Chapter Outline
Plain Language Summary 166
Introduction 166
Improving Screening by Basing Its Application on Risk 167
Risk Factor-Adapted Mammographic Screening 168
How Many Risk Factors? 168
Familial Risk 168
Combining Standard Risk Factors: Risk Models 169
Adding Mammographic Density to Standard Measures 170
Adding Breast Cancer Risk Associated Single Nucleotide Polymorphisms (SNPs) 171
Screening Based on Prediction of Risk of Missed and of Interval Cancers 173
Effectiveness of Mammography 173
Tomosynthesis 174
MRI 175
Ultrasound 176
Improvements in the Estimation of Mammographic Density 177
Predicting the Women Who Need Screening Modalities in Addition to Mammography 177
Challenges of Risk Factor-Based Screening 178
Collecting Risk Information 178
Adding Other Risk Factors 178
Giving Risk Information 179
Organizational Issues for Screening Programs 179
Cost-Effectiveness 180
Evidence for Effectiveness 180
Opportunities of Risk Factor-Adapted Screening 180
Future Research Directions and Trends 181
Conclusions 181
List of Acronyms and Abbreviations 181
References
Plain Language Summary
There is widespread interest in trying to change the interval at which women undergo breast cancer screening based on their actual risk of breast cancer. This risk-based screening may improve the proportions of breast cancer detected early and possibly reduce the number of women who need to be screened with reduction in the associated harms of overdiagnosis. Risk factors are already used to determine screening: for example, age is used to determine who is eligible for screening and age of onset of screening may be reduced and screening frequency maybe increased in women with a family history. Women with BRCA1 or BRCA2 mutations are screened using MRI in addition to mammography because of the potential for missing cancers and the high risk of interval cancers in mutation positive women. The question arises whether such risk-adapted screening can improve the ability to detect breast cancers whilst reducing the number of women that are unnecessarily recalled for harmless changes on the mammogram. In general, incorporation of additional risk factors such as hormonal factors, the density of breast tissue on the mammogram, and genetic factors to age and family history improves the predictive accuracy of risk estimates. Additional screening modalities such as ultrasound, MRI, and digital breast tomosynthesis may increase detection rates and reduce cancers occurring between screens. However, it is not clear how these modalities and additional risk factors may be applied in breast screening and national programs, and whether this will be beneficial to the women screened. Here we discuss some of the challenges and potential opportunities of attempting to introduce risk-adapted screening.
Introduction
The use of mammography is first identified on PubMed in 1939 and population screening first referred to in 1968. The introduction of population-based screening programs occurred in the late 1980s after the results of a number of important randomized controlled trials showed a reduction in mortality from 2-yearly mammography in women particularly over 50 years of age. Most national screening programs and screening guidelines recommend annual or biennial two-view mammography, which has transitioned between 2005 and 2015 from film-screen to full field digital mammography. The UK opted for a screening interval of 3 years based on recommendations made in the Forrest Report in 1986. The original aim was to offer mammography screening to all women aged 50–64 years of age. Women were invited dependent on their locality between ages 49 and 53 years (screening vans travel around each screening area) and then offered screening on a 3-yearly basis. The upper age limit was extended to 69 years in 2000 and there is currently an age extension trial evaluating the effectiveness of an additional screen aged 47–49 years and another aged 71–73 years compared with the standard screening. Although currently screening ends at 70 (or 73 in age extension) women may self-refer for screening beyond this age. Most other countries with screening programs, including Scandinavia and the Netherlands, offer 2-yearly screening based on a period below the 30-month lead time. Countries with no screening programs, such as the USA, recommend annual screening in their guidelines.
Over the past two decades, deaths from breast cancer in most westernized countries have fallen by 30–40% in women <70 years. Some of this reduction has been attributed to mammographic screening. It is, however, difficult to distinguish the potential benefits of mammography from more widespread use of adjuvant systemic therapies and general improvements in overall care. A recent review of the UK National Health Service Breast Screening Programme (NHSBSP) estimated that it reduced breast cancer mortality by 20%, thus saving 1300 lives annually. The challenge is to improve the effectiveness of mammographic screening further. One approach is to assess the value of screening based on risk, where women at high risk are screened more frequently and earlier than those at low risk.
Improving Screening by Basing its Application on Risk
One hypothesis, which remains to be tested is that screening based on risk will lead to improvements in breast cancer mortality. Screening is more efficient in subgroups with higher disease prevalence. Risk-based screening may increase benefits and decrease harms and costs associated with screening Risk may be used to adapt several aspects of screening strategies. First, women at high risk may benefit from starting screening at an earlier age and/or stopping screening at a later age. Second, women at high risk of a true interval cancer (ie, a fast growing tumor that develops and becomes symptomatic in between screens) may benefit from more frequent screening, and women at low risk or with slow growing tumors (eg, older women and women with fatty breasts) may avoid the harms of screening by being screened less frequently. Third, women at high risk of a missed cancer on mammography (eg, women at high risk and dense breast tissue) may benefit from supplemental screening with other modalities, such as tomosynthesis, ultrasound, or MRI. Last, women at high risk of a screening harm from mammography such as a false-positive result or radiation-induced breast cancer, may benefit from an alternative screening modality such as tomosynthesis for reducing false-positive recalls and ultrasound or MRI for avoiding ionizing radiation. These are separate but highly interrelated issues. For example, breast density increases risk of breast cancer but may also decrease the sensitivity for mammography, indicating a potential need for alternative or supplemental screening modalities. Family history in both first and to a lesser extent second degree relatives increases risk of breast cancer but is also associated with a greater incidence of interval cancers compared with women without a family history. The UK National Health Service Breast Screening Programme (NHSBSP) three-yearly screening may result in more interval cancers than programs that screen more frequently. There is evidence that women at very high risk of breast cancer are more likely to develop interval cancers and reduction of the screening interval or supplemental screening in this poor prognosis group may be indicated, particularly as the cancers that do develop in women at very high risk tend to have faster doubling times than those in the general population. The Swedish two-county study showed that women with a positive family history of breast cancer were more likely to develop breast cancer between their biennial screening examinations than similar women with negative family histories.
Risk Factor-Adapted Mammographic Screening
Screening is already based on risk since age is a major breast cancer risk factor and all national screening programs and screening guidelines recommend starting screening at an age when breast cancer becomes more common. Some countries recommend younger women be screened more frequently given evidence they have more aggressive tumors. However a very recent paper suggests that menopausal status might be a better factor than age when considering screening interval. Premenopausal women were more likely to be diagnosed with later stage disease with poorer prognostic characteristics when screened biennially versus annually. However, there was no difference for postmenopausal women. And no differences within age group. Biologically, this might make sense if hormones affects aggressiveness.
In some countries, the age of commencing screening is lower and screening more frequent when another major risk factor is taken into account, namely a family history of breast cancer, since the disease arises at a younger age and often more rapidly in this group. These two risk factors are important since they are used as criteria to improve the utility and cost of screening. However, women may have other risk factors which may add to family risk or be present in the absence of a family history and the question is whether these other factors improve risk estimation and, in turn, whether their application will result in further reductions of breast cancer mortality.
Women with a family history may be referred to genetics services by their family doctor. However, factors other than age and family history are either not well known by women or their family doctor to affect risk, such as age of first pregnancy, or are occult, such as mammographic density and single nucleotide polymorphisms (SNPs), and require further investigation for their estimation. Thus further questions arise concerning the number of risk factors we need to know in order to determine risk as precisely as possible, how this information may be collected, and how such information may be given to women and applied in national screening programs and screening guidelines.
How Many Risk Factors?
If risk factor directed screening were tested in randomized trials versus standard screening, decisions would need to be made concerning the optimal methods for estimation of breast cancer risk. There may have to be a balance between what is desirable versus what is possible. A relatively simple approach is to ask women at screening for information concerning age, family history, and other factors such as age of first pregnancy. More difficult approaches include determination of mammographic density and measuring breast cancer risk SNPs and possibly adding this information to standard risk factors.
Familial Risk
Family history of breast cancer is one of the strongest risk factors for developing the disease. Early age at onset, bilaterality and multiple affected relatives, particularly on the same side of the family, increase breast cancer risk. It is estimated that 27% of breast cancer is accounted for by hereditary factors although only 15–20% of women who develop breast cancer have a family history of the disease. Approximately 10% of familial risk is associated with inheritance of mutations in high and moderate risk genes, and although important to individuals, screening for such gene mutations on a population basis is not currently practicable and is only relevant to a small proportion of women. Genome-wide association studies are likely to unravel much of the remaining “inherited component” in the next 5–10 years. Except in very rare cases such as Cowden’s disease, there are no phenotypic clues that help to identify women who carry pathogenic mutations. Evaluation of the family history, therefore, remains necessary to assess the likelihood that a predisposing gene is present within a family. Women with a strong family history of breast cancer are also at increased risk before the age at which most mass screening programs and screening guidelines recommend starting screening.
Family history clinics have been set up around the developed world since the 1980s. Evidence is emerging of the potential benefit of annual mammography in women with a first-degree family history in their forties and there may also be benefit in the thirties for women with a strong family history. For women at very high risk under age 50 years, MRI breast screening has been shown to be very effective at early detection, in particular in carriers of pathogenic mutations in BRCA1 and BRCA2 . There is some evidence for a survival benefit, but this is still not conclusive for BRCA1 carriers in whom mammography has a low sensitivity of <40% in a combined mammography and MRI-based screening program and for whom there are very high rates of interval cancers with mammographic screening alone. As a result of the strong evidence for high MRI screening sensitivity, this was recommended annually for women at very high risk of breast cancer (including BRCA1/BRCA2/TP53 carriers) aged 30–49 years of age in 2006 by NICE in the UK and also in 2007 for those at 25% or higher risk lifetime in the USA. Updated NICE guidelines in 2013 also recommend annual mammography until aged 70 years in mutation carriers (see also chapter: Screening for Breast Cancer in Women With Dense Breasts ).
Other risk factors
Although family history is the most easily identified risk factor for breast cancer apart from age, even women with no family history may be at sufficient risk to consider earlier or more frequent screening or supplemental screening by virtue of combinations of other factors. Early menarche, late first full term pregnancy or nulliparity, current combined hormone replacement therapy (HRT) use after 50 years and postmenopausal obesity can all increase the risk of breast cancer by up to 20–50% over the average. Mammographic breast density is one of the strongest risk factors, increasing risk by 4–6 fold between the bottom and top 20% of mammographic density in the population. High mammographic density also substantially increases the chances of developing interval breast cancers, suggesting that density should be incorporated into risk models. Stratification of screening frequency by risk is likely to be cost-effective. Screening interval could be shorter for women at high-risk and longer for those at low-risk. A major challenge with such a policy is identification of an individual woman’s breast cancer risk.
Combining Standard Risk Factors: Risk Models
Breast cancer risk is usually assessed using models which include family history of breast cancer and possibly other cancers (eg, ovarian), reproductive and hormonal history (age at first full term pregnancy, menarche, and menopause, and postmenopausal hormone therapy use), height and weight, and history of prior benign breast biopsies and their histology. Models generally perform well for predicting risk in a population (eg, 1 in 4 lifetime risk) but not for individual risk (“am I the one to develop cancer or one of the three who will not”). For screening on a population basis this risk stratification is probably already sufficient. The Gail, BCSC, and Tyrer-Cuzick models may be used to determine general risk whereas others, such as Claus, BRCAPRO, the Manchester Score, and BOADICEA, are currently used to predict the presence of mutations in high risk genes, such as BRCA1 and BRCA2 .
In the US, the Gail model is widely used. It is based on age, number of first degree relatives with breast cancer, number of surgical breast biopsies, and reproductive factors as above. In a comparison of models using UK data, it was demonstrated that for women with a family history of breast cancer, the Tyrer-Cuzick model performed better than the Gail model; similar results were reported in studies in the US. The Tyrer-Cuzick model has a more extensive familial component, and includes HRT use, height and weight, and the histology of surgical biopsies. The BCSC risk model also has better discriminatory accuracy that the Gail model. It includes breast density, age, race/ethnicity, first degree family history, and history of breast biopsy and was recently updated to include type of benign breast disease. It has better discriminatory accuracy than the Gail model. Given it only includes five risk factors, it is easy to use in clinical practice.
Adding Mammographic Density to Standard Measures
Mammographic breast density is one of the strongest risk factors for breast cancer increasing risk by 4–6 fold between the bottom and top 20% of mammographic density in the population suggesting that density should be incorporated into risk models. Density also has a substantial heritable component. Some data indicate that stratification of screening frequency by risk may be more effective and likely to be cost effective if density is included (see also chapter: Screening for Breast Cancer in Women with Dense Breasts ).
There is evidence that incorporating density into the standard models can increase the model’s predictive values when added to the Gail model irrespective of the method of density estimation (BIRADS, percent density, and volumetric assessment ) and to the BCSC model. In the PROCAS (Predicting the Risk Of Cancer At Screening) study, percentage density was estimated by two radiologists for each of >50,000 women in the Manchester NHSBSP using a visual analog scale (VAS) and adjusted for age and body mass index. Fig. 7.1 shows a distribution of the odds ratio for the Tyrer-Cuzick model alone, adjusted visual density, and the combination of the two. The value of such analyses may be assessed by the increase in the proportion of women appearing in the high and low risk groups. Density modestly improved on the T-C model. However, when the two methods were combined, the proportion of women in the moderate risk group (5–8% 10-year risk) increased from 8.6% using the TC model to 12.3% when the T-C results were combined with adjusted visual density. In the high risk group (>8% 10-year risk) the proportion increased from 1.2% to 2.7% ( Fig. 7.1 ).

In the PROCAS study density was also estimated using volumetric assessment of density (see below). The value of this method is that it may be estimated automatically making it more transferable to national screening programs.
The overall spread of risks can be seen in Fig. 7.2 from a combination of Gail and Tyrer-Cuzick incorporating mammographic density.

Adding Breast Cancer Risk Associated Single Nucleotide Polymorphisms (SNPs)
Incorporating common genetic variants (SNPs) into risk assessment is also likely to improve the precision of risk assessments in women with and without a family history. Using 77 SNPs in 33,673 breast cancer cases and 33,381 control women of European origin a three-fold difference in lifetime risk between women in the lowest and highest quintiles of the Polygenic Risk Score (PRS)was reported (5.2% (lowest) and 16.6% (highest) for a woman without family history, and 8.6% and 24.4% for a woman with a first-degree family history of breast cancer). Using a threshold of 2.4% for 10-year risk to commence screening 20% of the population would never reach that risk based on a PRS alone.
There have been a small number of studies using fewer SNPs to assess how to improve the prediction of risk. On the whole the increase in risk prediction has been modest (summarized in ).
In the PROCAS study salivary samples were collected from women in the study and the top 18 breast cancer risk SNPs estimated in 9346 of them ( Fig. 7.3 ).

In this subgroup of women,(relative to the main study ( n ≥ 50,000)), the proportion of women in the moderate and high risk categories increased from 9% using the T-C model alone compared with 18% when density and SNP18 were added to TC.
The addition of SNPs and density also improved the precision of predicting cancers. Fig. 7.4 shows that over 50% of the cancers occurred in the 32% of the population at above average risk (>3.5% 10-year).

%BC—Percentage of total prospective breast cancers per risk for TC alone.
%BC2—Percentage of total prospective breast cancers per risk for TCMDSNP.
Screening Based on Prediction of Risk of Missed and of Interval Cancers
Whilst tailoring screening interval according to individual risk of developing breast cancer would be an important first step, it is also important to realize the limitations of mammography as a tool for breast cancer screening, and to consider alternative screening modalities that could potentially be used to supplement mammography in a personalized screening program to identify those most at risk of an interval cancer.
Effectiveness of Mammography
Mammographic sensitivity depends on a number of variables, including the density of the breasts, the woman’s age, the nature of the cancers that occur, and factors influencing mammographic interpretation. It is important to realize the limitations of mammography and that the efficacy of mammography as a screening tool for breast cancer depends on the composition of a woman’s breasts.
The sensitivity of screening mammography (the number of cancers detected at mammography as a proportion of the sum of the screen detected cancers and those presenting as interval cancers in the following year) is approximately 90% for women with fatty breasts, but only 50–70% for those with dense breasts. This is because of the masking effect caused by dense fibroglandular tissue. Whilst younger women in general have denser breasts than older women, there is some evidence to suggest that younger age is independently associated with poorer mammographic sensitivity, probably because the more aggressive cancers that tend to occur in younger women often grow rapidly, but also have mammographic features that are more difficult to distinguish from normal tissue than other cancers. These are particular problems with the triple negative cancers that occur in women with BRCA1 gene mutations.
Review of the previous screening mammograms of women presenting with interval cancers or with cancers detected on incident screening shows that in around 25% of cases there is evidence of the cancer on the previous screening mammograms. Double reading is used routinely in some screening programs, and this has been shown to increase the screening yield by 10–15%. Single reading with computer aided detection (CAD) is also used, especially in the USA, although the high false prompt rate of CAD remains a problem with no improvement in sensitivity in a large US study in community practice.
Full field digital mammography (FFDM) has now largely replaced film-screen technology. The Digital Mammographic Imaging Screening Trial (DMIST) study from the USA demonstrated that digital mammography is significantly more sensitive than film-screen technology in women below the age of 50 and in those with dense breasts. However, breast density remains a significant factor limiting sensitivity. Women with dense breasts are at a disadvantage in a one-size-fits-all screening program; not only are they at higher risk of developing cancer, but if a cancer is present it is less readily detected. Furthermore, with the exception of the fewer than 10% of women aged over 40 who have extremely dense breasts, composite densities due to the overlap of normal tissue are more common in those with denser breasts, resulting in a higher rate of false positive recall for further assessment.
Despite these limitations, X-ray mammography has a number of advantages as a mass screening modality: it is relatively inexpensive, widely available, quick to perform, and able to detect microcalcification. Mammographic interpretation is well-established and there is a large pool of trained readers. Disadvantages of mammography, apart from the sensitivity and specificity issues noted above, include the use of ionizing radiation (although at a very low dose) and discomfort caused by the breast compression which can deter women from reattending.
Tomosynthesis
One of the most exciting recent developments in screening technology is digital breast tomosynthesis (DBT), in which a series of very low dose mammograms taken over a limited angular range are reconstructed into a series of thin (usually 1 mm) contiguous slices. This can be done in the same breast compression as a conventional 2D digital mammogram and gives a similar radiation dose. Each DBT slice contains in-focus information at a particular depth along with an out-of-focus component from other depths. As the reader scrolls through the stack the reduction in the masking effect of overlapping tissues makes many cancers (particularly those associated with architectural distortion) conspicuous. It also allows composite glandular densities seen on the corresponding 2D mammogram to be resolved.
For several reasons, including microcalcification assessment and ease of comparison with serial examinations, 2D mammography has continued to be used in addition to DBT. However, in order to reduce the need for the additional radiation, synthetic 2D mammograms created from the DBT projection images have been developed and shown to be diagnostically equivalent to conventional 2D mammograms.
Although there are no randomized trials comparing DBT with 2D mammography in the literature, a number of retrospective reader studies including the UK TOMMY study and three prospective population screening studies examining the relative contributions of 2D mammography and DBT have been published. In general these have shown a superior cancer detection performance of 2D+DBT over 2D mammography alone (40% more invasive cancers in the largest series ) and a higher specificity. In-service retrospective studies show similar findings (see also chapter: Evolution of Mammography Screening: From Film Screen to Digital Breast Tomosynthesis ).
DBT appears to offer particular advantages for screening women with dense breasts. The TOMMY study demonstrated a 93% sensitivity of DBT+2D in dense breasts compared to 86% for 2D only ( p = 0.03). Specificity was also significantly higher at 69% (95% CI 67–71) compared with 58% (95% CI 56–60) for 2D only. A retrospective review from the USA demonstrated that on multivariate analysis the greatest reductions in recall rates were seen in younger women (25% with 2D alone to 11% with DBT+2D in those below 40, 16.3% to 10.4% for those aged 40 to 49; p < 0.01) and those with dense breasts (adjusted odds ratios for heterogeneously dense and extremely dense compared to fatty of 2.17 and 1.56; p < 0.001).
Widespread implementation of screening with DBT is likely to be limited by a number of factors, including the capital cost of the mammography equipment, the need for additional digital image storage and, probably most importantly, the examination reading times which are approximately doubled. Nonetheless, even in screening programs with limited additional capacity, selective screening with DBT of women in the first screening round and continuing for those with dense breasts and at increased risk may be cost-effective, particularly as these are the women in whom the reduction in recall rate with DBT is likely to be greatest. Further, the evidence on DBT is evolving rapidly, for example one study has shown that DBT population screening based on single-reading performs substantially better than double-reading of standard mammography, and another study found that one-view DBT detected significantly more cancers than two-view mammography. More details on DBT for population screening are included in chapter “Evolution of Mammography Screening: From Film Screen to Digital Breast Tomosynthesis” .
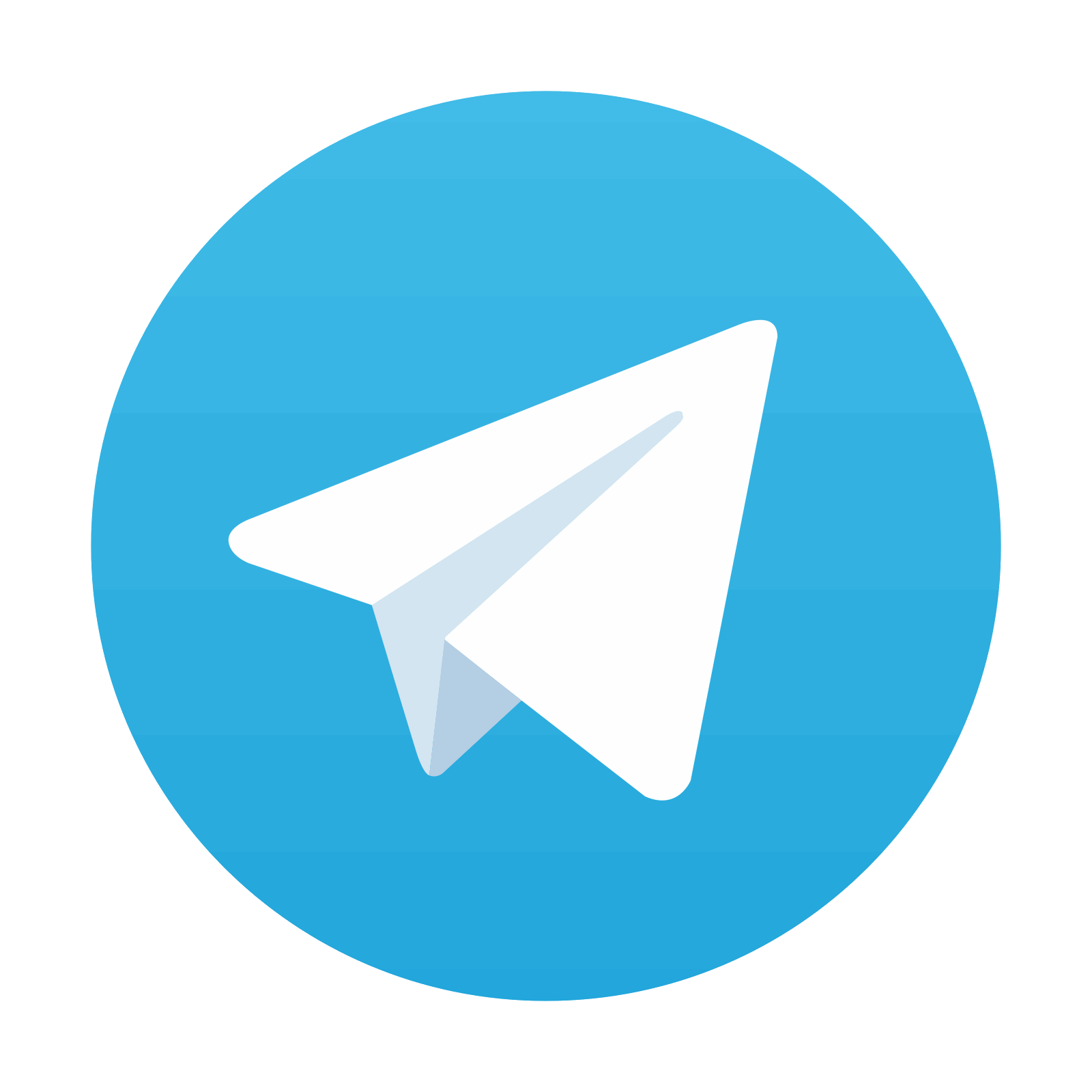
Stay updated, free articles. Join our Telegram channel

Full access? Get Clinical Tree
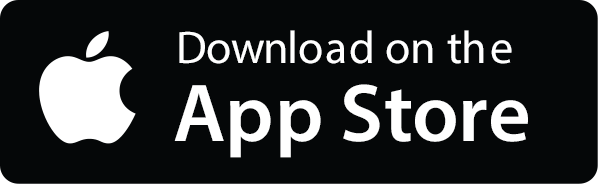
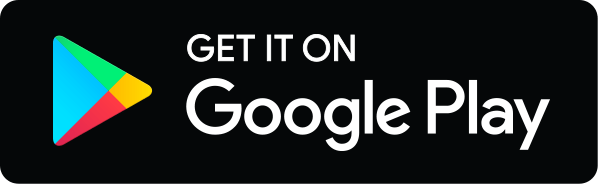