure were at an increased risk for cardiomyopathy.
Interventions aimed at preventing or reducing long-term anthracycline-associated cardiotoxicity in childhood cancer survivors include limiting anthracycline doses in most therapeutic regimens to less than 300 mg/m 2 , adding cardioprotectants such as dexrazoxane to anthracycline-based regimens, and prescribing afterload-reducing agents to prevent progression of asymptomatic dysfunction to overt heart failure. Data from clinical trials in childhood ALL show that the addition of cardioprotectants did not adversely affect survival. Although an early analysis of a Pediatric Oncology Group clinical trial for Hodgkin lymphoma, which randomized the use of dexrazoxane as a cardioprotectant, demonstrated a possible increase in second cancers with dexrazoxane exposure, a larger cohort of survivors of childhood acute lymphoblastic leukemia treated with dexrazoxane did not demonstrate an increased risk for second cancers. Childhood cancer survivors diagnosed with anthracycline-associated left ventricular dysfunction have been treated with angiotension-converting enzyme inhibitors with the aim of reducing afterload and preventing the progression of left ventricular dysfunction to heart failure. In a longitudinal study of 18 survivors with left ventricular dysfunction, treated with enalapril and followed for a median of 10 years, all initially showed improvement in left ventricular function. The effects, however, were transient, lasting 6 to 10 years, followed by deterioration of left ventricular function over time. However, given results from large studies of medical interventions for patients with asymptomatic left ventricular dysfunction (who have not received anthracyclines), as well as data from adult cancer patients, many cardiologists who evaluate and treat adults with anthracycline-induced cardiomyopathy will intervene with angiotensin-converting enzyme inhibitors. Future investigations should be directed at testing interventions to prevent cardiac injury during therapy and to prevent progression of asymptomatic left ventricular dysfunction to overt cardiac disease in survivors.
Irradiation-associated cardiomyopathy is most often restrictive, with impaired diastolic function, although systolic dysfunction may also be observed. Radiation is believed to induce injury to the myocardial microvasculature, which in turn causes myocardial ischemia, and ultimately myocardial fibrosis, resulting in diastolic dysfunction. Studies have demonstrated a significant incidence of diastolic dysfunction in asymptomatic patients who undergo radiation therapy. In a small cohort of Hodgkin lymphoma survivors treated with a median mediastinal dose of 35 Gy, evaluated at a mean of 9 years after therapy, 20% had evidence of diastolic dysfunction on cardiac imaging. The extent to which these changes are progressive, and whether subclinical dysfunction will result in clinical heart failure over time, is unknown. As noted earlier, there is a significantly increased risk for cardiotoxicity with combined exposure to both anthracyclines and irradiation.
Screening for cardiomyopathy with serial echocardiograms is currently recommended for survivors treated with anthracyclines and/or thoracic radiation therapy, with the frequency of echocardiogram screening depending on the individual survivor’s risk for cardiomyopathy as determined by age at exposure, cumulative treatment dose, and concurrent treatment with thoracic irradiation. The use of newer modalities for screening, such as cardiac MRI, is an area of active investigation. The extent to which screening will result in a reduction in cardiac morbidity and mortality in survivors remains unknown.
Coronary Artery Disease
Survivors treated with thoracic irradiation are at increased risk for the development of radiation-associated coronary artery disease (CAD), which is an established cause of early mortality. Radiation injury to the coronary arteries is considered to be caused by acute endothelial damage followed by subsequent initiation or acceleration of atherosclerosis. Irradiation-associated coronary artery stenosis is most common in the proximal coronary arteries, which are not necessarily shielded during cardiac blocking. In a historic cohort, childhood Hodgkin lymphoma survivors treated with more than 40 Gy of mediastinal radiation had a fortyfold excess risk for fatal myocardial infarction compared with an age-matched general population. A report on 314 Hodgkin lymphoma survivors treated at age younger than 20 years found a fivefold increased risk for myocardial infarction at a median of 19 years after treatment, and a British cohort that included 2158 Hodgkin lymphoma survivors treated at age younger than 25 years found a nineteenfold increased risk for death from myocardial infarction. A recent study assessing the long-term risk for clinically validated, symptomatic cardiac events in 5-year survivors of childhood cancer demonstrated a significantly increased 30-year cause-specific cumulative incidence of cardiac ischemia for survivors treated with cardiac irradiation compared with survivors treated without cardiac irradiation (2.2% [95% CI, 0% to 4.7%] vs. 0.3% [95% CI, 0% to 0.8%], P = .01)]. Modern irradiation techniques are designed to reduce radiation to the coronary arteries, although reduction in irradiation-associated CAD has not yet been proven.
The risk for asymptomatic CAD in survivors is described in many studies; however, optimal screening and interventions to detect and treat irradiation-associated cardiovascular disease have not been determined. Survivors at risk for irradiation-associated CAD are advised to adopt preventive measures commonly used to reduce the risk for atherosclerotic CAD in the general population (aggressive treatment of hypertension, diabetes, obesity, dyslipidemias). Although the efficacy of such measures is not proven in this population, at least one cardiac risk factor was present in all survivors who developed CAD in a single-institution study of 415 Hodgkin lymphoma survivors ; these data suggest that standard cardiovascular risk–reducing strategies might be beneficial to survivors.
Stress echocardiograms can be used as a noninvasive method to detect asymptomatic ischemic heart disease by observing stress-induced wall motion abnormalities. Noninvasive nuclear scintigraphy has also been considered to screen survivors for asymptomatic CAD. A report of screening 294 asymptomatic Hodgkin lymphoma survivors treated at a median age of 25 years with more than 35 Gy found 20% had electrocardiographic findings consistent with prior ischemia and 14% had abnormal stress echocardiograms or stress-induced perfusion defects by nuclear scintography. The survivors with abnormal nuclear perfusion studies who went on to cardiac catheterization were diagnosed with irradiation-induced microvascular damage in the myocardium that was not amendable to surgical intervention. This study showed that the increased sensitivity of adding perfusion scanning in addition to stress echocardiography was at the expense of decreased specificity, with false-positive detection of disease not amendable to intervention. This calls into question the benefit of adding nuclear studies to routine screening of asymptomatic survivors for CAD. The roles of modalities such as CT angiography, MR angiography, and coronary artery calcium scores in the childhood cancer survivor population are yet to be established.
Surgical treatment of CAD in survivors with prior radiation exposure can be complicated by mediastinal fibrosis, myocardial fibrosis, and the coexistence of other irradiation-associated morbidities, including valvular disease, conduction abnormalities, and pulmonary dysfunction. In a recent report from Wu and colleagues, adults with irradiation-associated heart disease undergoing cardiothoracic surgery had significantly higher mortality compared with a matched control population undergoing similar procedures. The high surgical risk in patients with irradiation-associated heart disease should be carefully considered when planning surgical interventions.
Arrhythmias
A full spectrum of cardiac arrhythmias and conduction disturbances have been reported in childhood cancer survivors treated with both irradiation and anthracyclines. These include atrioventricular nodal bradycardia, sick-sinus syndrome, complete and incomplete heart block, prolonged QT interval, and serious ectopy (ventricular tachycardia). In a series of 48 survivors of pediatric Hodgkin lymphoma treated with mediastinal irradiation (median dose, 40 Gy), 75% were found to have conduction abnormalities. The most frequent conduction disturbance was right anterior bundle branch block, the most anterior structure in the conduction system. These arrhythmias are rarely symptomatic; however, the clinical concern is that conduction abnormalities may be progressive. Because conduction abnormalities typically are asymptomatic, they usually are detected in association with other irradiation-induced cardiac abnormalities.
Valvular Disease
The mitral, aortic, and tricuspid valves can all be affected by irradiation-associated fibrous endocardial thickening. Valvular insufficiency is more common than valvular stenosis, but stenosis tends to be more severe, requiring intervention. The frequency of left-sided valvular regurgitation is reported to be 16% to 24% in chest-irradiated survivors of Hodgkin lymphoma screened by echocardiography at a median of 10 years, and valvular dysfunction was not observed at a radiation dose less than 30 Gy. In a cohort of 48 long-term survivors of pediatric Hodgkin lymphoma (median age at diagnosis, 16 years; median dose, 40 Gy), left-sided regurgitation was noted in 36% and valvular defects were detected in 42%. In a recent report, the 30-year cause specific cumulative incidence of symptomatic valvular disease in survivors treated with cardiac irradiation was 2.5% (95% CI, 0% to 5.0%), compared with 0.1% (95% CI, 0% to 0.3%) for those treated without cardiac irradiation. Valvular defects can be progressive, which is of particular concern for young survivors. In a single-institution series of 415 survivors of Hodgkin lymphoma who received mediastinal irradiation, clinically significant valvular dysfunction developed in 6.2% at a median of 22 years. In this cohort, aortic stenosis was the most common clinically significant valve lesion and when results were compared with those of an age-matched general population the relative risk for needing valve surgery was eightfold higher in survivors. The only treatment risk identified for significant valvular disease in this cohort was irradiation technique that resulted in a higher total dose to the heart. Symptoms of valvular heart disease are the same in survivors as the general population, and valve function can be monitored by routine echocardiographic screening.
Pericarditis
The incidence of late-occurring irradiation-associated pericarditis has been significantly reduced by modern irradiation techniques. In historic cohorts of children treated with orthovoltage mediastinal irradiation, 7% to 15% developed symptomatic constrictive pericarditis, compared with 0% to 2.5% of children treated with megavoltage therapy. Although the incidence of pericarditis has been significantly reduced in recent cohorts, older childhood cancer survivors continue to be at risk for pericarditis. Presenting signs and symptoms include pleuritic chest pain, shortness of breath, a friction rub, and electrocardiographic changes. A survivor presenting with symptomatic pericarditis is at risk for progressing to cardiac tamponade. Management includes pericardiocentesis and pericardiectomy, which are high-risk procedures in this patient population with known risks for other irradiation-associated cardiac morbidities.
Cerebrovascular Disease
Survivors treated with irradiation to the head and neck are at risk for the development of clinically significant premature cerebrovascular disease. Irradiation-induced cerebrovascular disease can encompass numerous abnormalities, such as moyamoya disease, vascular malformations including venous-based cavernous malformations (cavernomas) or intracranial aneurysms, and extracranial internal carotid stenosis. Abnormalities such as cavernous malformations may be asymptomatic and noted incidentally on follow-up imaging or may present as neurologic symptoms such seizures, headache, or with hemorrhage, potentially necessitating surgical interventions. Intracranial aneurysms can be life threatening. There are limited long-term data on the risk for late-occurring cerebrovascular disease in survivors of childhood cancer. Data from the CCSS, based on self-report, demonstrated an increased risk for stroke in survivors of brain tumors (RR, 29) and of leukemia (RR, 6.4) when compared with sibling controls, and risk for stroke was associated with a mean cranial irradiation dose greater than or equal to 30 Gy. In addition, among leukemia and brain tumor survivors who did not receive cranial radiation therapy, the risk for stroke remained significantly increased when compared with siblings. CCSS data also demonstrate an increased risk for stroke among survivors of Hodgkin lymphoma (RR, 4.32), with mantle irradiation strongly associated with subsequent stroke. Importantly, in the CCSS cohort, smoking was associated with a threefold excess risk for stroke in survivors of Hodgkin lymphoma. In a study investigating cerebrovascular mortality in a French/United Kingdom cohort of 4227 five-year survivors of childhood cancer, at a median follow-up 29 years after radiation to the brain, the risk for death from cerebrovascular disease increased linearly with local radiation dose to the prepontine cistern. Survivors who received greater than 50 Gy to the prepontine cistern had a notably increased risk for death from cerebrovascular disease (RR, 17.8).
Risk-based surveillance for cerebrovascular disease currently includes careful assessment by history and physical examination for neurologic and neurocognitive signs and symptoms. Neuroimaging is indicated for diagnostic assessment in symptomatic patients. The Children’s Oncology Group Guidelines recommend consideration of baseline Doppler ultrasonography of carotid vessels 10 years after radiation therapy to the neck in those with radiation exposure of more than 40 Gy. In a report on 30 childhood cancer survivors who received neck irradiation, Meeske and colleagues noted an increase in carotid disease on carotid ultrasound assessment compared with healthy control subjects, including in those treated with lower doses of radiation (18 to 30 Gy). Further investigations are needed to better define risks and to inform screening recommendations. Abstinence from smoking should be emphasized. Aggressive medical management of other risk factors associated with cerebrovascular disease in the general population should also be advised.
Modifiable Cardiovascular Risk Factors
As noted earlier, attention to modifiable cardiovascular risk factors, such as obesity, hypertension, diabetes mellitus, dyslipidemia, and smoking, may be particularly important in the care of survivors of childhood cancer. A recent assessment from the CCSS found that two or more cardiovascular risk factors were reported by 10.3% of survivors. In this report, acquisition of modifiable cardiovascular risk factors, particularly hypertension, increased the risk for severe, life-threatening, and fatal cardiac events. These results reinforce the importance of early diagnosis and careful management of modifiable cardiovascular risk factors in survivors.
Pulmonary System
Pulmonary toxicities as a consequence of cancer-directed therapy can range in severity from asymptomatic abnormalities on pulmonary function testing to life-threatening disease. Childhood cancer survivors are at increased risk for death from pulmonary complications. In an analysis of late mortality among 5-year survivors from the CCSS cohort, childhood cancer survivors were 8.8 times more likely to die of a pulmonary event compared with the U.S. population (age-, calendar year-, and sex-matched). Therapeutic exposures, including radiation therapy, certain chemotherapeutic agents (bleomycin, carmustine, lomustine, busulfan, and cyclophosphamide), thoracic surgery, and hematopoietic stem cell transplantation, are associated with late pulmonary complications. Recognition of these toxicities informed changes in upfront cancer-directed therapies, such as the reduction or elimination of radiation therapy and bleomycin in the treatment of Hodgkin lymphoma.
The late effects of radiation therapy on the respiratory system include reduced lung volumes, pulmonary fibrosis, and decreased chest wall growth. Risk factors for radiation-induced pulmonary late effects include concurrent pulmonary toxic chemotherapy, lung radiation dose greater than 15 Gy, spinal irradiation, and total-body irradiation. Data from the CCSS cohort showed an increased risk for pulmonary fibrosis (RR, 4.3), emphysema (RR, 2.0), abnormal chest wall development (RR, 5), and chronic pneumonia (RR, 2.2) in those survivors who had chest irradiation. At 20 years after diagnosis, chest irradiation was associated with a cumulative incidence of pulmonary fibrosis of 3.5%.
Treatment with chemotherapy including bleomycin, busulfan, cyclophosphamide, and nitrosoureas is also associated with the development of pulmonary fibrosis. In the CCSS cohort, an increased risk for pulmonary fibrosis was seen in those exposed to bleomycin (RR, 1.4), carmustine (RR, 1.6), and busulfan (RR, 2.1). A plateau in cumulative incidence of pulmonary fibrosis was not observed up to 15 years after diagnosis in survivors treated with chemotherapy alone or in combination with radiation therapy.
Current long-term follow-up guidelines include obtaining a baseline chest radiograph and pulmonary function testing, including spirometry and diffusing capacity, in survivors with significant risk factors for late pulmonary toxicity. Attention to smoking abstinence and cessation is also recommended. Reports of worsening bleomycin-associated pulmonary fibrosis after exposure to 100% oxygen suggest that survivors receive a pulmonary evaluation before undergoing anesthesia and avoid situations with increased oxygen concentrations such as scuba diving.
Endocrine System
Hypothalamic-Pituitary Dysfunction
Hypothalamic pituitary dysfunction is a potential late effect in childhood cancer survivors who undergo radiation therapy for CNS, orbital, facial, or nasopharyngeal cancers. CNS tumors of the optic pathway, hypothalamus, or suprasellar region can also cause long-term hypothalamic-pituitary dysfunction as a result of hypothalamic injury from tumor growth or surgical resection. The incidence of pituitary hormone deficiencies after cranial irradiation varies by radiation dose and other treatment factors, such as age at therapy and duration of follow-up. The reported range in prevalence of anterior pituitary deficiencies after a median dose of 45 to 55 Gy cranial radiation is as follows: growth hormone (GH), 85% to 100%; TSH, 3% to 60%; luteinizing hormone/follicle-stimulating hormone (LH/FSH), 5% to 40%, and adrenocorticotropic hormone, 4% to 30%. This variability is a result of differences in cohort composition as well as different measures used to define hormone deficiencies. The GH pathway is the most sensitive to radiation and, therefore, GH deficiency is the most likely manifestation of hypothalamic hypopituitarism in survivors treated with cranial irradiation. The pituitary-thyroid, gonadal, and adrenal axes are less sensitive to late onset radiation toxicity, and, as such, centrally mediated deficiencies of these hormones are usually not observed in survivors treated with cranial irradiation below the range of 35 Gy.
Growth Hormone Deficiency.
The severity and latency of GH deficiency diagnosed in survivors is related to cranial irradiation dose. GH deficiency can be observed after doses as low as 18 to 24 Gy with late onset typically more than 10 years after therapy, whereas a cranial dose of more than 30 Gy can result in GH deficiency within 5 years after treatment. This suggests that after a dose threshold of 18 to 25 Gy the incidence of GH deficiency increases with increased period of follow-up. Clinical features of GH deficiency in survivors who have cranial irradiation can include poor growth, increased fat mass, reduction in lean body mass, decreased strength and exercise tolerance, adverse lipid profile, and decreased bone mineral density (BMD). These clinical features can be improved by GH replacement. However, the use of GH replacement in GH-deficient survivors after they obtain final height is controversial, with more to be determined regarding the long-term benefits, safety, and risks for GH replacement in GH-deficient survivors in adulthood.
The safety of GH replacement in childhood cancer survivors with regard to the risk for cancer recurrence or the development of a second primary cancer is controversial. Several studies report a lack of association between history of treatment with GH and primary cancer recurrence. In a report from the CCSS, there was no documented increased risk for primary cancer recurrence in GH-treated survivors. However, exposure to GH was associated with an increased risk for second neoplasms (RR, 3.21; 95% CI, 1.88 to 5.46). An updated report from the CCSS continued to demonstrate this association (RR, 2.15; 95% CI, 1.33 to 3.47). The association between development of second cancers and GH treatment is based on relatively few events and could be confounded by ascertainment bias (GH-treated patients could have had increased surveillance) and suggests the need for further study. The frequency and duration of screening for GH deficiency in childhood cancer survivors, the long-term effects and safety of GH replacement therapy, and the utility of long-term replacement therapy beyond attainment of final height require further investigation.
Skeletal Toxicities
Skeletal toxicities, including low BMD and osteonecrosis are increasingly recognized potential complications of therapy for childhood cancer. In children and adolescents undergoing treatment for cancer, acquisition of peak bone mass may be compromised. Cancer-directed treatment exposures, nutritional deficiencies, or physical inactivity can all contribute to decreased BMD in the setting of treatment of childhood cancer. Risk factors for low BMD identified in cohorts of childhood cancer survivors include prior treatment with corticosteroids, methotrexate, cranial irradiation, and total-body irradiation. Survivors with hypogonadism, nutritional deficiencies, and prolonged immobilization or physical inactivity are also at risk for low BMD. In the general population, osteoporosis-associated fractures result in significant morbidity and mortality; however, the association between low BMD and fracture risk has not been established for childhood cancer survivors. A report from the CCSS did not note an increased prevalence of fracture in adult survivors of childhood cancer compared with siblings ; however, the risks for long-term skeletal morbidity with advancing age remain to be defined.
Baseline bone density evaluation has been advised upon entry into survivorship care for those with treatment exposures associated with low BMD. Dual-energy x-ray absorptiometry (DXA) is a commonly used modality that provides quantitative measurement of bone mineral content and indirect calculation of BMD. DXA has well-established normative pediatric values. However, as a two-dimensional technique, DXA has limitations and cannot provide direct volumetric measurements of BMD and is confounded by bone size. Use of other modalities such as quantitative computed tomography (QCT) has been largely investigational. Nutritional counseling to ensure sufficient intake of calcium and vitamin D in the diet, and supplementation in the setting of inadequate intake, has been recommended. Management of conditions associated with decreased BMD, such as GH deficiency and hypogonadism, could potentially enhance bone health; however, the overall potential risks and benefits of such interventions with limited available data on long-term bone health outcomes must be considered.
Childhood cancer survivors are at increased risk for the development of osteonecrosis (also referred to as avascular necrosis). Osteonecrosis typically effects weight-bearing joints and can result in pain, loss of function, and joint collapse requiring surgical intervention including joint replacement. Cancer treatment exposures associated with the development of osteonecrosis include glucocorticoid therapy and irradiation, with several studies indicating that exposure to dexamethasone is associated with a greater risk for osteonecrosis than exposure to prednisone. Treatment as an adolescent has consistently been identified as a risk factor for the development of osteonecrosis. Osteonecrosis has been identified as an acute toxicity of cancer therapy, with potential long-term impact on the quality of life of survivors. In the CCSS cohort, the 20-year cumulative incidence of osteonecrosis was 0.43%, with survivors 5.6 times more likely to have reported osteonecrosis compared with siblings. The risk for osteonecrosis was greatest among survivors of allogeneic stem cell transplant for underlying diagnoses of leukemia and in survivors of acute lymphoblastic leukemia, acute myelogenous leukemia, and bone sarcoma. In this study, the cumulative incidence of osteonecrosis increased with time after treatment. Whether this reflects the worsening of previously asymptomatic bony lesions or a continued risk for the development of new lesions over time is unclear. Indeed, data are limited on the longitudinal outcomes of those diagnosed with osteonecrosis and more remains to be determined about the risk for late-occurring osteonecrosis.
In addition, bone and soft tissue in treatment fields are vulnerable to irradiation-induced hypoplasia and fibrosis, resulting in reduced or uneven skeletal growth. Survivors treated with irradiation that included the spine (>12 Gy) and long bones are at risk for scoliosis, shortened trunk height, and growth asymmetry.
Disorders of Glucose Metabolism
Survivors of childhood cancer appear to be at increased risk for the development of insulin resistance and diabetes mellitus (DM). In a cross-sectional evaluation of survivors of childhood acute lymphoblastic leukemia, Oeffinger and colleagues noted that survivors of this type of leukemia treated with and without cranial irradiation had an increased prevalence of insulin resistance when compared with a cohort composed of older individuals from the same community. In a report from the CCSS, the prevalence of DM was almost twice as high in childhood cancer survivors compared with siblings, with an increased risk for DM associated with total-body irradiation (OR, 7.2; 95% CI, 3.4 to 15; P <.001), abdominal irradiation (OR, 2.7; 95% CI, 1.9 to 3.8; P <.001), and younger age at diagnosis. This increased risk for DM with abdominal radiation exposure in this cohort appeared to be independent of body mass index (BMI). Survivors of acute lymphoblastic leukemia who had cranial irradiation were 1.6 times as likely as siblings to report DM (after adjustment for BMI). Survivors treated with total-body irradiation or with abdominal irradiation have been reported to have an increased risk for DM in several recent reports. A report of 2520 survivors from France and the United Kingdom demonstrated a dose-response relationship between irradiation to the tail of the pancreas, where beta cells are concentrated, and risk for DM (RR of DM, 11.5% [95% CI 3.9 to 34] with 10 Gy or more to the tail of the pancreas). Irradiation to other part of the pancreas was not associated with an increased risk for DM in this study. Further investigation of the mechanisms of development of DM and the interplay between treatment-related and modifiable risk factors in survivors is needed. Screening for DM should be considered in survivors, with implementation of aggressive risk-reduction strategies as clinically indicated.
Thyroid Disorders
The thyroid gland is extremely sensitive to radiation toxicity; subsequently, thyroid abnormalities are among the most common irradiation-induced morbidities after childhood cancer therapy. Childhood cancer survivors exposed to thyroid radiation as a consequence of direct neck irradiation for Hodgkin lymphoma, sarcomas, nasopharyngeal or oropharyngeal carcinomas, and other tumors are at risk for thyroid abnormalities. The clinical manifestations of thyroid irradiation injury include hypothyroidism, hyperthyroidism, thyroid nodules, and thyroid cancer. Because even low doses and scatter radiation can damage thyroid tissue, survivors who received seemingly distant irradiation such as whole-lung irradiation, craniospinal irradiation, and total-body irradiation for bone marrow transplant are also at risk for thyroid abnormalities. In addition, neuroblastoma survivors treated with 131 I-MIBG are at risk for hypothyroidism. Although coadministration of potassium iodide is considered protective, a single-institution follow-up study of 16 survivors, a median 15 years after treatment, found 8 of 16 with clinical hypothyroidism. Primary hypothyroidism (low thyroxine [T 4 ] level with elevated TSH or compensated normal T 4 with elevated TSH) is the most common thyroid abnormality observed after thyroid irradiation. Survivors in the CCSS cohort had a seventeenfold increased risk for hypothyroidism, compared with siblings. The incidence of hypothyroidism increases with increasing radiation dose to the thyroid; at 20 years after treatment with 35 to 44.99 Gy the cumulative incidence is 35%, and with 45 Gy or more the cumulative incidence is 50%. Risk factors identified for hypothyroidism are larger dose of radiation, older age at diagnosis, and female gender. Although the greatest risk for hypothyroidism occurs the first 5 years after treatment, no plateau for thyroid disease has been observed and cumulative incidence continues to rise beyond 20 years of follow-up. Central hypothyroidism (low TSH, low T 4 ) can be observed as a late toxicity of cranial irradiation to the hypothalamic pituitary axis, although centrally mediated deficiencies are usually not observed in survivors treated with cranial irradiation below 35 Gy. Hyperthyroidism is an uncommon late effect and is sometimes observed after high-dose thyroid irradiation greater than 35 Gy. Irradiation-induced thyroid nodules are commonly detected in survivors treated with head and neck irradiation. A recent cohort study of over 3000 survivors reported the cumulative incidence of irradiation-associated thyroid adenomas as 5.5% (95% CI, 4.2 to 7.2) at 40 years of follow-up. A small cohort study of Hodgkin lymphoma survivors reported the median latency of thyroid nodules as 10 years (range, 1 to 25 years) after therapy, and in this study only 10% of nodules detected by physical examination or screening ultrasonography were malignant.
Recommended screening for survivors at risk for irradiation-associated thyroid disease include annual palpation of the thyroid by a skilled examiner and annual measurements of serum thyroid hormones beginning 1 year after completion of therapy. Thyroid replacement therapy is successful in treating irradiation-associated hypothyroidism. Treatment with thyroxine to suppress elevated TSH may reduce the frequency of benign nodules. Routine ultrasound screening of asymptomatic survivors is controversial, because the majority of thyroid nodules detected in survivors have an indolent course and a minority of the nodules are malignant. Survivors with palpable thyroid nodules are advised to undergo high-resolution thyroid ultrasonography with fine-needle aspiration of any large or suspicious nodules. Nodules with benign pathology can be followed by serial ultrasonography and resampled if there is interval change, and patients whose nodules that have indeterminate or malignant pathology are referred for thyroidectomy. Total thyroidectomy is often recommended to survivors because irradiation-associated thyroid cancers can be multicentric, the remaining thyroid is at risk for malignancy, and occult malignancy has been detected in nodules believed preoperatively to be benign.
Parathyroid Disorders
Neck irradiation is associated with an increased incidence of hyperparathyroidism. Studies of adults treated with head and neck irradiation for nonmalignant conditions during childhood show a 2.5-fold increased risk for hyperparathyroidism, with a prolonged latency of 25 to 50 years. Hyperparathyroidism was diagnosed in 10% (5/53) of childhood cancer survivors treated with head and neck irradiation who underwent surgery for thyroid disease at a single institution, with a latency of 15 to 34 years. Survivors treated with neck irradiation as children should have monitoring of their serum calcium concentration as they approach older adulthood, especially if they have thyroid disease.
Short Stature
Many factors can contribute to growth disturbances in childhood cancer survivors, and it may be challenging to isolate a specific cause for diminished height in an individual child. Final height in survivors can be directly affected by cancer therapy and indirectly affected by treatment-related hormonal deficiencies and poor nutrition. Identified independent risk factors for diminished final height in childhood cancer survivors include treatment with cranial irradiation (related to GH deficiency), treatment with total-body irradiation, treatment with spinal irradiation, and young age at diagnosis (<age 13 years, with children <age 4 years at greatest risk). Survivors of pediatric brain tumors and of leukemia who undergo cranial or craniospinal irradiation are at particular risk for short stature. In a report from the CCSS, among survivors of acute lymphoblastic leukemia significant risk factors for short stature included diagnosis before puberty, female sex, higher-dose cranial radiation therapy (≥20 Gy), and irradiation of the spine. In a CCSS report assessing 921 brain tumor survivors, nearly 40% of survivors were below the 10th percentile for height. Risk factors included earlier age at diagnosis and higher doses of radiation therapy to the hypothalamic-pituitary axis.
Irradiation-associated bone hypoplasia and fibrosis can directly impede skeletal growth, such that radiation fields that include the spine or lower extremities can result in diminished adult final height. Irradiation-associated spinal deformities (kyphosis or scoliosis) can also impact final adult height. Prolonged use of corticosteroids can impair longitudinal growth and reduce final adult height. Proposed mechanisms of corticosteroid-induced growth suppression include suppression of pituitary growth hormone secretion, reduction of insulin-like growth factor type 1 production by the liver, and possibly impaired action of insulin-like growth factor type 1 on bone, all of which contribute to diminished growth. Antimetabolite chemotherapy is associated with decreased bone growth and diminished final height in some clinical studies. This finding is supported by animal and in vitro models, which demonstrate that antimetabolite chemotherapy damages growth plate chondrocytes and impairs growth.
Loss of height velocity during a prolonged course of treatment without subsequent catch-up growth can also result in diminished final attained height. Interruption of growth during the active treatment period can be related to the malignant process itself, poor nutrition, or growth suppression by therapy. In a single-institution study of final height in survivors of childhood acute lymphoblastic leukemia, decrease in height growth scores in children undergoing active treatment was noted as soon as 6 months after beginning therapy.
Treatment-induced deficiencies of GH, thyroid hormone, testosterone, and estrogen can all contribute to reduced final height in survivors. The effect of hormone deficiencies on final height can be minimized by early recognition and use of hormone replacement therapy. Precocious puberty, a late effect observed after cranial irradiation, can also indirectly result in diminished final height because early onset of puberty reduces the time interval of prepubertal growth. Cranial irradiation for CNS leukemia prophylaxis (18 to 24 Gy) is associated with relatively early and precocious puberty in girls, and higher doses of cranial irradiation often used to treat brain tumors can precipitate early puberty in both males and females.
All childhood cancer survivors, and especially survivors exposed to therapies particularly associated with growth impairment such as total-body irradiation and cranial irradiation, should be monitored for appropriate growth during and after therapy.
Obesity
Obesity has been reported as a potential late complication in survivors of childhood cancer. Obesity is frequently defined as a BMI greater than the 95th percentile for age, or greater than or equal to 30 after age 21 years. Irradiation-induced hypothalamic damage has been implicated in the pathogenesis of obesity in childhood cancer survivors, resulting in impaired growth, low metabolic rate, altered body composition, and dysregulation of eating behaviors. Indeed, cranial irradiation has been identified as a risk factor for obesity in adult survivors of acute lymphoblastic leukemia. A study of 1765 young adult acute lymphoblastic leukemia survivors enrolled in the CCSS identified female gender (RR, females 2.6, males 1.9), younger age at diagnosis in females (<4 years of age), and treatment with a radiation dose greater than 20 Gy during cranial irradiation as risk factors for obesity. Treatment with lower-dose radiation therapy or chemotherapy only was not associated with an increased risk for obesity in this study. In a follow-up longitudinal analysis of changes in obesity and BMI among adult survivors of acute lymphoblastic leukemia from the CCSS, survivors treated with cranial radiation therapy had a significantly greater increase in BMI when compared with the sibling cohort. The rate of increase was not significantly increased for survivors treated with chemotherapy alone. In contrast, a prospective study measuring BMI of 422 children with acute lymphoblastic leukemia and non-Hodgkin lymphoma before, during, and after completion of therapy found no difference in the rate of BMI increase between irradiated and nonirradiated children and concluded that high BMI at diagnosis was the best predictor of adult obesity in survivors of these malignancies.
Physical inactivity as a consequence of treatment-associated morbidities, including cardiopulmonary disease that limits exercise tolerance, neurocognitive disabilities that limit coordination and participation, chronic pain, genetic factors, and social factors, all likely contribute to the obesity observed in survivors. Green and colleagues assessed risk factors for obesity among 9284 adult CCSS participants. In a multivariable analysis, impaired physical function, hypothalamic/pituitary irradiation, use of paroxetine, and younger age at cancer diagnosis were each independent predictors of obesity, whereas meeting the Centers for Disease Control and Prevention guidelines for physical activity and a reported moderate amount of anxiety were associated with a reduced risk for obesity. This study, including the findings of an association of a specific antidepressant and of poor physical functioning with obesity, highlights the importance of further investigation of the interplay between obesity, pharmacologic exposures, physical activity, and other modifiable risk factors. Survivor care should include education regarding the long-term health consequences of obesity and the reduction of modifiable risk factors for this late effect.
Metabolic Syndrome
An increased risk for metabolic syndrome and/or its component parts has been observed in survivors of childhood cancer. Metabolic syndrome is a grouping of component physiologic disturbances that is associated with an increased risk for developing cardiovascular disease and diabetes mellitus. Although there are various definitions of metabolic syndrome in the literature, definitions generally include measures of obesity, insulin resistance, dyslipidemia, and hypertension. Identified treatment-related risk factors associated with metabolic syndrome in childhood cancer survivors include cranial irradiation and total-body irradiation. Older attained age and a sedentary lifestyle have also been implicated. Because metabolic syndrome is an established risk factor for cardiovascular disease and diabetes mellitus in the general population, aggressive management of modifiable cardiovascular risk factors in survivors is warranted.
Central Nervous System
Therapy for childhood cancer can result in long-term adverse sequelae on function of the CNS and sensory organs. Surgery, radiation therapy, and chemotherapy used to treat brain tumors and solid tumors in the head and neck region and cranial irradiation and chemotherapy used as CNS prophylaxis for leukemia and lymphoma can all contribute to neurologic, cognitive, and sensory deficits observed in survivors.
Neurocognitive Impairment
There is a broad spectrum of neurocognitive impairments observed in childhood cancer survivors. These include deficits in executive functioning (planning and organization), sustained attention, memory (visual sequencing and temporal memory), processing speed, visuomotor integration, and diminished intelligence. As a result of these deficits, survivors may experience learning difficulties, academic failure, and behavioral changes. Neurocognitive impairment is observed in survivors treated with cranial radiation. Methotrexate, administered intrathecally as CNS prophylaxis or at high doses systemically (single dose >1000 mg/m 2 ), is also associated with neurocognitive late effects. Indeed, neurocognitive impairment has been noted in adult survivors of acute lymphoblastic leukemia treated with chemotherapy alone (without cranial irradiation). Brain tumor survivors treated with surgery alone are at risk for neurocognitive impairment attributable to direct brain injury from tumor growth, surgical resection, hydrocephalus, and seizures.
Risk factors for neurocognitive impairment in childhood cancer survivors treated with radiation include higher dose, young age at exposure (<3 years), female gender, and personal or family history of learning disabilities. Additional risk factors include total-body irradiation exposure and concurrent treatment with dexamethasone, methotrexate, or cytarabine. Lower-dose cranial irradiation as CNS prophylaxis for leukemia/lymphoma (18 to 24 Gy) is more commonly associated with information processing or learning disabilities, whereas higher-dose cranial irradiation necessary to treat brain tumors is more often associated with global cognitive impairments and diminished intelligence. Neurocognitive deficits are persistent, and new deficits may emerge over time or become evident during periods of increasing cognitive demands. Armstrong and colleagues reported that adult survivors of childhood acute lymphoblastic leukemia who received 24 Gy of cranial irradiation had increased immediate and delayed memory impairment. In addition, survivors who received 24 Gy of cranial irradiation were found to function at a level one to two decades older than their chronologic age on delayed memory assessments, raising concern for early onset of cognitive impairment.
Formal neurocognitive testing is used to diagnose cognitive impairments. A full battery of tests administered by specially trained psychologists is indicated to evaluate the full spectrum of potential deficits. Survivors diagnosed with neurocognitive impairments should be referred to specialized services at the treating center or in the community to facilitate access to educational, rehabilitation, social, and vocational resources. Cognitive remediation, educational interventions with individualized learning programs, and family support that address survivor specific needs are all necessary for survivors to meet full educational potential.
Neurologic Deficits
Surgery for brain tumors and head and neck cancers can result in permanent neurologic deficits that are specific to the location of the primary cancer. For example, postoperative cerebellar mutism may persist years beyond the surgical resection of a posterior fossa tumor. In addition, postoperative hydrocephalus, infections, and seizures can evolve into chronic neurologic conditions that result in neurologic deficits that can be stable or progressive over time.
Peripheral sensory and motor neuropathy presenting as weakness, paresthesias, or pain and episodic vasospasm of the digits (Raynaud phenomenon) are potentially late effects associated with vincristine and vinblastine therapy. Treatment-induced neuropathy might restrict survivors from physical activity; however, impaired motor performance, measured in a cohort of 128 childhood cancer survivors, was not associated with cumulative vincristine dose, nor was prolonged vincristine exposure associated with physical inactivity observed in acute lymphoblastic leukemia survivors in the CCSS cohort. Management of these late effects is primarily symptomatic, and a trial of agent used to treat neuropathic pain or prevent peripheral vascular spasm can be considered.
Irradiation-induced cerebrovascular disease, such moyamoya disease, vasculitis, and vascular malformations (venous-based cavernous malformations or intracranial aneurysms) have been reported. Late onset of seizures was reported in 6.5% of brain tumor survivors in the CCSS cohort and cortical irradiation of greater than 30 Gy was associated with a twofold risk for this complication. Also in the CCSS cohort, an increased risk for late-occurring stroke was reported among survivors of brain tumor (RR, 29), leukemia (RR, 6.4), and Hodgkin lymphoma (RR, 4.32). Rare neurologic late effects of radiation therapy associated with higher doses of radiation (>50 Gy) include focal brain necrosis and spinal cord myelitis.
Leukoencephalopathy, presenting as spasticity, ataxia, dysarthria, dysphagia, hemiparesis, or seizures, is a rare complication of treatment of childhood cancer and has been associated with cranial irradiation with doses of radiation greater than 18 Gy as well as chemotherapy with methotrexate and dexamethasone. Signs and symptoms of leukoencephalopathy may present without imaging abnormalities (brain MRI, MR angiography, CT); conversely, findings on neuroimaging (white matter changes, cerebral atrophy, dystrophic calcifications, mineralizing microangiopathy) do not necessarily correlate with clinical severity. Leukoencephalopathy has also been described as a late manifestation of childhood histiocytic syndromes with no known treatment association.
Late-onset neurologic complications can present as a variety of nonspecific neurologic symptoms, including headache, seizure, hemiparesis, or other focal findings. Neuroimaging is indicated for diagnostic assessment. Neurovascular abnormalities or irradiation-associated abnormalities of the brain parenchyma can also be incidental findings on neuroimaging obtained for other indications. The role of screening with neuroimaging in asymptomatic survivors is not yet defined, with no current data to support that screening asymptomatic survivors reduces morbidity or mortality from neurologic complications. However, cancer survivors who have received cranial irradiation and have nonspecific neurologic symptoms might benefit from early evaluation by a neurologist and/or early neuroimaging.
Vision
Childhood cancer survivors’ vision may be impaired by surgery, localized irradiation, total-body irradiation, or chemotherapy with corticosteroids. Visual late effects are both anatomic and functional, including cataracts, orbital hypoplasia, lacrimal gland atrophy, conjunctival corneal damage, retinopathy, glaucoma, and optic nerve damage. Cataracts are a visual late effect frequently diagnosed in bone marrow transplant survivors and survivors treated with cranial irradiation. Bone marrow transplant conditioning regimens that include total-body irradiation are associated with significant risk for cataract development that can require surgical intervention. Chemotherapy-based conditioning regimens are not as likely to induce cataracts, and those that develop are usually less severe and do not requiring intervention. Survivors with visual symptoms, those with a history of an ocular tumor, those who received total-body irradiation, or those treated with cranial, orbital, or ocular irradiation are at highest risk for late-onset ocular complications and should receive ongoing follow-up by an ophthalmologist.
Hearing
Hearing loss is a late effect experienced by childhood cancer survivors exposed to head and neck radiation and/or platinum-based chemotherapy. Radiation fields that include the temporal bone and adjacent soft tissues can cause impaired auditory function. Low-dose cranial irradiation alone (as was administered to leukemia patients) is unlikely to cause hearing loss; however, sensorineural hearing loss can occur at cranial doses greater than 50 Gy and at lower doses (40 to 50 Gy) when combined with ototoxic chemotherapy, especially when the treatment sequence is chemotherapy administered after irradiation. In a series of 157 children with brain tumors treated by radiation therapy only with a median dose of 54 Gy, the cumulative incidence of hearing loss in the speech frequency range was 27% at 5 years after therapy. The cumulative incidence of hearing loss steadily increased over the 9-year follow-up period, and no plateau was observed.
The effect of platinum-based chemotherapy exposure on hearing is dose and age dependent. Children treated at a younger age (<5 years) are more vulnerable to cisplatin ototoxicity at any dose level. The incidence of high-frequency hearing loss (4000 to 8000 Hz) is up to 50% in children treated with more than 450 mg/m 2 cumulative dose of cisplatin. Hearing loss in the speech range (500 to 3000 Hz) is rarely reported at cumulative cisplatin doses less than 360 mg/m 2 ; however, it has been reported at cumulative doses as low as 360 mg/m 2 , and the incidence is as high as 22% to 25% at cumulative dosages greater than 720 mg/m 2 . Cisplatin ototoxicity is typically bilateral and irreversible, and children with normal hearing at the completion of platinum therapy, who have not undergone cranial irradiation, are unlikely to have subsequent loss of hearing. Survivors treated with carboplatin in nonmyeloablative doses do not appear to be at risk for hearing loss, but carboplatin-containing conditioning regimens used in stem cell transplant can contribute to hearing impairment. Genetic polymorphisms associated with an increased risk for cisplatin-induced hearing loss in children have been identified. Such findings await further validation but reflect a potential for tailoring treatment and/or follow-up based on the genetic risk for chemotherapy-associated ototoxicity.
The role of otoprotectants, such as amifostine, administered before or during cancer therapy, has not been established, and studies are ongoing in this area. To minimize risk, clinicians should minimize exposure to other ototoxins, including aminoglycoside antibiotics and furosemide. In assessing a survivor, prior and current exposure to all ototoxic agents should be considered in the assessment of auditory function. The contribution of environmental factors (e.g., use of headphones, occupational exposures) to hearing loss in the young survivor population has not been documented. Survivors with treatment exposures that put them at risk for hearing loss or those with symptoms of hearing impairment should have serial auditory evaluations, and those diagnosed with hearing impairments should be prescribed hearing aids and classroom or workplace accommodations as indicated. Early intervention for auditory problems is particular important in the survivor population because hearing loss has the potential to compound already existing social, developmental, and cognitive impairments.
Reproductive System
Reproductive function depends on the complex interaction of hormonal, anatomic, and psychosocial factors. Treatment of childhood cancer directed at the brain, spinal cord, pelvis, genitourinary organs, and gonads can all impair future reproductive potential. Therapy that is directly toxic to the ovaries and testes predisposes survivors to primary hypogonadism resulting in both diminished sex hormone production (testosterone, estrogen) and damage to oocytes and spermatocytes. Secondary hypogonadism, impaired secretion of gonadotropins (LH, FSH), can be the result of radiation and surgery to the hypothalamic-pituitary axis. Alternation of the neurovascular or structural anatomy of the reproductive organs or reduction in the volume of gonadal tissue by radiation and surgery can also impair reproductive function. As with most late effects, chemotherapy-associated gonadotoxicity is directly related to the individual agent and cumulative dose. Specifically, the alkylating agents cyclophosphamide and procarbazine pose a greater risk for gonadal dysfunction than cisplatin and dacarbazine. In general, the ovary is less vulnerable to chemotherapy-induced damage than the testis. As with other late effects, irradiation-associated toxicity to the ovaries and testes is dependent on the radiation dose and field. The availability of established fertility preservation strategies for patients newly diagnosed with cancer (semen cryopreservation, oophoropexy, oocyte cryopreservation) may improve long-term fertility outcomes for survivors treated with gonadotoxic therapy.
Females
Ovarian dysfunction can be a result of acute ovarian toxicity that persists or premature ovarian failure. The majority of prepubertal and adolescent girls receiving standard-dose chemotherapy will retain or recover ovarian function; however, those receiving high-dose alkylating agents, conditioning for bone marrow transplant, or those exposed to pelvic irradiation are at risk for irreversible ovarian toxicity, which manifests as both estrogen deficiency and infertility. Of note, irradiation to the pelvis, abdomen, or spine may include the ovaries in the field. Ovarian doses greater than 10 Gy can result in complete ovarian failure, requiring hormone replacement therapy. Survivors treated with both radiation therapy and gonadal-toxic chemotherapy have a worse prognosis for ovarian function. Cranial irradiation that has a radiation dose greater than 22 Gy to the hypothalamic-pituitary access is associated with reduced fertility, and use of more than 30 Gy is associated with ovarian failure as a result of impaired secretion of gonadotropins (LH, FSH). Survivors diagnosed with ovarian failure should be offered treatment with hormone replacement therapy in consultation with an endocrinologist.
Survivors who retain or recover ovarian function after completing therapy are at risk for premature menopause (defined as cessation of menses prior to age 45 years). Risk factors for premature menopause include increasing age, older age at treatment, increasing radiation dose to the ovaries, and increasing cumulative dose of alkylating agents. The pathophysiology of therapy-induced premature menopause is reduction in number of ovarian follicles, which supports the finding that prepubertal girls with the greatest reserve of follicles are less vulnerable to premature ovarian failure than older survivors. In the historic Five-Center Study, which included 1067 female survivors, the risk for reaching early menopause (age <25 years) for those treated between the ages of 13 and 19 years was four times greater than sibling controls. Increased risk for premature menopause was observed in survivors treated with irradiation below the diaphragm (RR, 3.7), alkylating agents (RR, 9.2), or both (RR, 27). In this historical cohort, 42% of women treated with irradiation below the diaphragm and alkylating agents had reached menopause by age 31 years. The overall relative risk for premature menopause in survivors in the CCSS cohort was thirteenfold higher than sibling controls, with a cumulative incidence of 8% by age 40. In this study, the cumulative incidence of menopause for survivors exposed to alkylating agents only was 5% at age 35 years and increased to 15% by age 40 years, and survivors exposed to both radiation therapy and alkylating agents had a cumulative incidence of menopause of 15% at age 30 years that increased to 30% by age 40 years. Of note, the threshold dose of cumulative alkylating agent exposure below which ovarian function is preserved has not been determined, and in pelvic irradiation even the lowest radiation doses (1 to 99 cGy) are associated with premature menopause.
Survivors who retain ovarian function but are at risk for premature menopause should be educated about their potentially reduced window of fertility and offered referral to fertility specialists for counseling and intervention. Clinical tests to predict the precise timing of menopause are not available; however, the serum antimüllerian hormone level is promising as a predictor of ovarian reserve and is likely to be recommended for routine monitoring of cancer survivors at risk for premature menopause in the near future. Hormonal interventions to potentially prolong ovarian function have not been clinically effective. Cryopreservation of embryos (which require a partner or sperm donor) is an option for a survivor who anticipates early menopause. Recent successes in the techniques of cryopreservation of eggs during the period of ovarian function create new options for survivors at risk for premature menopause.
Males
Males treated with alkylating agents as children and adolescents are at risk for infertility secondary to impaired testicular germ cell function and low testosterone resulting from Leydig cell insufficiency. Alkylating agent gonadal toxicity is dose dependent, and pubertal status or age at exposure is not protective. Of note, the majority of male survivors with alkylating agent–induced infertility will have normal testosterone production, with normal sexual function and secondary sexual characteristics. Abnormal semen analyses have been observed with cumulative cyclophosphamide doses greater than 5.0 to 7.5 g/m 2 . However, Leydig cell insufficiency (elevated serum LH, normal testosterone) or Leydig cell failure (elevated LH, low testosterone) is associated with higher total cyclophosphamide doses (>20 g/m 2 ). Survivors treated with unilateral orchiectomy and not exposed to additional gonadal toxic therapy usually maintain adequate testicular function.
A survivor’s risk for irradiation-induced testicular dysfunction depends on radiation dose and age at exposure. Testicular germ cells are very sensitive to radiation damage. Changes in spermatogenesis can be observed after doses as low as 0.1 Gy, and irreversible azoospermia usually occurs after doses greater than 2 Gy. In contrast, testosterone production is usually preserved with testicular doses up to 20 Gy. Irreversible damage to testicular germ cell and endocrine function usually occurs after exposure to more than 20 Gy of radiation, requiring androgen replacement therapy. However, survivors who are beyond puberty at the time of diagnosis can usually tolerate higher doses of radiation before loss of testicular endocrine function.
Although reduced testicular volume on physical examination, elevated serum gonadotropins (e.g., FSH), and low serum inhibin B suggest infertility, the only definitive measures of a survivor’s potential fertility is by semen analysis or paternity. Of note, male survivors with ejaculatory azoospermia diagnosed by semen analysis soon after completion of therapy have the potential to recover spermatogenesis many years later. Thus a sexually active survivor who is not contemplating paternity must be counseled about birth control, even if he has had an abnormal semen analysis in the past. Furthermore, survivors with ejaculatory azoospermia who desire biologic paternity may be candidates for the surgical procedure of testicular biopsy with sperm extraction. These men should be referred to a urologist with this expertise for consultation.
Survivors with delayed onset of puberty or symptoms of hypoandrogenism should be screened with morning serum testosterone, LH, and FSH evaluation. If results are abnormal, testosterone replacement therapy should be guided by the appropriate subspecialist. Of note, serum testosterone levels normally decline with advancing age, so survivors at risk for treatment-associated hypoandrogenism should have serum testosterone levels reevaluated as they reach older adulthood.
Sexual Function
Surgery or irradiation of the pelvis or lumbar spine, treatment-associated hormonal insufficiencies, medical comorbidities, and the social and emotional changes associated with a cancer experience may predispose childhood cancer survivors to sexual dysfunction. An emerging literature on the prevalence and risk factors for sexual dysfunction in young adult survivors of childhood cancer shows a higher than expected prevalence of both males (20% to 32%) and females (37% to 52%) reporting a problem in one or more areas of sexual function. Normal sexual function is dependent on the complex interplay of both physical and social and emotional factors. Physical factors that can cause sexual dysfunction include disruption of normal pelvic anatomy from pelvic or spinal surgery or irradiation, hormonal deficiency, increasing age, and emotional distress. Survivors at the greatest risk for psychosexual dysfunction include those diagnosed during adolescence, those with a brain tumor, and those with poor health status; these factors may be related to social isolation and delayed psychosexual development. Because sexual function is an important aspect of quality of life and all survivors are at risk for psychosexual dysfunction related to their cancer experience, it is recommended that all adolescent and young adult survivors have an assessment of their sexual function as part of follow-up care. The assessment for sexual dysfunction in the childhood cancer survivor includes a thorough psychosexual history, sexual history, medical history, and physical examination, including Tanner staging. Sexual history should include detailed questions about social relationships; body image; sexual experiences; libido; for women, lubrication genital sensation, orgasm, dyspareunia, and postcoital bleeding; and for men, nocturnal emissions, spontaneous erections, orgasm, and quality of ejaculate. If sexual dysfunction is a concern, consultation with gynecologist, urologist, or psychotherapist is indicated.
Pregnancy
Women who undergo abdominal or pelvic irradiation who are able to conceive children are at risk for complications of their pregnancy and delivery. Irradiation-induced damage to uterine muscle and blood flow adversely affects survivors’ prognosis for a healthy full-term pregnancy. Pelvic and abdominal radiation fields that include the uterus are associated with pregnancy complications, including spontaneous abortions, preterm labor, low-birth-weight infants, infants who are small for gestational age (SGA), neonatal death, and postpartum hemorrhage. A report on more than 4000 pregnancies from the CCSS showed that the only risk factor for adverse pregnancy outcomes was pelvic irradiation, which was associated with low-birth-weight infants (RR, 1.84). No other treatment factor was a risk for any adverse pregnancy outcome in this large cohort. When the analysis was restricted to 2201 liveborn children of 1264 survivors in the CCSS cohort, maternal uterine irradiation (>5 Gy) was associated with prematurity (OR, 3.5), low birth weight (OR, 6.8), and SGA infants (OR, 4.0). Estrogen therapy does increase uterine size and stimulate blood flow but is not shown to improve uterine muscular function.
Survivors with therapy-associated cardiac toxicity (either subclinical or overt) are at risk for pregnancy-associated congestive heart failure and should be monitored before pregnancy, during pregnancy, and after delivery by obstetricians aware of potential complications. Peripartum acute congestive heart failure has been described in survivors treated with anthracyclines and chest irradiation; however, the incidence has not been determined. One study of 37 anthracycline-exposed pregnant women showed that prepregnancy left ventricular dysfunction was a risk factor for acute congestive heart failure during pregnancy, supporting the need to closely monitor at-risk survivors. Survivors with risks for other organ toxicities such as endocrinopathies, pulmonary fibrosis, and renal insufficiency should also be meticulously evaluated and monitored before pregnancy, during pregnancy, and after delivery.
Offspring
Many studies have investigated the possible association between exposure to cancer therapy during childhood and a mutagenic or teratogenic effect on future offspring. Fortunately, no study to date has found in increased risk for either congenital anomalies or cancer in the offspring of adult survivors of nonhereditary childhood cancer treated before conception, This is true for both male and female survivors of childhood cancer and survivors treated with both chemotherapy and radiation therapy. Of note, survivors of childhood cancers that are associated with genetic syndromes and hereditary cancer predisposition (e.g., Cowden syndrome, Li-Fraumeni syndrome, retinoblastoma) are at increased risk for their offspring being diagnosed with cancer. These survivors should be referred for genetic counseling and genetic testing as desired and appropriate (see Box 72-2 ). Preimplantation genetic testing may be available to survivors with a known mutation in a cancer predisposition gene who are contemplating pregnancy.
Renal System
Chronic renal disease is a late effect associated with irradiation to the kidneys as part of an abdominal field or total-body irradiation; chemotherapy with ifosfamide and high-dose cisplatin; nephrectomy, especially in combination with other nephrotoxic therapy; and treatment with other nephrotoxic agents, including antibiotics and immunosuppressants. The pathophysiology of irradiation injury to the kidney is progressive arteriolonephrosclerosis, resulting from irradiation injury of the renal microvasculature with subsequent secondary damage to the glomeruli and tubules. Irradiation-associated renal injury is dose related, and the threshold dose is approximately 15 Gy; however, nephrectomy and exposure to other nephrotoxic agents lower that threshold. In a cohort of bone marrow transplant survivors treated with fractionated total-body irradiation, 12 to 14 Gy over 3 to 4 days, late renal dysfunction was reported in 35% of acute lymphoblastic leukemia survivors and 71% of neuroblastoma survivors. Modern conditioning regimens with altered fractionation should reduce the incidence of renal insufficiency in transplant survivors.
Chemotherapy with cisplatin at doses greater than 200 mg/m 2 can cause nephropathy secondary to glomerular or tubular injury. This injury is usually acute and reversible; however, it can be stable or progressive. Carboplatin does not appear to be associated with long-term nephrotoxicity. Ifosfamide nephrotoxicity is also a result of glomerular or tubular injury and presents most often as renal tubular acidosis. The greatest risk for ifosfamide nephrotoxicity is at total dose greater than 45 g/m 2 , and age younger than 3 years. Combination nephrotoxic chemotherapy, nephrectomy, and therapy with nephrotoxic antibiotics all contribute to survivors’ risk for long-term renal damage.
The risk for chronic renal disease in survivors treated with unilateral nephrectomy alone appears to be minimal. However, data suggest that patients treated with unilateral nephrectomy do manifest subclinical evidence of early reversible renal injury detectable by elevated urine levels of microalbumin. A study linking Wilms tumor survivors in the National Wilms Tumor Study group to the U.S. End-Stage Renal Disease registry followed for a median of 11 years showed a low risk for end-stage chronic renal failure in nonsyndromic Wilms tumor survivors (cumulative incidence, 0.6% at 20 years). These data suggest that Wilms tumor survivors with clinically detectable renal dysfunction will not routinely progress to end-stage renal disease. Of note, this cohort has not reached an age when survivors might be at the greatest risk for chronic renal failure. Survivors of Wilms tumor with the highest cumulative incidence of chronic renal failure at 20 years are survivors with predisposition syndromes such as Denys-Drash syndrome (74%), WAGR syndrome (36%), genitourinary abnormalities (7%), and survivors with bilateral disease (12%). Survivors of Wilms tumor with progressive tumor in the contralateral kidney or nephrogenic rests and survivors who have undergone irradiation have also been identified as having an increased risk for renal dysfunction.
Survivors treated with nephrotoxic therapy should be routinely monitored for early clinical signs of renal dysfunction, including hypertension, proteinuria, and elevated serum creatinine concentration. Survivors with the earliest signs of renal injury might benefit from therapeutic interventions with angiotensin-converting enzyme inhibitors to reverse or halt progression of renal injury. Survivors with a single kidney should be counseled to adopt health behaviors to maintain kidney health, including hydration, avoidance of prolonged use of nonsteroidal antiinflammatory drugs, prompt treatment of urinary tract infections, and meticulous control of coexisting diabetes and hypertension.
Genitourinary System
In addition to the renal late effects discussed previously, survivors of childhood cancer are also at risk for toxicity to the genitourinary system from surgery, treatment with pelvic and whole-abdominal irradiation, and alkylating agent chemotherapy. Genitourinary surgery can result in long-term functional impairments, including urinary incontinence and sexual dysfunction. The primary late effects associated with irradiation include fibrosis or hypoplasia of the anatomic components of the male and female genitourinary systems, including urinary bladder, ureter, urethra, prostate, vagina, and uterus, resulting in chronic pain or dysfunction. Survivors experiencing anatomic or functional genitourinary late effects, including sexual dysfunction, should be referred to a gynecologist or urologist as indicated for evaluation and management of their symptoms.
Chemotherapy with cyclophosphamide and ifosfamide has been associated with protracted hemorrhagic cystitis and bladder fibrosis, although this late effect has been reduced by concurrent therapy with mesna. Hemorrhagic cystitis that develops beyond the time of therapy more often has a viral etiology or is associated with chronic graft-versus-host disease (GVHD) in transplant survivors. Although the risk for bladder pathology in childhood cancer survivors is low, routine monitoring with urinalysis of survivors exposed to cyclophosphamide and ifosfamide is clinically indicated.
Gastrointestinal System
Potential late effects of cancer therapy on the gastrointestinal system are late onset surgical complications such as adhesions, small bowel obstruction, and fecal incontinence ; irradiation-induced functional impairment; and secondary neoplasms of the gastrointestinal tract and hepatobiliary system. Hepatic fibrosis is a rare late complication of antimetabolite chemotherapy (methotrexate, mercaptopurine, thioguanine), and the fibrosis typically follows acute hepatic toxicity such as venoocclusive disease. As with other organ systems, irradiation can result in functional impairment and second cancers of the gastrointestinal organs consequently exposed to radiation as a result of location in a treatment field. The clinical spectrum of irradiation-induced gastrointestinal toxicity that has been reported includes salivary gland dysfunction, esophageal stricture, Barrett esophagus, hepatic fibrosis and cirrhosis, focal nodular hyperplasia, cholelithiasis, and chronic enterocolitis. In the CCSS, survivors had nearly twice the risk for gastrointestinal disease than that observed in the sibling cohort. Survivors were more likely to require colostomy/ileostomy (RR, 5.6 [95% CI, 2.4 to 13.1]), undergo liver biopsy (RR, 23.1 [95% CI, 7.5 to 77.8]), or self-report cirrhosis (RR, 8.9 [95% CI 2.0 to 40.0]). Risk factors for gastrointestinal toxicity in long-term survivors included older age at diagnosis (>10 years), high-dose alkylating agent and/or anthracycline exposure, abdominal irradiation, and abdominal surgery. Of note, survivors were more likely to have more than one gastrointestinal condition than their siblings and more frequently reported common conditions such as diarrhea, ulcers, polyps, and heartburn.
Common gastrointestinal symptoms reported by a childhood cancer survivor, such as abdominal pain and heartburn, should be evaluated in the context of his or her treatment history. A survivor’s risk for a serious gastrointestinal pathologic process should be considered when planning a diagnostic workup and therapy for common symptoms. Children requiring abdominal surgery as part of their cancer therapy are at risk for late onset small bowel obstruction and should be educated about his late effect. Because survivors exposed to abdominal irradiation are considered to be at higher risk for colon cancer, and self-report polyps at greater frequency, they are advised to begin colon cancer screening earlier than what is recommended for the general population (10 years after completing radiation therapy or age 35 years, whichever comes last).
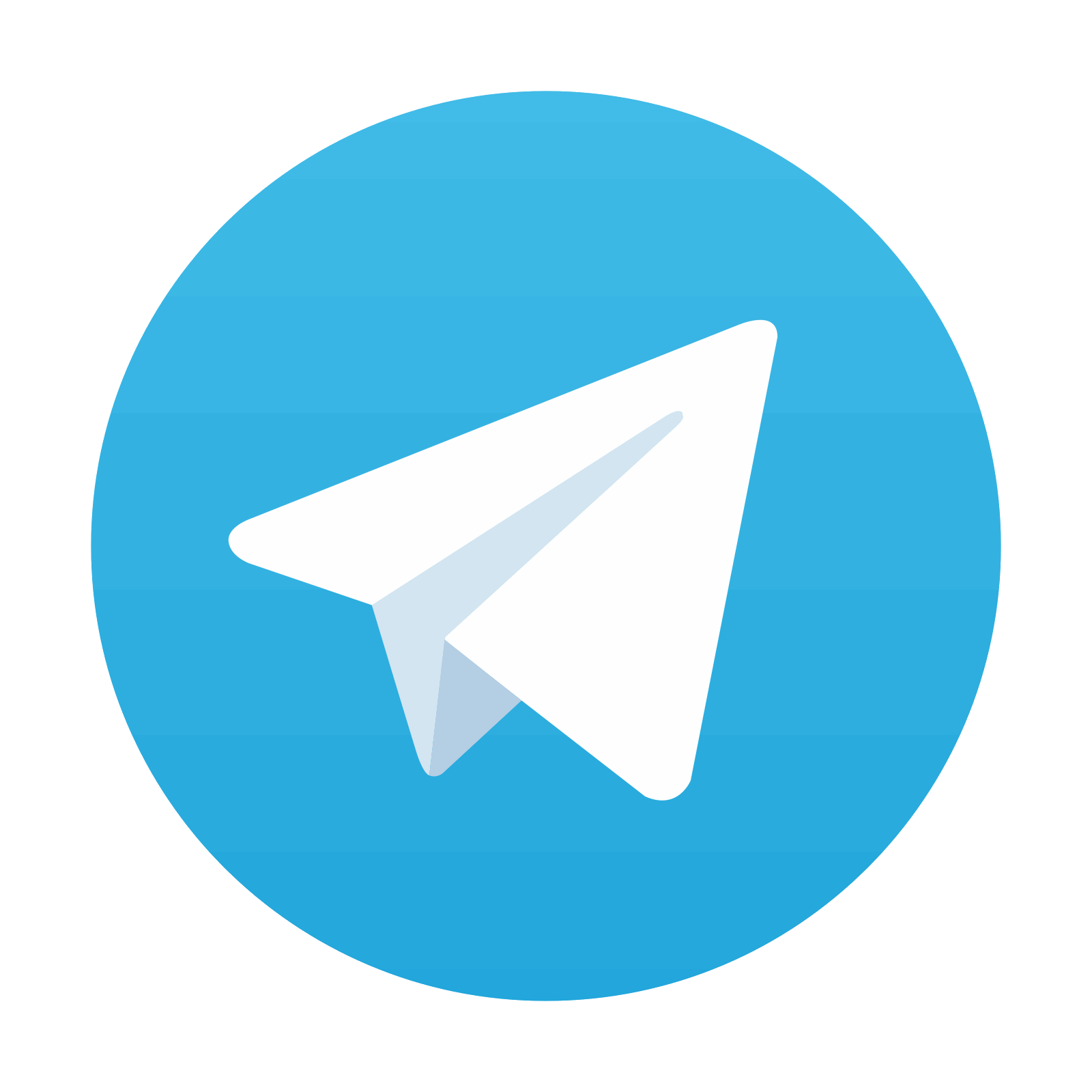
Stay updated, free articles. Join our Telegram channel

Full access? Get Clinical Tree
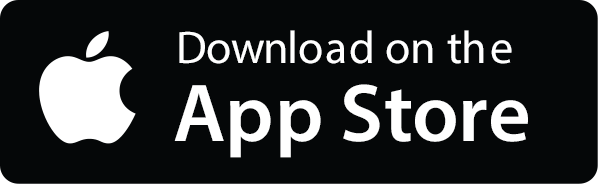
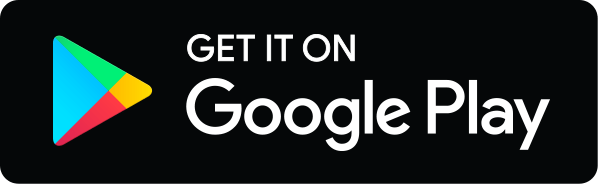