36.1
Introduction
Bone mass, a composite measure of bone size and mineral density, is a determinant of bone strength and acquired during skeletal growth and development. Although up to 85% of bone mass is heritable, genetic polymorphisms explain only a small portion of bone mass variation in healthy individuals . Many additional factors influence bone acquisition during infancy and childhood, including gender, calcium intake, vitamin D status, physical activity, obesity, and pubertal timing. In addition, studies have highlighted the importance of the intrauterine environment and maternal factors such as maternal smoking, physical activity, and nutrition on fetal bone acquisition and long-term bone health . Animal calciotropic hormone knockout models have provided significant insights into transplacental calcium transport mechanisms, whereas epidemiologic studies have provided insights into the life-long significance of bone acquisition during fetal development, infancy, and childhood. Finally, randomized clinical trials have demonstrated that physical activity and calcium intake impact pediatric bone acquisition.
36.2
Bone acquisition in utero
36.2.1
Mineral metabolism and calciotropic hormones in utero
The human fetus accumulates 25–30 g of calcium during a normal term pregnancy . Approximately 80% is accrued during the third trimester, when the skeleton mineralizes rapidly . At 20 weeks of gestation the fetal calcium accretion rate is 50 mg/day; by 35 weeks the rate has increased to 330 mg/day . Therefore gestational age has a major effect on bone mass at birth . Similarly, most total body phosphorus and magnesium present at birth are acquired during the last trimester.
Fetal mineral transport and metabolism are uniquely adapted to provide sufficient minerals to mineralize the skeleton fully before birth. Calcium, magnesium, and phosphorus are transported actively across the placenta to meet the large mineral demands . During the third trimester, circulating total and ionized calcium and phosphate concentrations in the fetus significantly exceed maternal values, with serum magnesium concentrations minimally elevated above the maternal concentration . Near the end of gestation, fetal intact parathyroid hormone (PTH) concentrations are much lower than maternal values . Although concentrations are low, PTH availability is critically important; fetal mice lacking parathyroid glands have marked hypocalcemia and undermineralized skeletons . In late gestation, fetal 1,25-dihydroxyvitamin D [1,25(OH) 2 D] is also lower than the maternal concentration , perhaps due to high circulating serum phosphate and low PTH. As detailed later, fetuses lacking vitamin D receptors (VDRs) have normal serum mineral concentrations and normal skeletal mineralization ; therefore low 1,25(OH) 2 D concentrations may also reflect its relative unimportance for fetal mineral homeostasis.
Active transplacental calcium transport against a concentration gradient occurs by a mechanism analogous to calcium transfer across the intestinal mucosa. There are several Ca 2+ channels, transporters, and exchangers on the placental brush-border membrane facing the maternal circulation and on the basal plasma membrane facing the fetal circulation, including the calcium binding protein, Calbindin-D 28k , on the maternal side and the transient receptor potential cation channel (TRPV)-6, Ca 2+ /Na + exchanger (NCX)-1, and Calbindin-D 9k on the fetal side . Calcium entry occurs through channels, including voltage-dependent calcium entry channels, on maternal-facing basement membranes. Calcium is then carried across these cells by calbindins and actively extruded at fetal-facing basement membranes by Ca 2+ -ATPase and, to a lesser extent, by NCXs . Less is known about phosphate channels and transporters. NaPi-IIb sodium-phosphate cotransporters can be found both in placental labyrinthine cells as well as in embryonic endoderm. This suggests that this cotransporter likely has an important role in fetal phosphate homeostasis . Placental magnesium transporters are not well characterized.
Calciotropic gene knockout mice models have provided insight into maternal-to-fetal calcium transport regulation . Candidate hormonal signals included PTH, PTH-related peptide (PTHrP), 1,25(OH) 2 D, and calcitonin. Kovacs et al. utilized a VDR gene knockout model to study maternal and fetal VDR absence effects on fetal–placental calcium transfer and fetal mineral homeostasis. Although maternal VDR null mice were profoundly hypocalcemic and VDR-null fetuses had low body weight, the VDR-null fetuses maintained normal serum ionized calcium concentrations and a skeletal ash weight that was appropriate for their lower body weight. As detailed later, maternal calcium intake affects fetal bone mass . Therefore normal maternal-to-fetal calcium transfer in the murine VDR knockout model does not necessarily imply that the human fetus is unaffected by maternal hypocalcemia. Rather, it indicates that the placental unit can extract calcium from the maternal circulation in a non-vitamin D-dependent manner.
Prenatally, PTHrP, is an important regulator of fetal blood calcium and placental calcium transport . Fig. 36.1 illustrates the significantly lower ionized calcium and maternal–fetal calcium gradient in homozygous PTHrP-ablated fetal mice compared with littermates. Animal studies have also demonstrated that PTHrP regulates fetal–placental calcium transport through a receptor distinct from the PTH/PTHrP receptor. In utero injections of PTHrP(1–86) and PTHrP(67–86) significantly increased calcium accumulation in PTHrP-ablated fetuses; however, PTHrP(1–34) and intact PTH(1–84) had no effect. Therefore PTHrP bioactivity for placental calcium transport is specified by a midmolecular region that does not use the PTH/PTHrP receptor.
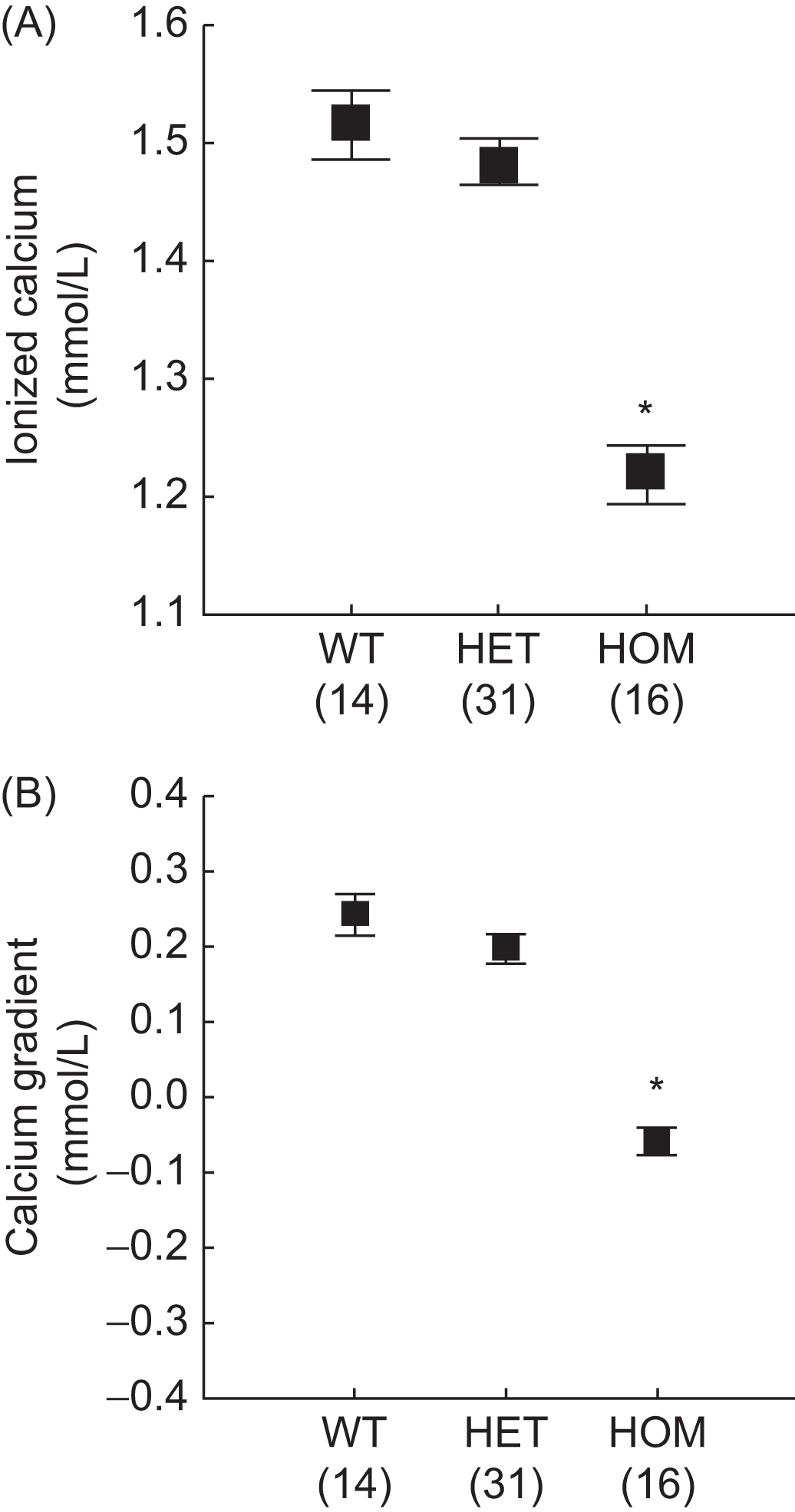
A calcitonin/calcitonin gene-related peptide (CGRP)-α gene knockout model was used to determine whether calcitonin and CGRP-α are required for normal fetal mineral homeostasis and placental calcium transfer . Fetal circulating calcium, phosphate, and PTH did not differ by genotype, and the calcium content of the fetal skeleton was normal; however, serum magnesium and skeletal magnesium content were significantly reduced in null fetuses. These findings indicated that calcitonin and CGRP-α are not necessary for normal fetal calcium metabolism but may regulate aspects of fetal magnesium metabolism.
Mothers meet fetal calcium demands by significantly increasing intestinal calcium absorption . The maternal skeleton may also contribute calcium. Prospective, longitudinal studies have demonstrated that serum 1,25(OH) 2 D concentrations increase twofold during the first trimester in human pregnancy and this increase is maintained until term . Similarly, mineral balance and calcium kinetic studies in humans have repeatedly and consistently detected a positive maternal calcium balance and an approximately twofold increase in the intestinal absorption of calcium during all three trimesters .
Maternal bone resorption markers are low in the first trimester and increase to values approximately twice normal in the last trimester . In contrast, markers of bone formation are low early in gestation and variably rise to normal levels by term. These patterns suggest that bone turnover is low in the first half of pregnancy but may be increased in the third trimester. This increase occurs at the time of the peak rate of calcium transfer to the fetus and may result from mobilization of maternal skeletal calcium stores to help supply the fetus .
36.2.2
Impact of the intrauterine environment
Given the rapid rate of fetal bone accrual, environmental perturbations may have important effects on bone acquisition . In addition, intrauterine programming may have life-long effects on bone mass and body composition through hormonal set point modulation . Evidence that childhood bone acquisition and peak bone mass are modified by the intrauterine environment comes from several sources, including retrospective cohort studies relating adult bone mass and geometry to birth weight; maternal-offspring studies demonstrating associations between maternal diet, anthropometry, physical activity, and smoking and childhood bone mass; prospective cohort studies; and prospective intervention studies demonstrating beneficial effects of maternal nutritional supplements on childhood bone health . Furthermore, VDR polymorphism and growth hormone gene polymorphism analyses suggest that gene–environment interactions during early life may determine later osteoporosis . Therefore maternal nutrition and intrauterine growth optimization should be included among strategies to optimize peak bone mass.
36.2.2.1
Fetal programming: developmental origins of osteoporosis
Environmental influences such as increased calcium and vitamin D intake and physical activity have significant benefit on childhood bone acquisition; however, these benefits are not always sustained after a particular level of intake or activity is discontinued. In contrast, there appear to be significant and sustained influences of genetic factors and the intrauterine environment on bone health. The landmark series of studies by Barker (reviewed in Ref. ) demonstrated that early life cues prepare the developing human for a particular postnatal environment. Intrauterine and early postnatal environments permanently affect body build, physiology, and metabolism; this phenomenon is known as “fetal programming.” For example, Barker demonstrated that low birth weight for gestational age, secondary to poor maternal nutrition and fetal undernourishment, was associated with increased rates of coronary heart disease and of the related disorders stroke, hypertension, and type 2 diabetes . Slow growth during infancy and rapid weight gain after 2 years of age exacerbated the effect of slow fetal growth on lifetime cardiovascular disease risk. These associations extended across the whole range of birth weights, suggesting that normal variations in fetal nutrient delivery have profound long-term effects.
Intrauterine programming contributes to the risk of osteoporosis later in life. Numerous population-based studies relate weight at birth and 1 year of age to adult bone mass, size, and density . For example, Dennison et al. evaluated the relative contributions of pre- and postnatal factors to hip and spine BMC measured by dual-energy X-ray absorptiometry (DXA) in the seventh decade of life in more than 900 men and women enrolled in the Hertfordshire Cohort Study. Birth weight was significantly associated with BMC in the spine and proximal femur in men and women; relationships between weight at 1 year of age and BMC were even stronger. There were no significant interactions between birth weight and weight at 1 year as bone mass predictors. Therefore weight at each measured point in life was important in ultimate adult bone mass determination. Adding lifestyle and other factors (e.g., age, cigarette and alcohol consumption, physical activity, social class, hormone replacement therapy, and years since menopause) contributed little to the model. A subsequent analysis of proximal femur bone geometry demonstrated that weight at 1 year of life was positively associated with measures of femoral width and intertrochanteric cross-sectional moment of inertia , supporting the hypothesis that different patterns of growth in utero and during the first year of life lead to persistent differences in bone geometry in adulthood.
Additional analyses within the Hertfordshire cohort suggest that in utero undernutrition modifies genetic influences on bone size and density . The observed relationship between adult spine BMD and VDR genotype varied according to birth weight ( Fig. 36.2 ). Among individuals in the lowest birth weight tertile, spine BMD was higher in individuals of VDR BB genotype after adjustments for age, sex, and adult weight. In contrast, spine BMD was lower in individuals of BB genotype who were in the highest birth weight tertile.
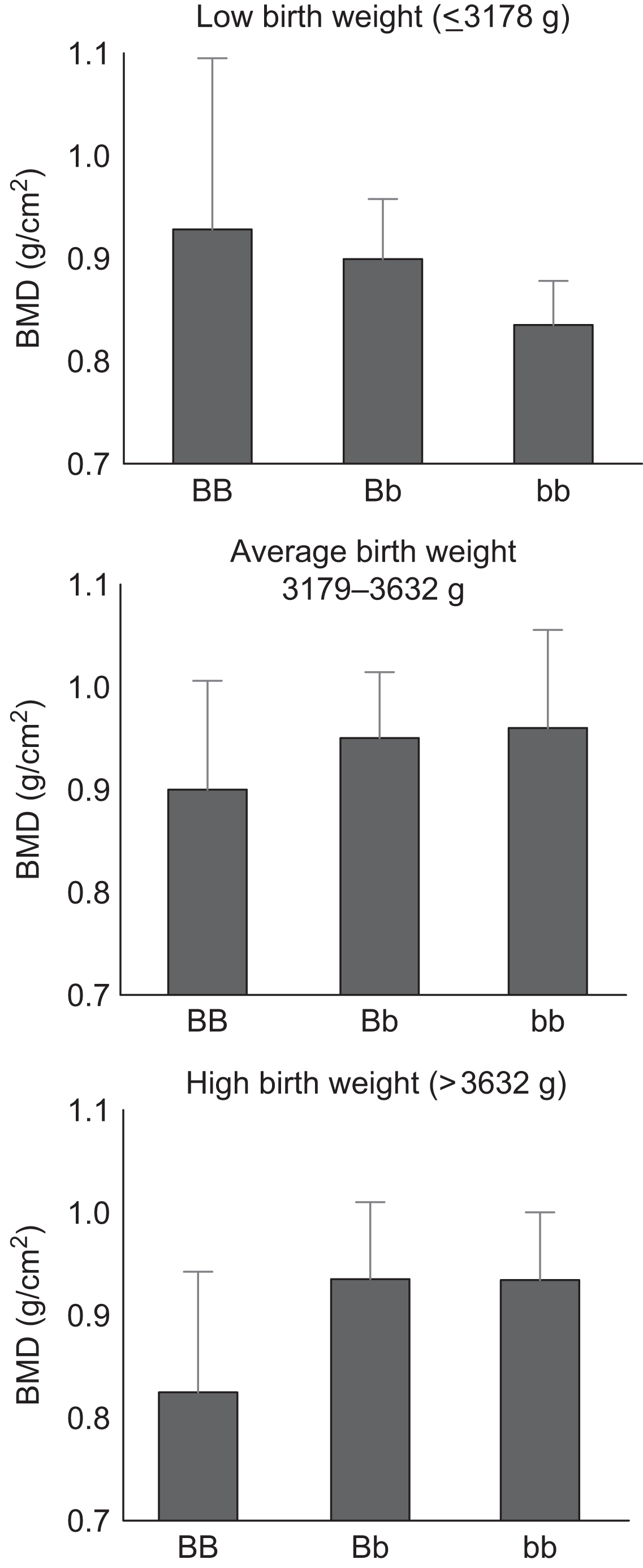
Subsequent studies from other cohorts from the United Kingdom , Australia , Finland , the United States , and India have confirmed the significant relationship between fetal growth during pregnancy, birth weight, birth length, weight gain during infancy, and bone mass in young or older adults. Ganpule et al. demonstrated that birth length was associated with whole body BMC at 6 years of age, independent of age, gender, height at 6 years of age, parental height, parental BMC, gestation, and maternal dietary intake. Antoniades et al. examined the relation between birth weight and adult bone mass in 1411 female twins at mean age 48 years, while accounting for confounding by maternal factors and a range of early environmental factors. Overall, intrapair differences in birth weight were significantly associated with differences in BMD at the spine, total hip, and femoral neck. Within the dizygotic twins the associations between BMD and birth weight were entirely explained by the birth weight association with adult height and weight. However, the associations between birth weight and BMD in the spine, femoral neck, and forearm remained significant after adjustment for intrapair differences in height and weight in the monozygotic twins. Monozygotic twins had greater intrapair variability in birth weight than dizygotic twins; this difference was attributed to greater unequal competition for nutrients or placental blood supply between monochorionic monozygotic twins (i.e., sharing a placenta).
Pearce et al. have suggested that the predominant fetal life effect on adult bone health is mediated through achieved height. They used a life course approach to quantify the direct and indirect effects of fetal (position in family, weight, and social class at birth), childhood (breastfeeding, growth, infections, social class in childhood, and age at menarche), adult life (social class, alcohol consumption, smoking, diet, reproductive history, exercise, and hormone replacement therapy use), and adult size (height and weight) factors on hip and spine BMD in 389 adults aged 49–51 years. In this cohort, birth weight was not associated with adult hip or spine BMD. Birth weight was positively associated with bone area in men and women; however, after adjustment for adult height and weight, the association remained significant in men only. Overall, fetal factors accounted for 5.2%–6.9% of BMD variation in men, and less than 1% in women. For both sexes, nearly half of the BMD variation explained by early life (fetal, infancy, and childhood combined) was mediated through adult height. Since even in the Hertfordshire cohorts the associations of low birth weight and weight at one year were stronger for BMC than BMD, it may be that patterns of skeletal growth are primarily established earlier in life and then mineralization is mediated by subsequent nutrition and skeletal loading .
Circulating hormone profiles have been examined to explain associations between birth weight and adult disease . A series of studies demonstrated that birth weight and weight at 1 year of age were associated with basal growth hormone and cortisol in elderly adults, and endogenous cortisol profiles in elderly men were determinants of rates of bone loss and BMD . Dennison et al. examined associations between common human growth hormone (GH1) gene single nucleotide polymorphisms and weight in infancy, circulating growth hormone profiles, adult bone mass, and bone loss rates in 337 elderly adults in the Hertfordshire Cohort Study. Homozygotes at loci GH1 A5157G and T6331A displayed lower basal growth hormone concentrations, lower baseline BMD, and accelerated bone loss. Furthermore, there was a significant interaction between weight at 1 year of age and GH1 genotype on rate of bone loss ( Fig. 36.3 ). Subjects who were both homozygous for a deleterious allele and had lower birth weight experienced accelerated bone loss compared with persons who had the wild-type variant. Subjects of normal or higher weight did not demonstrate this allelic association. Therefore common diversity in the GH1 region predisposes to osteoporosis via effects on growth hormone gene expression, and early environment influences the GH1 genotype effect on bone loss.
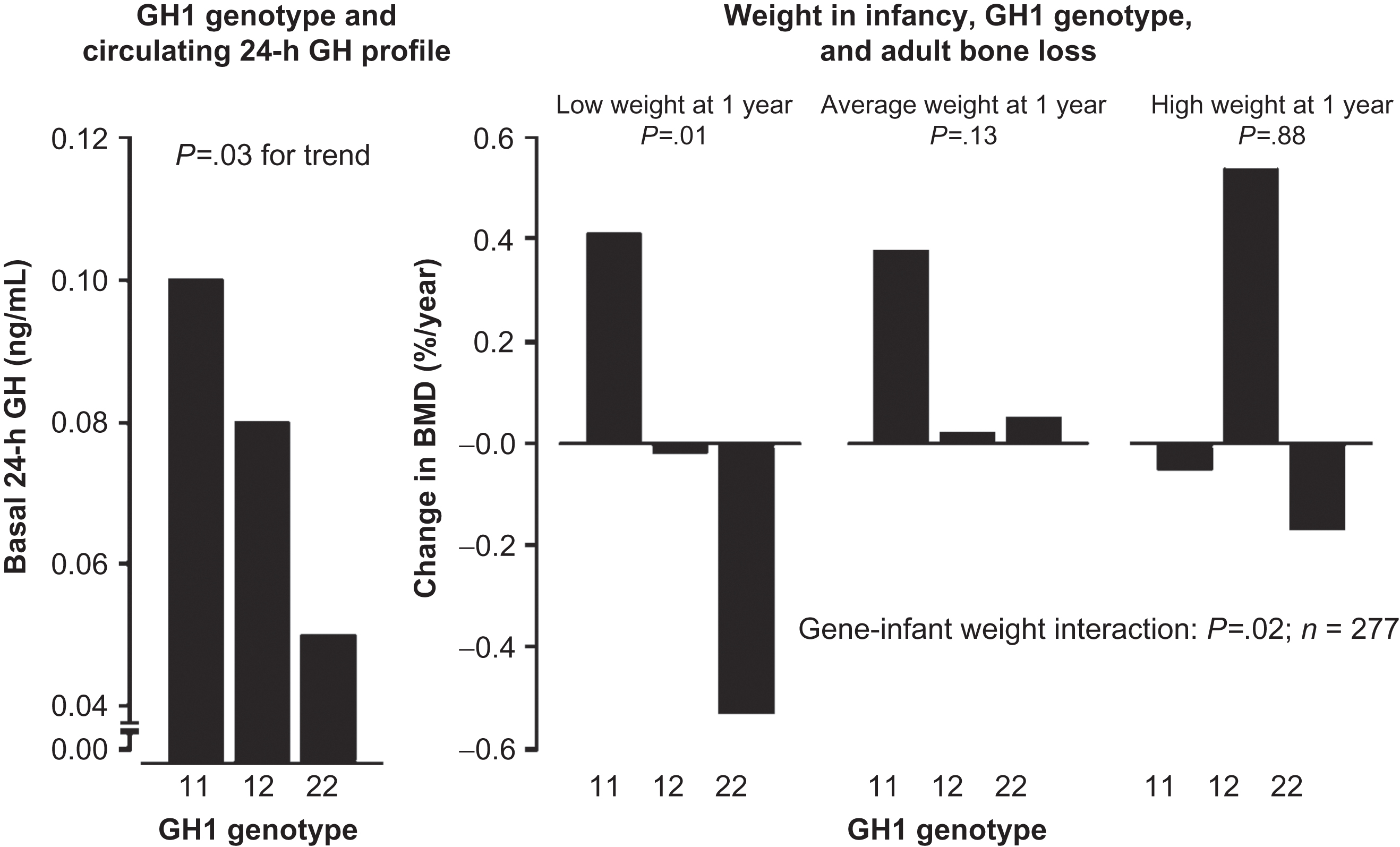
Data also support a link between fetal/infant growth rates and adult hip fracture risk . Studies of 7086 Finnish subjects, in whom birth and childhood growth data were linked to later hospital discharge records, demonstrated several major determinants of hip fracture risk (adjusted for age and sex), including tall maternal height ( P <.001) and lower birth length. A decreased trajectory of linear growth during intrauterine and early postnatal life was linked with an increased risk of hip fracture six or seven decades later.
36.2.2.2
Maternal and paternal factors
Maternal nutrition and lifestyle factors are intrauterine environment determinants of infant size. In addition, neonatal bone mass is highly correlated with body weight and length ( Fig. 36.4 ). Therefore disorders that compromise infant size (e.g., intrauterine growth retardation) or result in a premature delivery significantly affect neonatal bone mass. It is important to consider the confounding effect of body size when assessing maternal factors’ impact on newborn bone mass. For example, Fig. 36.4 illustrates that an estimate of volumetric BMD that is generated from BMC adjusted for bone area, birth length, weight, and age eliminates the association between the bone measures and birth length, birth weight, and gestational age .
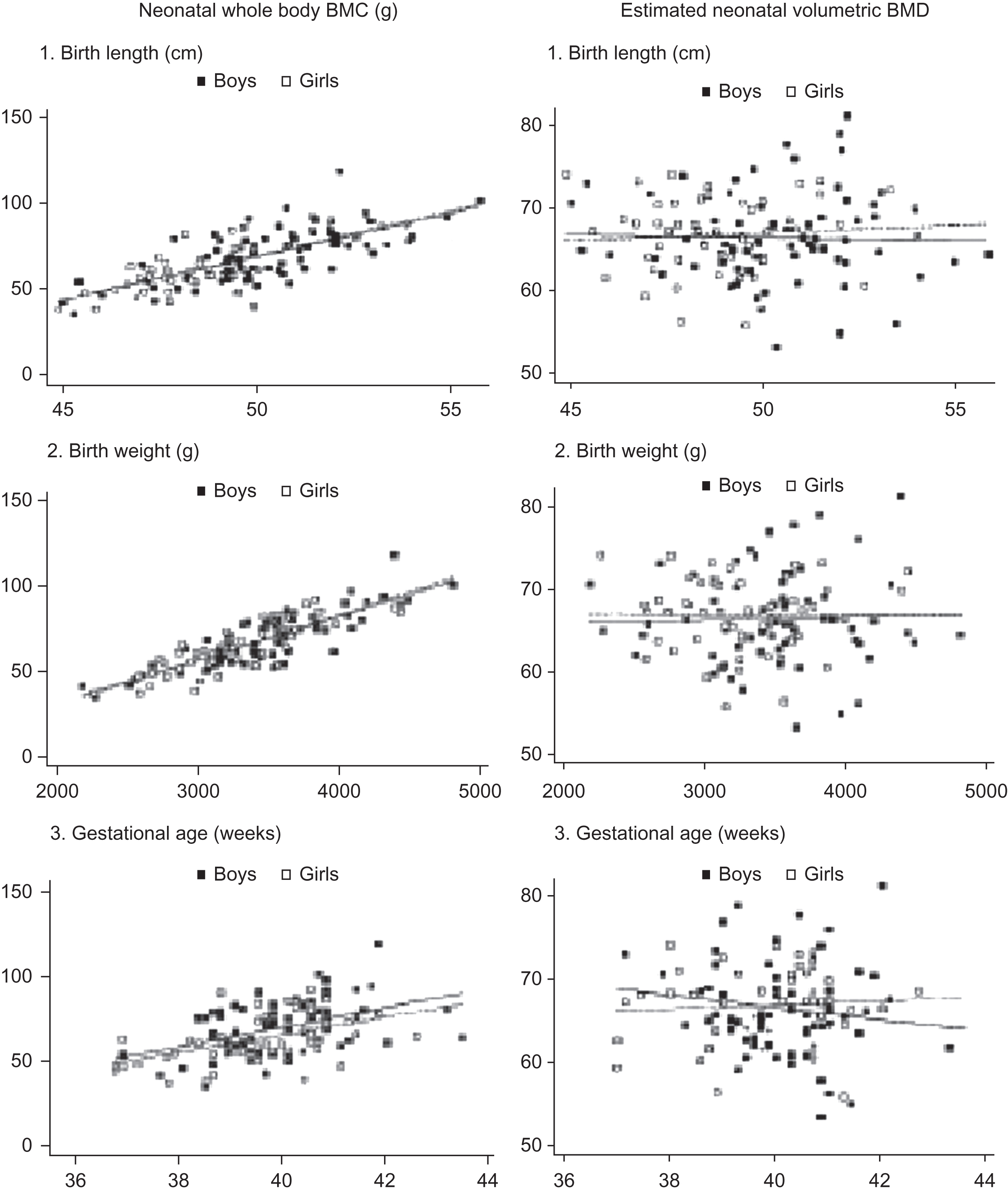
Maternal nutrition and parental body size
In a population-based cohort study of healthy term deliveries, newborn bone mass positively correlated with gestational age as expected . There were also strong positive associations between bone mass measures (whole body and spine BMC and BMD), placental weight, and body size (birth weight, head circumference, crown-heel length, and abdominal circumference) in the infants. Paternal height and birth weight have been strongly correlated with infant BMC in the spine and whole body; maternal height associated with spine BMC, and maternal birth weight associated with whole body BMC. In multivariate regression models gestational age, maternal birth weight, maternal skinfold thickness, paternal height, and paternal birth weight have had independent positive associations with infant whole body BMC. Negative associations were smoking during pregnancy, vigorous maternal physical activity, and faster walking pace during pregnancy. Infant spine BMC was positively associated with gestational age, maternal height, maternal skinfold thickness, greater physical activity (in contrast to the effects seen in the whole body), and calcium intake. Including infant length in the BMC model weakened the effects of maternal smoking and paternal height. Including placental weight in the models did not appreciably alter the coefficients for other variables.
Subsequent studies within this cohort-related umbilical cord insulin-like growth factor-1 (IGF-1) and IGF binding protein (IGFBP)-3 concentrations and cord leptin concentrations to neonatal BMC and body composition. There were strong, significant positive associations between cord serum IGF-1 concentration and whole body BMC, lean mass, and fat mass after adjusting for gestational age and sex. However, there was no association between cord serum IGF-1 and BMC adjusted for bone size. That is, cord serum IGF-1 is more closely related to the size of the neonatal skeleton than to its degree of mineralization. Neither cord serum IGF-1 nor IGFBP-3 explained the relationships previously reported between maternal influences and neonatal bone mass . There were strong positive associations between umbilical venous leptin concentration and whole body BMC and estimated volumetric BMD, lean mass, and fat mass. The associations with neonatal BMC and fat mass, but not with lean mass, were independent of the associations between cord IGF-1 concentrations and neonatal body composition. Furthermore, cord leptin explained the relationship between newborn BMC and maternal fat stores. Therefore umbilical venous leptin predicts both the size of the neonatal skeleton and its estimated volumetric mineral density, and maternal fat stores may mediate their effect on fetal bone accrual through variation in fetal leptin concentrations. In this regard, leptin may be a key hormonal mediator of the intrauterine environmental effects on early skeletal development, although in a prospective study of 57 randomized preterm newborns, lower cord leptin levels were not associated with lower lumbar spine BMD during the first 2 years of life . On the other hand, the bone-suppressive effects of maternal undernutrition shown to persist in adult rat offspring were attenuated by neonatal leptin treatment . The mechanisms by which maternal nutrition alters bone development and the developmental programming process in offspring warrants further investigation.
A woman’s own birth weight is a determinant of her offspring’s birth weight. However, animal cross-breeding experiments and human ovum donation studies suggest that this association is more dependent on the intrauterine environment than shared genotypes . Paternal height and birth weight are also associated with infant length, indicating a genetic influence on fetal skeletal growth . Although the maternal effect may be mediated by nutritional effects on the IGF axis and leptin, the paternal effect may be due to constitutive expression of the paternally imprinted IGF-2 gene . Maternal and paternal genomics in combination with fetal sex and nongenetic maternal factors have been shown to have distinct effects on fetal bone development . More studies are needed to dissect the genetic and epigenetic mechanisms that determine the observed variations in postnatal bone phenotypes.
To examine the prenatal influences on bone health further, 380 offspring of the participants in the Southampton Women’s Survey underwent DXA at age 4 years . Measures of intrauterine growth were strongly associated with childhood bone size and estimated volumetric density, suggesting that the intrauterine environment in late pregnancy may have lasting influences on postnatal skeletal development. A higher femur length growth velocity from 19 to 34 weeks’ gestation associated with skeletal size at age 4 years, whereas the change in fetal abdominal circumference (a measure of liver volume and fetal adiposity) predicted volumetric density. Both fetal measurements positively associated with BMC and areal BMD, indices influenced by both size and density. In a subsequent study within this cohort , that dichotomized fetal growth into early (11–19 weeks gestation) and late (19–34 weeks gestation) phases, early intrauterine growth was shown to more strongly relate to bone mass at age 4 years compared to late pregnancy growth that was a better predictor of bone mass at birth. These data support the notion that there is a time of adjustment postnatally prior to settling into a more stable trajectory.
Animal studies have explored potential mechanisms for maternal malnutrition’s adverse effects on fetal and early postnatal skeletal growth and bone acquisition Maternal undernutrition during early pregnancy reduces postnatal tibial growth in female offspring of rats by changing chondrogenesis . Bone acquisition depends on bone marrow mesenchymal stem cells (MSC) differentiating into osteogenic lineage cells that are responsive to maternal environment changes. In one study , poor maternal nutrition negatively affected offspring MSC by reducing proliferation by 50%, demonstrating that altered offspring growth may be mediated through reduced MSC proliferation and metabolism. Oreffo et al. demonstrated that a low protein maternal diet (9% vs 18% casein) delays MSC proliferation and differentiation during early life, although there is some subsequent catch-up. In a micro-CT study, female offspring from mothers fed a restricted protein diet during pregnancy had femoral heads with thinner, less dense trabeculae that were more likely to fail with mechanical testing. This effect was not consistent by site as the vertebrae had thicker, denser trabeculae and were structurally stronger .
Mice whose mothers consumed a lifetime high-fat diet and then themselves maintained on a high-fat diet had increased marrow adiposity in the femur as adults compared to offspring from standard chow-fed dams . Female offspring also displayed differences in bone morphology, including more rod-like trabecular structure . There is evidence for a role of vitamin K-dependent proteins (e.g., osteocalcin) in bone development, and studies are examining the effect of a high-fat diet during pregnancy and postnatally on vitamin D-dependent proteins and their correlation with bone development in mouse offspring . Human studies suggest a negative association between maternal fat intake during the third trimester and femoral neck and lumbar spine BMD in offspring at age 16 years . In addition, women consuming a healthy diet in late pregnancy characterized by high intakes of fruit, vegetables, and low intakes of sugar, soft drinks, and processed meats and foods had offspring with greater bone size and areal BMD at age 9 years . Additional human studies are needed to understand fully the mechanisms that underlie the skeletal response to altered perinatal nutrition .
Components of the maternal diet have also been related to bone mass in school-age children in the United Kingdom, India, and Tasmania . The studies in the United Kingdom and Tasmania reported substantial associations between in utero diet in a well-nourished population and later bone mass in their children; however, the micronutrients of greatest importance and the timing of exposure to those nutrients require further investigation. In contrast the study in India was conducted in a less well-nourished population and detected significant independent effects of maternal calcium intake at 28 weeks of gestation on bone mass in 6 year old offspring . The Avon Longitudinal Study of Parents and Children (ALSPAC) assessed maternal diet at 32 weeks of gestation and whole body and spine DXA bone mass at 9 years of age in more than 4000 children . Regression analyses were carried out between DXA BMC and BMD values and dietary factors, adjusted for age, gender, energy intake, and parental social class and education. Positive associations between maternal magnesium intake and child whole body BMC were no longer observed after adjusting for child height. Similarly, positive associations between maternal potassium intake and child spine BMC were no longer observed after adjusting for child weight. A significant association was observed between maternal dietary folate intake and spinal BMC adjusted for bone area using a linear regression model, which persisted after adjusting for height and weight. The authors hypothesized that (1) maternal magnesium intake may benefit growth through effects on maternal calcium concentrations and subsequent alterations in PTH, PTHrP, and chondrocyte differentiation and (2) maternal potassium intake may affect weight gain in utero. The relation between maternal dietary folate intake and spine BMC was independent of height and weight. However, there was no association between bone mass and maternal intake of folic acid supplements during pregnancy, suggesting that dietary folate may be a marker of a particular diet type. Nonetheless, the association between folate and bone mass is consistent with observations in adult populations . Overall, maternal diet accounted for less than 1% of the variability in childhood bone mass.
Jones et al. examined the association between maternal diet during the third trimester of pregnancy and bone mass in 173 Tasmanian children (8 years of age). After adjustment for confounders, femoral neck BMD was positively associated with magnesium and phosphorus density of the maternal diet; lumbar spine BMD was positively associated with magnesium, phosphorus, and potassium and negatively associated with fat density; and whole body BMD was positively associated with magnesium, potassium, and protein and negatively associated with fat density (all P <.05). After further adjustment for other significant dietary factors, the only significant remaining associations observed were for phosphorus and fat at the lumbar spine. Calcium intake was not associated with BMD at any site, possibly due to a high average intake.
A 10-year retrospective cohort study conducted in 350 pregnant African American adolescents (ages 12.7–17.9 years) examined determinants of fetal femur length obtained by prenatal ultrasound between 20 and 34 weeks of gestation . Maternal dairy intake was significantly and positively associated with fetal femur growth after adjustment for gestational age, biparietal diameter, maternal age and height, and prepregnancy BMI ( P =.001, r 2 =0.97). The relation between dairy intake and femur length may be due to calcium or other nutrients in dairy foods, such as phosphorus, magnesium, zinc, and vitamin D. Relationships between the growth of the fetal femur and the degree of mineralization are not known.
Maternal dietary intake was examined at 18 and 28 weeks of gestation in a cohort of 700 children in rural India, and DXA scans were performed in both parents and children 6 years after pregnancy . Compared to the US growth statistics, the children were thin (mean BMI Z -score −2.06 in males and −2.12 in females) and short (mean height Z -score −1.24 in males and −1.37 in females). Six percent of children were small for gestational age (SGA), and these children had lower whole body BMC; however, these differences were consistent with their lower weight and height. The mother’s energy, protein, and calcium intakes were low compared to Indian Council of Medical Research recommendations. Higher socioeconomic status was associated with greater milk and calcium intake. Both parents’ DXA measurements positively correlated with equivalent measurements in the children ( P <.001 for all). These relationships were independent of the child’s height, and the strength of these correlations was similar for fathers and mothers. Higher maternal parity was independently associated with lower whole body BMC in the child. Children of mothers who had a higher calcium-rich food intake during pregnancy (milk, milk products, pulses, nonvegetarian foods, green leafy vegetables, and fruit) had higher whole body and spine BMC and BMD, and children of mothers with higher folate status at 28 weeks of gestation had higher whole body and spine BMD. These relationships were independent of age, sex, socioeconomic status, maternal energy and protein intake, tobacco use, parental size and parental DXA measurements, placental weight, and the child’s height at the time of the DXA scan. The association between maternal calcium intake and whole body BMD is shown in Fig. 36.5 .
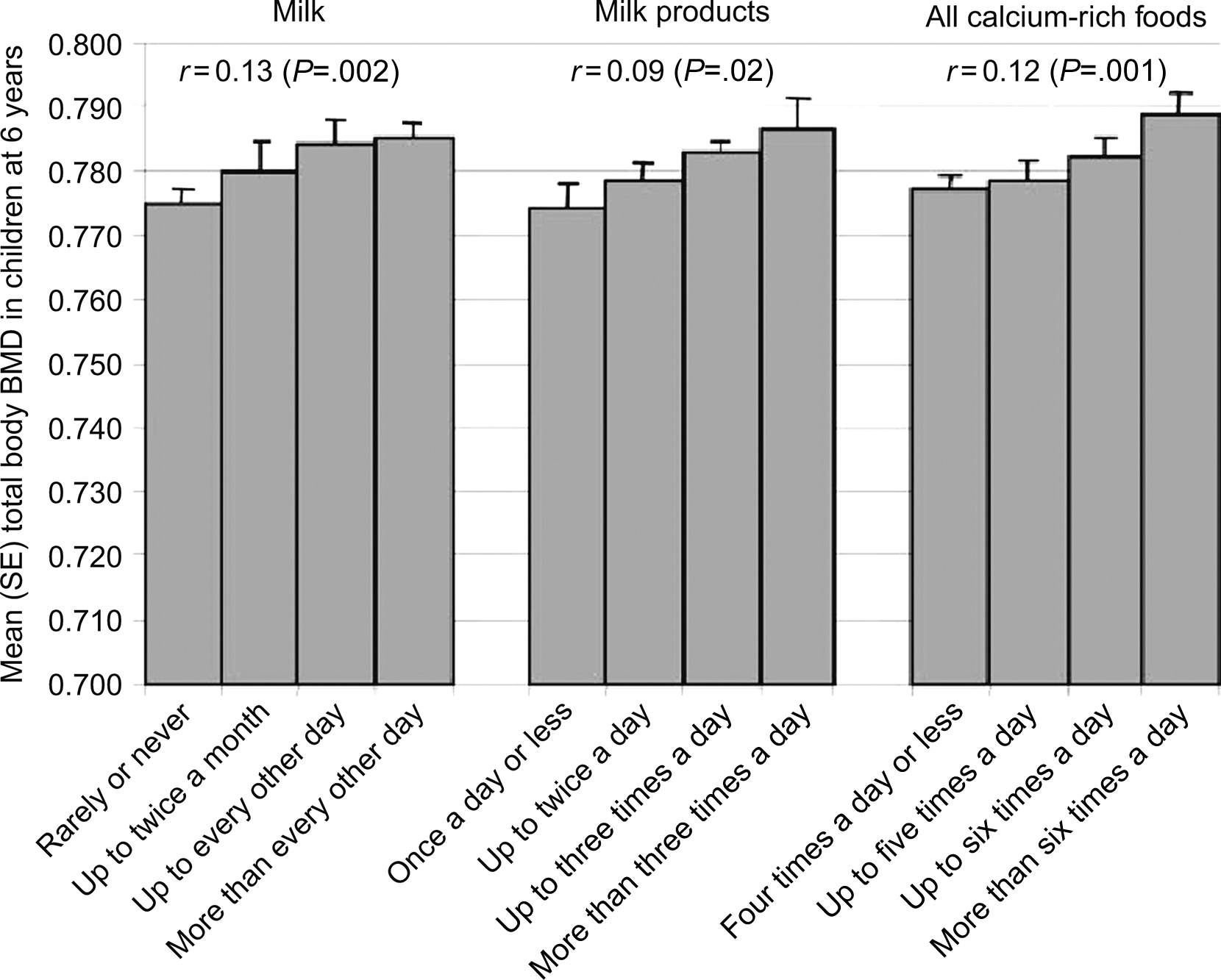
A prospective Dutch population–based cohort study of 2819 mothers examined the associations of maternal diets during the first-trimester with childhood bone mass at a median age of 6 years . In addition to the expected positive associations with maternal calcium and phosphorus intakes, this study found positive associations between childhood bone mass and maternal protein intake and vitamin B12 concentrations. Carbohydrate intake and homocysteine concentrations were negatively associated. Maternal fat, magnesium intake, and folate concentrations were not associated with childhood bone mass. In the fully adjusted regression model, maternal phosphorus intake and homocysteine concentrations most strongly affected bone mineral status, whereas maternal protein intake and vitamin B12 concentrations most strongly predicted bone mass adjusted for bone size in childhood. Protein is a component of the organic bone matrix and calcium and phosphorus are the main bone-forming minerals, suggesting a potential direct effect on fetal bone accrual, though additional studies are needed to support a link between maternal diet, epigenetic changes, and offspring bone development. Potential mechanisms for the influence of vitamin B12 include a direct effect as an osteoblast activity cofactor or in the metabolism and clearance of homocysteine . Homocysteine may interfere with collagen cross-linking or shift bone metabolism toward bone resorption . Elevated homocysteine concentrations are a risk factor for hip fractures in older persons .
There are limited human data on the effects of maternal fatty acid status in pregnancy on fetal bone accrual . In 727 Southampton Women’s Survey mother-child pairs , maternal long chain polyunsaturated fatty acid (LCPUFA) status was evaluated in late pregnancy (34 weeks gestation) and associated with bone mineral density in children at age 4 years. A key finding was that the n-3 fatty acids, eicosapentaenoic acid and docosapentaenoic acid, were positively associated with whole body and spine areal BMD in children and these associations were independent of potential confounding influences. Maternal n-6 arachidonic acid as a percentage of total fatty acids was inversely related to whole body BMC and areal BMD and to spine BMC, highlighting the importance of the balance of n-6 to n-3 fatty acids for bone accrual. This suggests that maternal LCPUFA status during pregnancy may have consequences for fetal bone metabolism that persist into early childhood, although this finding and its mechanism merit further study.
Calcium supplementation during pregnancy
Although calcium intake is essential for proper postnatal skeletal mineralization, and, as outlined above, some observational studies have found positive relationships between maternal dietary calcium intake and fetal/child bone outcomes, randomized clinical trials of calcium supplementation during pregnancy have produced conflicting results (reviewed in Ref. ). Koo et al. enrolled 256 mothers by 22 weeks of gestation; they were randomized to 2000 mg/day of elemental calcium or placebo until delivery (128 subjects per group). Treatment groups had similar gestational age, birth weight, birth length, whole body, and lumbar spine BMC. However, when BMC was analyzed by treatment group within quintiles of maternal dietary calcium intake, total body BMC was significantly greater in infants born to calcium-supplemented mothers in the lowest quintile of habitual dietary calcium intake (<600 mg/day) compared with placebo controls ( Fig. 36.6 ). The beneficial effect of calcium supplementation remained significant after adjustment for maternal age and maternal BMI and also after normalization for skeletal area, body length, and whole body lean mass in the infant. Therefore maternal calcium supplementation during the second and third trimesters increased infant bone mass in women with low dietary calcium intake; however, calcium supplementation in pregnant women with adequate dietary calcium intake did not significantly improve fetal bone acquisition.
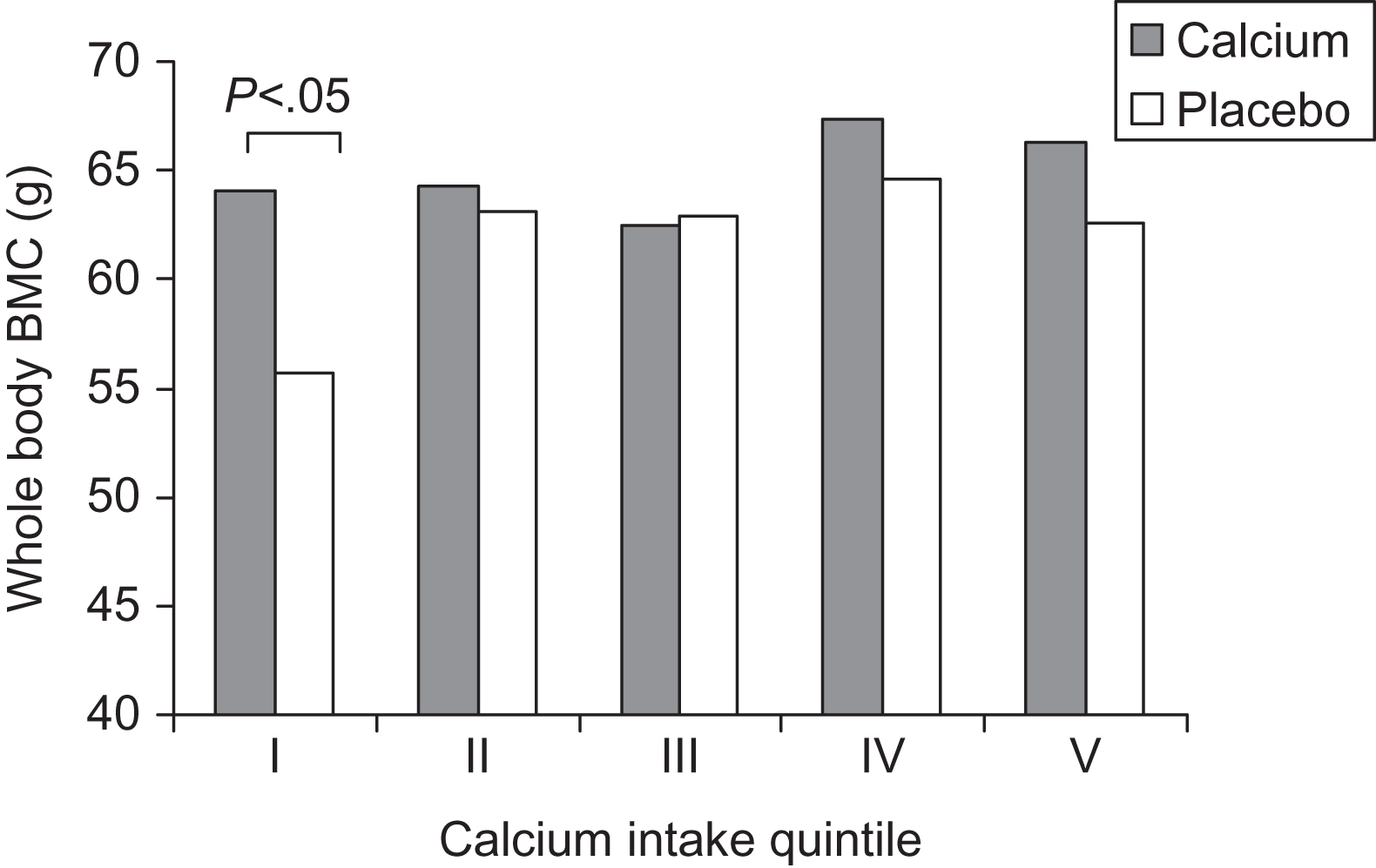
In contrast a calcium supplementation trial conducted in Gambian women with typically low calcium intake (300–400 mg/day) did not demonstrate a beneficial supplementation effect on neonatal and infant bone mass . This randomized, double-blind, placebo-controlled supplementation study was conducted in 125 Gambian women who received 1500 mg/day of elemental calcium or placebo from 20 weeks of gestation until delivery. No significant differences were detected between the groups in infant birth weight, gestational age, crown–heel length, head circumference, radius or whole body BMC, or in breast milk calcium concentration, infant growth, or bone mineral status during the first year of life. The authors hypothesized that the differences in results between their study and the one conducted in the United States may reflect differences in the timing of the bone density measurements, relative to the time of the infant’s delivery, when the exposure to the calcium supplement effectively ceased; the US neonates and Gambian neonates were scanned on day of life 2 and 12, respectively . Alternatively, it may reflect differences between the US and Gambian mothers in their adaptation to a low calcium intake or relate to the relatively small sample sizes in both studies. In a subsequent calcium supplementation trial designed to examine the effects of calcium supplementation (1500 mg/day) on blood pressure in pregnant Gambian women, calcium supplementation did not only did affect maternal blood pressure but also did not change the infant’s weight, length, and other measures of infant growth .
Vitamin D status and the impact of supplementation
Maternal serum 25(OH)D concentrations decrease during pregnancy, especially during the third trimester. At term, in cord blood, 25(OH)D values are typically 25%–30% lower than in maternal serum . Conversely maternal 1,25(OH) 2 D concentrations during the second and third trimesters are 150%–200% higher than prepregnancy, leading to increased fractional absorption of calcium .
Severe vitamin D deficiency in pregnancy has been associated with intrauterine growth retardation, abnormal neonatal ossification, and congenital rickets . Maternal vitamin D status also may influence placental calcium transfer . In rats, maternal administration of the synthetic vitamin D analogue 1-α-hydroxycholecalciferol, a bioactive form of vitamin D, resulted in greater fetal calcium content .
Prospective prenatal vitamin D supplementations studies have been performed in populations at high risk of deficiency . Marya et al. conducted a trial of vitamin D supplementation in 120 women in India. Seventy-five women received no supplementation, 25 women received 1200 IU vitamin D and 375 mg calcium per day during the last trimester, and 20 women received two doses of 600,000 IU of vitamin D orally at 7 and 8 months of pregnancy. The vitamin D supplemented groups had lower maternal and cord blood alkaline phosphatase concentrations, and mean birth weight was greater in infants of women treated with 600,000 IU vitamin D (3.14±0.45 kg) than in the infants of women either treated with 1200 IU vitamin D and calcium (2.89±0.32 kg) or not supplemented (2.73±0.36 kg).
Obstetric and neonatal outcomes of maternal vitamin D supplementation were examined in Pakistani women who received routine care (200 mg ferrous sulfate and 600 mg calcium daily) or routine care and vitamin D supplementation (4000 IU daily). Maternal vitamin D supplementation improved maternal and neonatal vitamin D status. The vitamin D supplemented group had higher Apgar scores at 1- and 5-minutes, but the obstetric outcomes and neonatal anthropometric parameters were comparable between groups . In a randomized controlled trial in Bangladesh , high-dose antenatal vitamin D supplementation (35,000 IU/week from 26 to 29 weeks of gestation) increased maternal and cord 25(OH)D and attenuated the early postnatal calcium nadir from birth to day 3 of life, suggesting that the effect of supplementation was mediated by change in maternal-infant vitamin D status. Vitamin D supplementation did not increase the risk of postnatal hypercalcemia. Vitamin D supplementation during midpregnancy (17–24 weeks of gestation) until birth or until 6 months postpartum had expected effects on maternal and infant 25(OH)D and calcium concentrations, maternal PTH and urinary calcium excretion, but maternal vitamin D supplementation did not improve fetal or infant growth at 1 year of age among 1164 infants . A metaanalysis concluded that vitamin D supplementation during pregnancy reduced the risk of infants being SGA and improved infant growth; another systematic review concluded that the evidence is insufficient to support definite clinical recommendations regarding vitamin D supplementation in pregnancy .
Studies conducted more than 45 years ago demonstrated nutritional osteomalacia in pediatric and adult immigrants living in urban communities in the United Kingdom . Immigrant women were particularly at risk of vitamin D deficiency during pregnancy. Neonatal consequences of this deficiency included hypocalcemia and overt rickets . Brooke et al. reported the results of a randomized placebo-controlled trial of 1000 IU/day ergocalciferol supplementation during the third trimester in 126 pregnant Asian women living in the United Kingdom (predominantly from the Indian subcontinent) with low 25(OH)D concentrations (mean, 8.0±0.8 ng/mL). Overall, 37% of participants had 25(OH)D concentrations less than 4 ng/mL at enrollment and the proportion increased to 53% at term among women in the placebo group. Mothers in the treatment group gained weight faster in the last trimester than those in the control group, and at term they and their infants had adequate plasma 25(OH)D concentrations. Mothers and infants in the control group had lower plasma 25(OH)D and calcium and raised alkaline phosphatase (bone isoenzyme) activity. Five control group infants developed symptomatic hypocalcemia. Infants in the control group, however, had significantly larger fontanelles, suggesting impaired ossification of the skull. Almost twice as many infants in the control group were SGA (29% vs 15%), yet there were no significant group differences in mean birth weight or length. Postnatally, infant weights diverged in the supplemented and placebo groups. The incremental increase in weight during the first 12 months of life was 5.92±0.92 kg in the infants of control mothers and 6.39±0.78 kg in the infants of treated mothers. A similar pattern was observed for infant length, with divergence from 6 months onward.
Supplementation studies have also been conducted in Caucasian populations. Devlin et al. randomized 40 women to either 1000 IU/day of vitamin D or no supplementation beginning at 6 months of gestation. All deliveries were in June. At the time of delivery, maternal and cord serum 25(OH)D concentrations were significantly greater in the supplemented women ( P <.001) than in controls; phosphate, PTH, and 1,25(OH) 2 D concentrations were not affected. The infants were evaluated at 4 days of age. Among the infants of control mothers the ionized calcium concentration and 25(OH)D and 1,25(OH) 2 D values were lower than in infants of supplemented mothers.
A study in Korean newborns assessing seasonal differences in whole body BMC and bone biomarkers of resorption provided early evidence that maternal vitamin D status affects neonatal bone acquisition . Infant weight and gestation did not differ according to season; however, winter newborn infants had markedly lower cord serum 25(OH)D and 1,25(OH) 2 D concentrations, higher concentrations of cross-linked C-terminal telopeptide of type I collagen (ICTP; a bone resorption marker), and 8% lower whole body BMC ( Fig. 36.7 ) than summer newborns. Infant whole body BMC was positively correlated with cord serum 25(OH)D values and negatively correlated with ICTP, which was also negatively correlated with 25(OH)D. Maternal and newborn 25(OH)D concentrations were highly correlated ( r =0.81, P <.0002). Mean calcium concentrations were lower in summer newborns than in winter newborns. In contrast, maternal serum calcium concentrations were higher in summer. These findings are consistent with poor maternofetal vitamin D status in winter, and the low whole body BMC in winter newborns is consistent with high bone resorption related to seasonally induced changes in vitamin D status. The seasonal effect (8% differential) on BMC was markedly greater than the seasonal difference (<2%) in BMC or BMD in adults . The Maternal Vitamin D Osteoporosis Study (MAVIDOS), a multicenter, double-blind, randomized placebo-controlled trial, recruited pregnant women from Southampton, Oxford, and Sheffield, United Kingdom and randomly assigned 565 women to 1000 IU/day and 569 to placebo. In this study, no overall effect of maternal supplementation during pregnancy was observed on offspring whole body BMC that was assessed within 2 weeks of birth . In a prespecified secondary analysis an interaction was seen for treatment and season of birth. For births in winter months, neonatal BMC, BMD, bone area, and body fat were greater among offspring of mothers supplemented with vitamin D. The results of the MAVIDOS childhood follow-up study at 6–8 years are awaited.
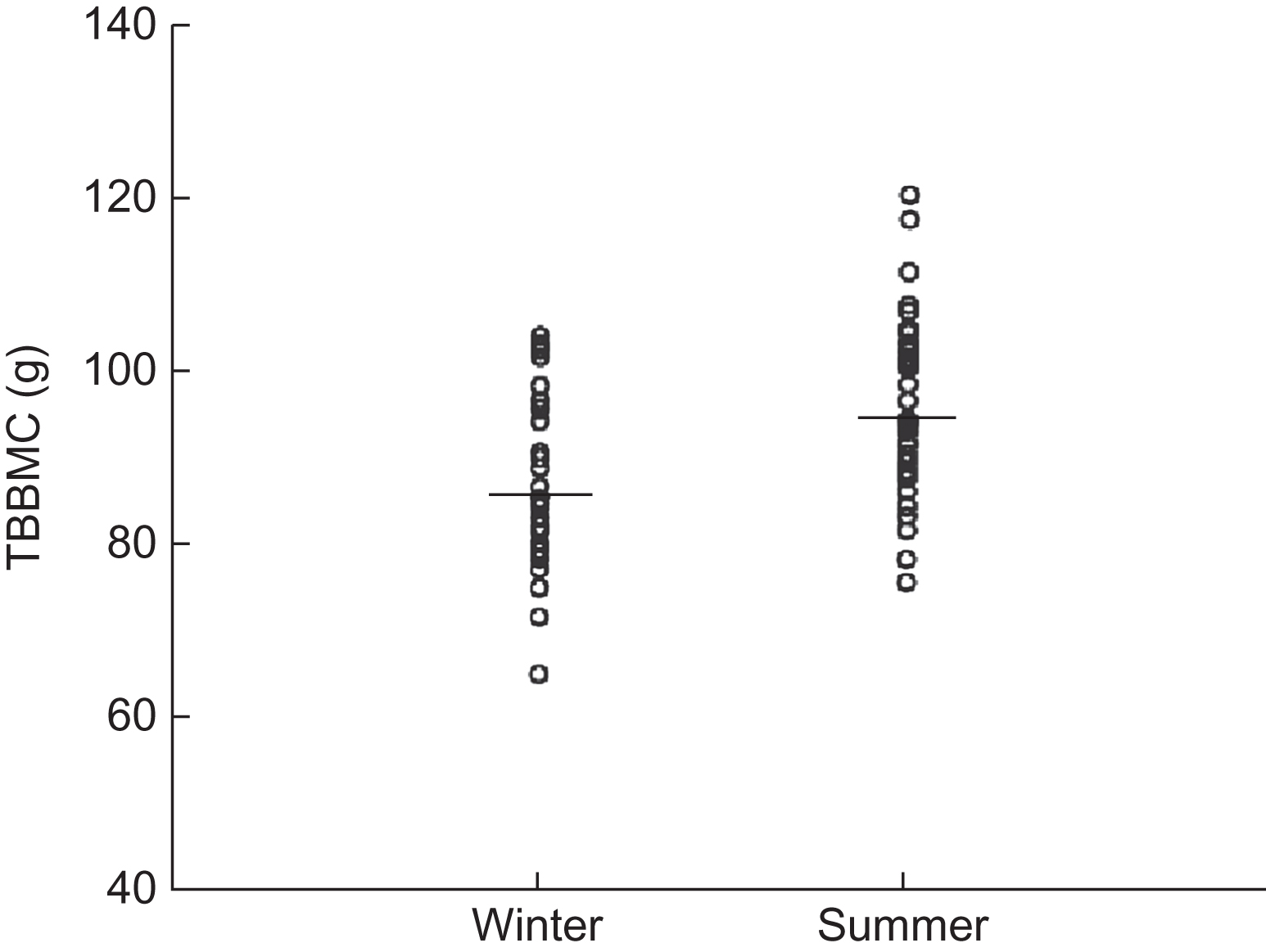
The relationship between maternal vitamin D status during pregnancy and childhood skeletal development by 9 years of age has been explored in other cohorts . Using ultraviolet B (UVB) exposure over the last trimester of pregnancy as a proxy measure for maternal vitamin D levels, Sayers and Tobias found positive associations between maternal UVB exposure and BMC, BMD, and bone area, but not area-adjusted BMC at 9.9 years of age in approximately 7000 subjects from the ALSPAC cohort, suggesting an effect on bone size and maternal vitamin D status on childhood periosteal bone formation . However, childhood bone mass was not related to maternal 25(OH)D levels in a sub-group of 355 ALSPAC mothers. An earlier study looking at maternal diet in pregnancy also showed no relationship between maternal vitamin D intake as assessed by food frequency questionnaire at 32 weeks gestation and total body DXA measures at 9 years of age . In a longitudinal study of 198 children recruited from a prior study of maternal nutrition and fetal growth in Southampton, United Kingdom, the body build, nutrition, and vitamin D status of the mothers had been characterized during pregnancy. During late pregnancy, 49 (31%) mothers had insufficient (11–20 ng/mL) and 28 (18%) had deficient (<11 ng/mL) 25(OH)D concentrations. Nine years later, the DXA scans were obtained in the children. Reduced 25(OH)D in mothers during late pregnancy was significantly associated with reduced whole body and lumbar spine BMC in children at age 9 years. Both the estimated UVB radiation exposure during late pregnancy and the maternal use of vitamin D supplements significantly predicted maternal 25(OH)D concentration and childhood BMC. Reduced umbilical venous calcium also predicted reduced childhood BMC, independent of albumin, phosphate, alkaline phosphatase, or creatinine concentrations. The authors concluded that maternal vitamin D insufficiency is associated with reduced bone mineral accrual in offspring during childhood, and that this association is mediated partly through umbilical venous calcium. Therefore vitamin D supplementation of pregnant women, especially during winter months, could lead to long-lasting reductions in their offspring’s risk of osteoporotic fracture. On the other hand, a prospective multiethnic population-based cohort study embedded within the Generation R Study (Rotterdam, Netherlands) showed an association between severe maternal 25(OH)D deficiency (<25 nmol/L) during mid-pregnancy and higher offspring BMC and larger bone area at age 6 years compared with maternal 25(OH)D>50 nmol/L. These associations were no longer significant after adjustment for childhood 25(OH)D status though, suggesting that vitamin D concentrations during childhood may be more relevant for bone outcomes than fetal 25(OH)D concentrations .
Maternal smoking and ethanol consumption
Maternal smoking has adverse bone effects in neonates and children . Possible mechanisms include impaired placental function, reduced uteroplacental blood flow, effects on fetal oxygen carrying capacity, and toxic effects of the heavy metal cadmium . Cadmium has specific adverse effects on osteoblast function and trophoblast calcium transport . In the prospective population-based Southampton cohort study, newborn whole body BMC and BMD were not related to maternal smoking at the time of the last menstrual period; however, whole body BMC and BMD were significantly lower in the infants of women who continued to smoke during pregnancy . After adjustment for gestation at birth the whole body BMC of the infants whose mothers smoked during pregnancy averaged 7.1 g (11%) lower than the whole body BMC of unexposed infants. However, their birth weights were lower by 306 g, on average. Whole body bone mineral apparent density (BMAD) was not related to smoking, suggesting that the reduction in BMC is commensurate with the reduction in body size. Neonatal spine BMC and BMD were not related to maternal smoking before or during pregnancy. Similarly, the Southampton Women’s Survey found that late pregnancy smoking was independently associated with lower neonatal whole body BMC, but there were not statistically significant relationships with estimated volumetric density .
Another population-based longitudinal study examined the relations between maternal smoking during pregnancy and bone mass in 330 Tasmanian children at 8 years of age . Maternal smoking during pregnancy was associated with significantly lower height and marginally lower weight in childhood, as well as disproportionately lower bone mass. That is, those children whose mothers smoked during pregnancy had significantly lower size-adjusted bone mass in the lumbar spine and hip. Further adjustment for placental weight led to nonsignificant results for smoking with both bone and growth parameters, suggesting that these associations were mediated through placental size and function. Maternal smoking at the time of the assessment of bone mass in the 8 year old children was not associated with childhood bone mass at any site. Therefore the timing of the exposure is critical, with detrimental effects in the offspring observed following maternal smoking during pregnancy. However, this long-term negative association between maternal smoking during pregnancy and bone mass in prepubertal children was no longer present at age 16 years . When 415 adolescents from the same birth cohort were examined, no association with maternal smoking and BMD was seen. This attenuation of effect in the same cohort of children suggested that the smoking effect on bone is transient and may disappear during puberty. At age 16 years, girls whose mothers smoked during pregnancy were shorter; those whose mothers breast-fed had higher bone mass and lower fracture risk.
Chronic heavy alcohol consumption is associated with osteoporosis in adults, with decreased bone mass and strength mainly due to a bone-remodeling imbalance with a predominant decrease in bone formation . A number of direct and indirect mechanisms responsible for alcohol-induced skeletal effects have been described (reviewed in Refs. ), including changes to osteoblast and osteoclast number and activity, increased osteocyte apoptosis, Wnt signaling suppression due to increased oxidative stress and upregulation of DKK1, a Wnt pathway antagonist, changes to cell differentiation associated with increased adipogenesis in bone marrow, and indirect effects of alcohol consumption through decreased calorie intake and body composition changes.
Published data on maternal ethanol consumption effects on bone health in offspring are limited to animal studies that demonstrate consistent deleterious fetal bone development effects. Keiver et al. reported that pregnant ethanol-fed rats developed low maternal blood ionized calcium concentrations and elevated PTH concentrations compared with controls; serum 1,25(OH) 2 D concentrations did not differ. The authors concluded that maternal ethanol consumption compromised the ability of the mother to regulate her blood calcium concentrations, possibly partly due to a failure to increase 1,25(OH) 2 D production. Keiver et al. and others have also shown that prenatal ethanol exposure decreases fetal body weight and bone length and delays skeletal ossification in the rat in a site-specific manner. Simpson et al. reported that prenatal ethanol exposure had effects on fetal skeletal development that were independent of those on overall fetal growth, and more severe effects occurred in the bones that undergo a greater proportion of their development in utero, suggesting that ethanol’s effects on bone are not limited to decreasing longitudinal growth. Furthermore, the effects of prenatal ethanol exposure persist into postnatal life, resulting in growth plate abnormalities , and decreased skeletal maturity scores at 2–4 weeks of age in rats . Given that delayed bone age and poor growth are established complications of fetal alcohol syndrome , these animal data are likely relevant to humans.
36.3
Bone acquisition in the preterm infant
Following delivery, the neonate must rapidly adjust mineral homeostasis regulation since the placental calcium infusion and source of PTHrP is lost. In humans, the neonate continues to accrete skeletal calcium at a rate of approximately 150 mg/kg/day, similar to the rate of the late-term fetus. Therefore the neonate depends on intestinal calcium intake, skeletal calcium stores, and renal calcium reabsorption to maintain normal blood calcium concentrations in the setting of continued rapid skeletal growth. In contrast to the fetus the newborn infant is more reliant on PTH and 1,25(OH) 2 D, and PTHrP is relatively less important for neonatal calcium and mineral homeostasis. For example, vitamin D deficiency or loss of function of the VDR (which has no or minimal effect on mineral homeostasis of the fetus) becomes obvious postnatally because of the dependence on intestinal calcium transport for supply of calcium, though in the first few weeks of life intestinal calcium absorption in newborns occurs by facilitated diffusion and is not vitamin D dependent .
36.3.1
Postnatal nutrition and physical activity
Because most fetal bone is acquired in the third trimester, one of the major factors affecting bone mass at birth is gestational age. Premature infants are prone to develop metabolic bone disease of prematurity precipitated by the loss of the placental calcium pump at a time when bone mineral acquisition is at its peak rate. This disorder is a consequence of inadequate calcium and phosphate intake to meet the mineralizing neonatal skeleton’s demands. Although intrauterine calcium retention during the last trimester averages approximately 140 mg/kg/day , calcium retention from standard enteral nutrition in the preterm infant is limited to only 70–80 mg/kg/day . Accordingly, daily calcium retention in the premature infant is only half that seen in utero, and BMC in premature infants at 40 weeks postconceptional age is significantly lower than BMC in term infants .
Special oral or parenteral formulas for premature infants that are high in calcium and phosphorus content have been developed to correct the demineralization process and to allow normal skeletal mineral accretion. An early study demonstrated that the supplementation of infant formula to provide a daily calcium intake of 220–250 mg/kg/day and a daily phosphate intake of 110–125 mg/kg/day in infants of 33–35 weeks’ gestational age resulted in a bone mineralization rate that approximated the intrauterine bone mineralization rate. Subsequently, the effect of mineral supplementation of human milk or infant formulas has been tested in several randomized clinical trials, with conflicting results. For example, Gross et al. randomized 50 healthy preterm infants to unsupplemented human milk (providing 40–50 mg/kg/day calcium and 23–30 mg/kg/day phosphorus), human milk mixed with a high mineral-containing formula (130 mg/kg/day calcium and 68 mg/kg/day phosphorus), or human milk mixed with a powdered fortifier (160 mg/kg/day calcium and 90 mg/kg/day phosphorus). Humeral BMC, determined by photon absorptiometry, did not differ across the three groups by 44 weeks postconceptional age. In contrast, Lapillonne et al. randomized 41 healthy, preterm infants (gestational age of 28–32 weeks) to either a control preterm formula or an isocaloric, nutrient-enriched preterm formula until 3 months of age (i.e., approximate expected term). Compared with control subjects, infants fed the experimental formula had 25% and 40% higher intakes of calcium and phosphorus, respectively. The BMC of infants fed the experimental formula was 23% and 35% higher at hospital discharge and expected term, respectively. A 2016 Cochrane Database Systematic Review reported that multinutrient fortification of human milk for preterm infants was associated with short-term improvements in weight gain and linear and head growth. A metaanalysis of data from five trials showed that the intervention group had statistically lower serum alkaline phosphatase levels, but substantial heterogeneity was present and there was limited evidence of an effect on BMC. Growth and developmental outcomes assessments beyond infancy were limited and showed no effect of fortification. A subsequent review of whether addition of calcium and phosphorus supplements to human milk leads to improved growth and bone metabolism of preterm infants revealed one trial conducted in Iran including 40 infants. There was no difference between human milk with calcium and phosphorus supplementation versus no supplementation for neonatal growth outcomes at 2, 4, or 6 weeks postnatal age. At 6 weeks, supplementation was associated with a small decrease in alkaline phosphatase; there were no data on fractures.
Lack of physical stimulation may contribute to metabolic bone disease of preterm infants and physical activity programs may enhance adequate nutrition in promoting growth and bone mineralization. In one of the earliest studies , 5–10 minutes of daily physical activity, consisting of range of motion with passive resistance to all extremities, in 26 preterm infants during a 4-week interval resulted in greater weight gain and radial BMC and BMD compared with controls. In a 2014 Cochrane Database Systemic Review , 11 trials were included, though all were small ( n =16–50) single-center studies. There was some evidence that physical activity programs might promote short-term weight gain and bone mineralization, but only one trial with 20 infants assessed long-term effects on bone. It showed no effect of physical activity administered during initial hospitalization at 12 months corrected age on whole body BMC or bone area. One trial with 16 participants reported on fractures and no fractures occurred in the treatment and control groups.
36.3.2
BMD and bone structure of former preterm infants
Investigators have examined BMC, BMD, and bone structure in children and adults who were born prematurely. A study of 25 former preterm girls, aged 7–9 years, reported lower areal BMD in the radial metaphysis, femoral neck, and total hip compared with controls . Differences persisted after adjustment for height and weight. Fewtrell et al. measured whole body and regional BMC and BMD by DXA at 8–12 years of age in 244 preterm children in a prospective randomized study of diet (human breast milk, preterm formula, and term formula) during the neonatal period. Preterm children were significantly shorter and lighter and had lower whole body BMC for age than their term peers. However, these differences disappeared after adjusting for bone area, body size, and pubertal status. Therefore the lower BMC was appropriate for the bone and body size achieved. Furthermore, comparison of the effects of the different diets in the neonatal period revealed no differences in BMC despite the major difference in early mineral intake. A follow-up analysis examined the associations between whole body and spine DXA results at 8–12 years of age and also weight and length at birth, 18 months, and 8 years of age . After adjusting for current size, there were significant negative associations between earlier size measurements and later whole body and lumbar spine BMC, which were stronger for length than for weight. Therefore among children of the same size in childhood, those who increased their size most, particularly in height, had the highest bone mass. When 220 of these same children were examined again at 20 years of age, the children were shorter, with greater BMIs, and lower spine BMD . Deficits were most pronounced in those who had been SGA at birth. Although infant dietary randomization was neither associated with peak bone mass nor bone turnover, those who had greater (>90%) intake of breast milk had higher whole body bone area and BMC than those who had <10% breast milk intake. These studies suggest both that nonnutritive factors in breast milk might enhance bone acquisition and that strategies to improve linear growth in vulnerable children may be important for maximizing bone acquisition.
Finally, Backstrom et al. examined bone structure and volumetric compartment density by peripheral quantitative computed tomography (pQCT) in 40 prematurely born but otherwise normally developed and healthy young adults (ages 18–27 years) and in 42 term-born adult controls. Body weight and height were similar in the two groups; however, the preterm group had significantly lower bone strength index (a composite measure of bone density and section modulus) at the distal tibia, distal radius, and tibial shaft. The lower bone strength index was largely due to smaller periosteal circumference, whereas trabecular and cortical compartment BMD did not differ between groups. Therefore prematurity was associated with small cross-sectional bone dimensions in young adulthood. The fracture implications of these deficits are not known.
36.4
Bone acquisition in term infants and children
Throughout childhood, skeletal mass increases through a combination of linear growth and changes in bone dimensions and density. The majority of studies characterizing age- and sex-specific patterns of axial and appendicular bone mineral acquisition during childhood and adolescence are based on DXA . Areal BMD and BMC increase steadily in the prepubertal years with growth and accelerate in both sexes after pubertal onset. However, DXA is limited in its ability to distinguish between differences in bone size, bone mass, and volumetric BMD in trabecular and cortical compartments. Furthermore, differing analytic techniques, variable adjustments of DXA results for maturation, body size, and body composition, and disparate study populations resulted in heterogeneity in findings of site-specific differences in bone mass according to sex. For example, some investigators reported greater femoral neck BMD in boys compared to girls , whereas others did not observe such differences .
Rauch and Schoenau proposed the following approach to the assessment of changes in volumetric BMD (mass per unit volume) during childhood and adolescence. Volumetric BMD is considered in three distinct levels. First, material BMD reflects the degree of mineralization of the organic bone matrix and is the amount of mineral divided by the volume of the bone matrix, excluding marrow spaces, osteonal canals, lacunae, and canaliculi. Second, compartment BMD is the amount of mineral divided by the volume of the trabecular or cortical compartments, including marrow spaces, osteonal canals, lacunae, and canaliculi, and it is a function of the material BMD and bone volume fraction. The trabecular compartment is defined as the space within the endocortical surface; the cortical compartment is limited by the periosteal and endosteal surfaces. Third, total BMD is the amount of mineral divided by the volume enclosed by the periosteal bone surface. These definitions are illustrated in Fig. 36.8 .
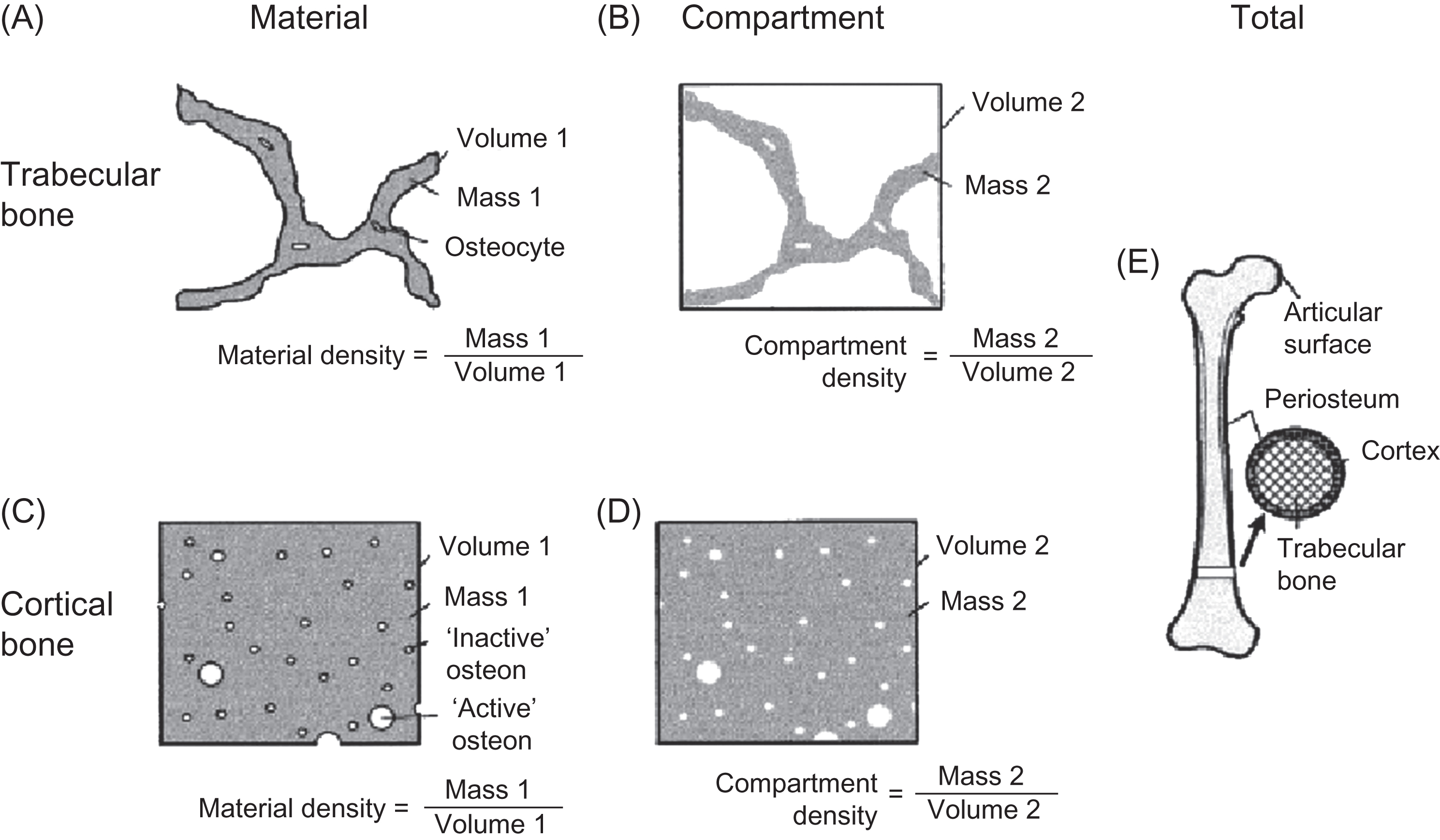
Material BMD cannot be determined with noninvasive densitometric techniques. As reviewed by Rauch and Schoenau , the relative ash weight of femur cortical bone specimens decreases after birth to a nadir in the first year of life and then increases thereafter . As trabecular bone turnover decreases from childhood to adulthood, iliac trabecular material BMD gradually increases from birth through adulthood .
Gilsanz et al. conducted QCT studies of trabecular and cortical compartment BMD and dimensions in children, identifying age-, maturation-, and sex-specific differences in the axial and appendicular skeleton. Prior to puberty, QCT estimates of trabecular volumetric BMD in the spine did not increase with age . The BMC increase with increasing vertebral volume (and stable BMD) was likely due to increases in trabecular thickness rather than trabecular number . Vertebral trabecular volumetric BMD did not vary according to sex or race prior to puberty; however, vertebral cross-sectional area was greater in boys at all pubertal stages . A study of the distal radial metaphysis trabecular compartment BMD by Neu et al. also demonstrated that BMD did not vary with age in prepubertal children; however, this study did not demonstrate the marked pubertal increase reported by Gilsanz et al. .
Densitometric studies of cortical compartment BMD changes with growth and maturation also produced varying results. A QCT study restricted to prepubertal children did not detect a variation in cortical compartment BMD with age in the femoral midshaft . However, a study using DXA (based on the assumption that the cortical diaphysis is circular in shape) detected significant increases in estimates of cortical compartment BMD in the femur . Studies based on direct bone specimen examinations reported that cortical compartment BMD decreases in the first few months of life and then increases until adulthood ; the majority of the increase occurring early in childhood.
Finally, changes in total BMD reflect changes in the trabecular and cortical compartment BMD and the relative volumes of these compartments. Changes in bone dimensions may result in decreases in total BMD, despite overall increases in bone mass . For example, in the first 6 months of life, cortical thickness decreases due to bone mass redistribution from the endosteal to periosteal surfaces with modeling . This results in a decrease in the proportion of the total bone cross-sectional area that consists of cortical bone and a precipitous decrease in total BMD despite significant BMC increases. During growth, total BMD rises significantly at sites where the relative cortical area (cortical area relative to total cross-sectional area) increases, such as the metacarpal bones, phalanges, and the forearm, but the increases are less evident in the femoral neck and midshaft . During puberty the cortical thickness increases since periosteal apposition is greater than endocortical resorption . Androgens preferentially promote periosteal apposition of bone, whereas estrogen at high concentrations stimulates endocortical bone gains . The net effect is a smaller bone size in girls than boys, despite similar cortical thickness .
36.4.1
Physical activity and the functional muscle–bone unit in children
If maximum bone mass meant maximum bone strength, evolution might have yielded solid bones without cavities . Instead, bone mass is distributed about a central axis to provide maximum strength with minimum material . The section modulus provides an estimate of resistance to torsional and flexural stress. Section modulus of an idealized cylindrical bone relates the periosteal radius (Rp) to the endosteal radius (Re) and is proportional to [(Rp 4 −Re 4 )/Rp], highlighting the exponential effect of small changes in bone dimensions on strength.
Bones can respond to increased mechanical loading by changing shape. This adaptive capacity is greatest during growth and is accomplished most efficiently in cortical bone by increasing cortical thickness, particularly at the periosteal surface. Trabecular bone is aligned with maximum stresses, resulting in variable strength along different axes (i.e., anisotropy) . Animal models have provided three critical rules governing bone adaptation to mechanical loading . First, loading stimulus frequency is critical; greater loading frequencies result in greater bone formation . Second, extended periods of loading do not produce greater bone accrual , but rest periods appear to increase bone mechanosensitivity . Third, bone cells accommodate to routine loading, but greater initial loading stimuli may produce greater gains in bone strength, even if the total loading stimulus is similar .
36.4.1.1
Observational studies of physical activity during childhood and bone mass
Unilateral loading models
Data from studies in childhood and adult elite racket sport and baseball players have provided significant insight into the structural effect of biomechanical loading and muscle forces on bone mass and dimensions. Kannus et al. examined side-to-side differences in humerus and radius BMC in 105 female Finnish tennis and squash players and 50 healthy female controls. The differences between the dominant and nondominant sides were 12.5%–15% in the racket sport players compared to 3.2%–4.6% among controls ( P <.001). However, the magnitude of the side-to-side differences was most pronounced in those who began playing the racket sport more than 5 years before menarche, in whom the BMC of the humeral shaft was almost 25% higher in the playing arm . In the subsequent 5 years, this population reduced the frequency of their training substantially without experiencing humeral or radial BMC decreases.
Kontulainen et al. used pQCT to identify the structural underpinnings of the greater BMC observed in the dominant upper extremity of 64 former elite female tennis or squash players and 27 matched controls. Long-term loading induced significant improvements in the density-weighted polar section modulus (a measure of bone strength) in the humeral shaft by periosteal expansion without changes in the endosteal dimension or cortical density. Again, the greatest differences were observed in those who began playing prior to menarche (i.e., “young starters”) ( Fig. 36.9 ). At the distal radius, trabecular volumetric BMD was greater in the dominant arm, but total area was greater only among those who began playing prior to puberty. In another pQCT study, Haapasalo et al. examined 12 adult former national-level male tennis players and demonstrated that exercise-induced bone gain was due to bone size enlargement without a change in volumetric BMD; however, no data regarding the effect of the training in the prepubertal years were provided.
