CALCITONIN
For reasons that are elucidated later, the following discussion distinguishes between CT and its precursor peptides (CTpr), which have been found to have important clinical and biologic functions. CT is initially biosynthesized as a larger precursor, procalcitonin (ProCT; Fig. 53-3), which is enzymatically cleaved into aminoprocalcitonin (NProCT) and the conjoined calcitonin:calcitonin carboxypeptide-I (CT:CCP-I), some of which, in turn, is further enzymatically processed to yieldfree immature CT and CCP-I. Immature CT is then amidated to yield the mature CT. (Procalcitonin and its subsequent posttranslational processing are discussed later.)
In this chapter, unless otherwise stated, the term CT, without further qualification, refers to the mature amidated hormone (molecular mass 3.42 kDa). The term immunoreactive calcitonin (iCT) is used to indicate either that the material being detected immunologically is not the mature hormone (i.e., it is incompletely processed CT or prohormone) or that in the particular referenced study, its precise molecular structure has not been determined or clarified. One should keep in mind that nearly all
studies have used methodologies that do not distinguish mature CT from immature CT or CTpr and were performed before the distinct biologic functions of the various CT peptides were known. This important limitation should be kept in mind when scientific data of tissue distribution, control of secretion, and bioeffects are interpreted.
studies have used methodologies that do not distinguish mature CT from immature CT or CTpr and were performed before the distinct biologic functions of the various CT peptides were known. This important limitation should be kept in mind when scientific data of tissue distribution, control of secretion, and bioeffects are interpreted.
Immature CT is a 33-amino-acid glycine-terminated peptide contained initially within the procalcitonin molecule. In the peripheral blood of normal persons, however, immature CT is found within the procalcitonin molecule as a separate peptide in which it is conjoined to the calcitonin carboxy-terminal peptide (CCP-I), and also asthe free 33-amino-acid peptide.
Mature CT is a free 32-amino-acid peptide terminating in an amidated proline. As with many other peptides, this amidation is important to the bioactivity of the hormone (see Chap. 167).
CT, in evolutionary terms, is a very conserved ancestral hormone that is found in primitive protochordate marine animals. Although the midportion of the hormone varies considerably among different species, common characteristics include the disulfide bond between the cysteine residues at the amino-terminal positions 1 and 7 and a carboxy-terminal proline amide. Of the known CTs, hamster and rat CT are the closest to the human form, differing by only two amino acids. Despite species differences, all of the CTs are bioactive in laboratory animals, although their potency and time curve of action vary with the receptor affinity and the rate of degradation.
DISTRIBUTION OF CALCITONIN
The principal source of the iCT found in mammalian serum is probably the C cells. In submammals (e.g., birds, fish, amphibians, reptiles), the thyroidal C cells occur within a discrete, separate ultimobranchial gland. During early embryogenesis, CT-containing cells migrate from the neural crest to this gland. In humans and other mammals, however, the ultimobranchial gland subsequently fuses with the thyroid gland (Fig. 53-4). In the human, the C cells (also called parafollicular cells) are found in the central portion of each lateral lobe (see Chap. 29). Within the mammalian thyroid gland, these cells constitute an endocrine system that is quite separate from the thyroid follicular cells.
In addition to the thyroid gland, iCT is found in many other tissues, such as the lungs, adrenal medulla, hypothalamus, pituitary gland, thymus, parathyroid glands, and the gastrointestinal and genitourinary tracts.6 In part, these tissue levels reflect the production of the hormone by their constituent neuroendocrine cells (see Chap. 175). However, nonneuroendocrine cells may contain low levels of iCT. Immunoreactive CT is found in the blood, urine, bile, gastric juice, and seminal fluid, and the concentration is particularly high in milk.
The wide distribution of iCT is similar to that of several other peptides of the diffuse neuroendocrine system (see Chap. 175) (e.g., somatostatin, cholecystokinin, mammalian bombesin, neurotensin) that were originally thought to emanate from a single tissue. In mammals, a large contribution to serum iCT is made by the C cells of the thyroid gland, and the responsivity of the mature CT to induced hypercalcemia appearsto reside mostly within these cells.
The highest concentration of CT in the body is found within the C-cell region of the thyroid gland. Within the lung, iCT is found in the pulmonary neuroendocrine (PNE) cells, and in humans, more iCT is found within the lungs than in the thyroid gland7; however, the extent to which extrathyroidal neuroendocrine production of CT contributes to circulating levels of the hormone is uncertain. Although the thyroid C cells originate from the neural crest, the iCT-containing cells of the gut and respiratory tract probably arise embryologically from a different source. (For a discussion of iCT in extrathyroidal tissues, see later in section Calcitonin Precursors and Derivative Peptides.)
CONTROL OF CALCITONIN SECRETION
The secretory control of CT is poorly understood. The physiologic secretagogues probably vary with the location of the hormone. Because of the hypocalcemic effects of pharmacologic doses of the hormone, early experiments searching for a possible feedback mechanism had focused on the stimulatory influence of increased serum calcium on iCT secretion.8 The relationship between serum calcium and iCT secretion is not consistent, however, and small, sudden changes of endogenous circulating calcium do not influence the serum hormone levels in humans.
Hypermagnesemia but not hyperphosphatemia induces iCT release from the thyroid gland.9 Various hormones are known torelease thyroidal iCT, some of them involving cyclic AMP (cAMP); usually, these effects are induced by pharmacologic concentrations, and whether these responses are physiologically relevant in humans is unknown. These substances include cholecystokinin, cerulein, secretin, glucagon, and gastrin. Physiologically, modulation by the sympathetic nervous system may be important because β-adrenergic agonists may induce secretion, and β-adrenergic antagonists and α-adrenergic agonists may diminish iCT secretion. Within the lung, the iCT of the pulmonary neuroendocrine cells is released by a nicotinic-cholinergic mechanism.10,11
Factors that decrease iCT secretion include dopamine, somatostatin, and the H2-receptor blocker, cimetidine.
EFFECTS OF CALCITONIN
Despite hundreds of studies, the physiologic role of CT remains unclear. Many of the investigations used pharmacologic doses of the hormone. The studies used differentin vivo and in vitro models that employed different species of the hormone and different species of animals.The full bioeffects of CT require the presence of the disulfide bridge and an intact NH2-terminal end, and the majority of pharmacologic studies have used the “mature” (processed and amidated) forms of CT.
Although multiple target tissues have been identified, bones, the gastrointestinaltract, the kidneys, and the central nervous system appear to be particularly important.
BONE EFFECTS
The osseous effect of CT is related to its inhibition of osteoclast function, which causes diminished bone resorption (Fig. 53-5).12 In addition, some data suggest that CT may also exert stimulatory effects on osteoblasts.13 In vivo, in most animals, the administration of large amounts of CT induces hypocalcemia and hypophosphatemia, an effect that is attributed to the decreased bone resorption. In vitro, CT protects against the bone-resorbing effects of parathyroid hormone, prostaglandins, and vitamin A. In humans, however, CT does not appear to play a role in the fine regulation of serum calcium. Thus, although the hormone may prevent a postprandial increase in serum calcium in some animals, this is not true in the human adult.
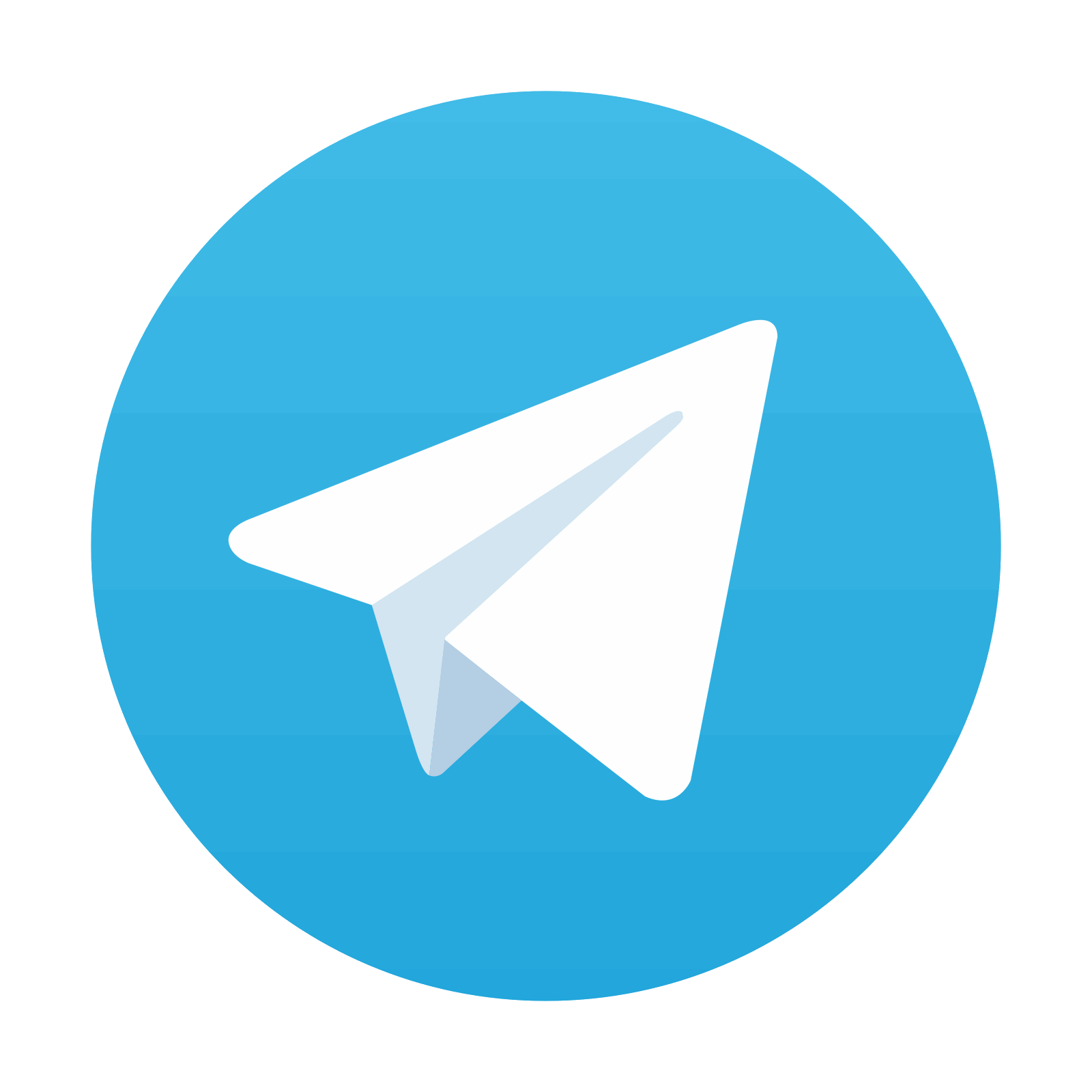
Stay updated, free articles. Join our Telegram channel

Full access? Get Clinical Tree
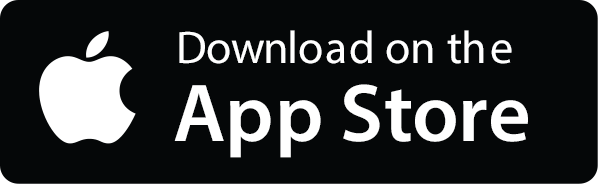
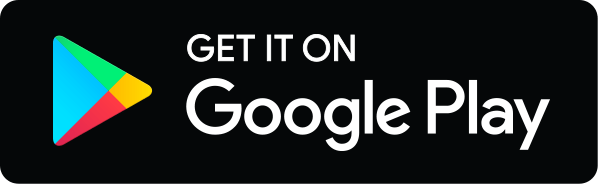
