Penicillins and monobactams Cephalosporins Carbapenems Aminoglycosides Quinolones Tetracyclines Macrolides Glycopeptides, lipopeptides, and streptogramins Oxazolidinones Sulfonamides Metronidazole and clindamycin Rifamycins Polymyxins Miscellaneous agents Chloramphenicol Nitrofurantoin Pivmecillinam Fosfomycin Topicals |
Penicillins and monobactams
The core structure of the penicillins consists of a thiazolidine ring attached to a β-lactam ring and an R-group side chain. The thiazolidine–β-lactam ring provides antibacterial activity, while the side chain determines the antimicrobial spectrum and pharmacologic characteristics. Penicillins are bactericidal agents that inhibit penicillin-binding proteins (PBPs), which are involved in the synthesis of peptidoglycan. PBPs vary in their concentrations among bacteria and in their binding affinity for β-lactam antibiotics. This largely explains why β-lactam antibiotics differ in their efficacy and antibacterial spectrum. One type of PBP is β-lactamase, which hydrolyzes and inactivates the β-lactam ring. The penicillins may be conveniently grouped into the following classes: natural penicillins, penicillinase-resistant penicillins, aminopenicillins, and extended-spectrum penicillins. In addition, some of the penicillins have been combined with β-lactamase inhibitors including clavulanic acid, sulbactam, and tazobactam, which widen their antibacterial spectrum of activity.
The natural penicillins, penicillin G and penicillin V, are so named because they can be purified directly from cultures of the mold Penicillium. Penicillin G is unstable in acid and inactivated by gastric contents. It is administered by intramuscular, subcutaneous, intrathecal, or intravenous injection. Benzathine penicillin G, the treatment for primary, secondary, and latent syphilis, is slowly absorbed from tissue after intramuscular injection and detectable in serum for up to 30 days. However, levels are inadequate for treating neurosyphilis and intravenous formulations should be used. Most bacteria have developed resistance to the natural penicillins. Those that remain susceptible include Streptococcus pyogenes, viridans group streptococci, some enterococci, some Streptococcus pneumoniae, Listeria monocytogenes, Neisseria meningitidis, some Haemophilus influenzae, Clostridium (except Clostridium difficile), Actinomyces israelii, and Leptospira. Penicillin V is only available for oral use and treats most of the same organisms as penicillin G, although it is less active against Haemophilus and Neisseria. Nafcillin, a penicillinase-resistant penicillin, is active against methicillin-sensitive Staphylococcus aureus, penicillin-susceptible strains of S. pneumoniae, and most anaerobic gram-positive cocci. Importantly, nafcillin does not treat enterococcus and Listeria. Oral formulations of penicillinase-resistant penicillins include dicloxacillin (which is frequently used for skin and soft-tissue infections [SSTIs]) and cloxacillin. The aminopenicillins, ampicillin and amoxicillin, have improved activity against enterococci compared to penicillin G. Amoxicillin is better orally absorbed than ampicillin, while the latter is effective in treating meningitis due to L. monocytogenes, N. meningitidis, and group B streptococci. Ampicillin has been combined with the β-lactamase inhibitor sulbactam and is often given for mixed bacterial infections, such as intra-abdominal infections and obstetric and gynecologic infections. Amoxicillin–clavulanate is an oral formulation that is also given when multiple organisms need to be treated, such as animal or human bite wounds. The extended-spectrum penicillins include piperacillin, ticarcillin, and carbenicillin. Piperacillin is almost always given in combination with the β-lactamase inhibitor tazobactam. It has a wide range of activity including streptococci, anaerobes, enterococci, many Enterobacteriaceae as well as Pseudomonas aeruginosa. Piperacillin–tazobactam is utilized for many serious infections including hospital-acquired pneumonia, neutropenic fever, polymicrobial SSTIs, intra-abdominal infections, complicated urinary tract infections (UTIs), and often empirically for sepsis. Of note, use of piperacillin–tazobactam has been identified as a risk factor for Candida glabrata and Candida krusei fungemia. Ticarcillin–clavulanate is another parenteral broad-spectrum agent with similar indications as piperacillin–tazobactam, although less active against enterococci.
Monobactams consist of a single β-lactam ring with side chains. Currently the only commercially available monobactam is aztreonam. Monobactams are active exclusively against gram-negative aerobic bacteria. Aztreonam is not absorbed from the gastrointestinal tract and is usually given by the intravenous route. It is the only β-lactam that can be given to patients with allergies to penicillin or other β-lactam antibiotics since there is no cross-reactivity between them. An inhaled formulation of aztreonam has been developed for chronic use in cystic fibrosis patients with endobronchial P. aeruginosa infection.
Bacteria employ a number of resistance mechanisms against the penicillins and monobactams. The most common is the production of β-lactamase, which covalently reacts with and lyses the β-lactam ring. Four different classes of β-lactamases have been identified, designated A through D. Other mechanisms include efflux pumps which push penicillin out across the bacterial outer membrane, porins which do not allow passage of penicillins into the cytoplasm, and production of low-affinity PBPs.
Cephalosporins
The second major group of β-lactams, cephalosporins, are widely used in clinical practice. Currently there are over 20 cephalosporins available worldwide. They are composed of a β-lactam ring fused to a six-member dihydrothiazine ring. This structure bestows more intrinsic resistance against β-lactamases compared to the five-member ring of penicillin. Cephalosporins are commonly classified according to generation, with agents in each generation having a similar antibacterial spectrum of activity. Successive generations gain activity against aerobic gram-negative bacteria. Enterococci are intrinsically resistant to cephalosporins, although the new methicillin-resistant S. aureus (MRSA)-active cephalosporins have lower minimum inhibitory concentrations (MICs) against ampicillin-sensitive strains. The mechanism of action of cephalosporins is similar to other β-lactam agents, namely binding to and inhibiting PBPs, which in turn prevents peptidoglycan synthesis. Cephalosporins are bactericidal drugs and cause persistent suppression of bacterial growth for several hours, called post-antibiotic effect (PAE), in gram-positive bacteria, but not in gram-negative organisms. Their rapidity of bacterial killing is determined by the amount of time that the drug concentration exceeds the MIC, with maximal killing at four times the MIC.
The first-generation cephalosporins include cefazolin, cefadroxil, and cephalexin with the first administered parenterally and the latter two orally. Cefazolin is commonly used for infections caused by methicillin-sensitive staphylococci and streptococci, such as SSTIs, endocarditis from susceptible strains, and surgical prophylaxis for foreign-body insertion and other clean and clean-contaminated surgical procedures with a high risk for infection. The oral first-generation agents have good oral bioavailability and are effective for many SSTIs. They are often used to transition to oral therapy after parenteral cefazolin or in the outpatient setting. They are not active against H. influenzae or Moraxella catarrhalis and should not be used for respiratory infections. Second-generation cephalosporins are divided into two groups, the true cephalosporins (cefuroxime) and the cephamycins (cefoxitin and cefotetan). Cefuroxime was frequently used in the past for respiratory tract infections, but its poor activity against penicillin-resistant S. pneumoniae has now limited its effectiveness. The cephamycins have good activity against aerobic gram-negative and anaerobic organisms and are first-line agents for intra-abdominal, gynecologic, and mixed skin infections. The parenteral third-generation cephalosporins (ceftriaxone, cefotaxime, ceftazidime, and ceftizoxime) have a number of important clinical indications. Ceftriaxone and cefotaxime are active against penicillin-resistant pneumococcus and are recommended for treatment of community-acquired pneumonia (combined with azithromycin) and meningitis. Ceftriaxone is the drug of choice for gonococcal infections and is combined with doxycycline to treat pelvic inflammatory disease. Both ceftriaxone and cefotaxime are active against Borrelia burgdorferi and are effective for neurologic Lyme disease. Also, these agents have good activity against Enterobacteriaceae, although caution should be used with monotherapy against Citrobacter, Serratia, and Enterobacter species as they can develop inducible resistance through chromosomal β-lactamase production. Ceftazidime has excellent activity against P. aeruginosa but limited activity against S. aureus. Several oral third-generation cephalosporins are available (cefpodoxime, cefixime, cefdinir, and cefditoren) and are commonly prescribed for respiratory infections, sinusitis, and otitis media. Currently the only available fourth-generation cephalosporin in the United States is cefepime. This drug has an enhanced spectrum of activity against gram-negative organisms including those with reduced sensitivity to third-generation drugs. Cefepime is recommended as monotherapy for patients with neutropenic fever, although it is often combined with an aminoglycoside. Ceftaroline and ceftobiprole are advanced-generation cephalosporins with broad gram-positive and gram-negative in vitro activity and comparable efficacy to vancomycin against MRSA. They are also active against ampicillin-sensitive enterococci but not against P. aeruginosa or Acinetobacter species. Ceftaroline has been approved in the United States to treat community-acquired pneumonia and SSTIs, including those caused by methicillin-sensitive and -resistant S. aureus.
Carbapenems
Among the most broad-spectrum antibiotics, carbapenems are often reserved for serious infections. There are four available agents in this class: ertapenem, meropenem, imipenem, and doripenem. Their chemical structure is slightly different from other β-lactams in that the sulfur is replaced by a methylene group and the ring contains a double bond. Imipenem is a substrate for the kidney enzyme dehydropeptidase-1. It is coadministered with cilastin, a dehydropeptidase-1 inhibitor. Carbapenems have a similar mechanism of action, i.e., binding to high-molecular-weight PBPs, and are not hydrolyzed by most penicillinases. All have excellent activity against many gram-positive cocci. Penicillin-susceptible Enterococcus faecalis is susceptible to imipenem with MICs less than or equal to 2 μg/mL but resistant to the other carbapenems. Enterococcus faecium is resistant to all carbapenems, as are methicillin-resistant staphylococci. Neisseria species, Haemophilus species, and Enterobacteriaceae are all highly susceptible to carbapenems. Doripenem is the most active against P. aeruginosa, while ertapenem is inactive. Ertapenem also has poor activity against Acinetobacter. Stenotrophomonas maltophilia and Burkholderia cepacia are intrinsically resistant to all four carbapenems. As a class, carbapenems are highly active against anaerobic bacteria.
Because of their activity against many gram-positive, gram-negative, and anaerobic bacteria, carbapenems treat a wide range of infections. Moreover, they are often a good choice for patients who have recently received other antibiotics or for empiric coverage of severe sepsis. From an antibiotic stewardship perspective, carbapenems are advantageous since they can replace multiple antibiotics with one drug. For instance, ertapenem, with its long half-life that permits once-daily dosing, is often given to treat polymicrobial infections. However, the convenience of carbapenems must be carefully balanced against the potential for the emergence of resistance. Adverse events are similar to other β-lactams, although as a class there is an increased risk for seizures, particularly with imipenem.
Resistance to carbapenems usually occurs through overproduction of efflux pumps, altering PBPs, diminished outer membrane permeability, or production of β-lactamases. Several species of Enterobacteriaceae carry the plasmid-borne carbapenemases KPC-1, KPC-2, and KPC-3 which are capable of degrading carbapenems. P. aeruginosa also has a distinct efflux pump that is capable of removing numerous antibiotics, including meropenem, doripenem, and ertapenem but not imipenem. The recently emerged carbapenemase NDM-1 (New Delhi metallo-β-lactamase-1) has quickly spread worldwide. It is a class B carbapenemase (also called a metallolactamase) that requires zinc at its active site.
Aminoglycosides
The first aminoglycoside to be produced was streptomycin in 1944. It was followed by neomycin, then gentamicin, tobramycin, and lastly amikacin in 1972. They are bactericidal agents that exhibit concentration-dependent killing. Aminoglycosides bind to the 30S subunit of the prokaryotic ribosome, preventing protein synthesis from mRNA. This occurs through penetration of the bacterial cytoplasmic membrane by an oxygen-dependent active transport mechanism. Because of this action, aminoglycosides work poorly in anaerobic environments such as abscesses. These agents demonstrate a significant PAE, which increases with the peak serum concentration. Aminoglycosides are rarely used as single agents and are usually combined with another antimicrobial, such as a β-lactam or vancomycin. This helps prevent the emergence of resistance and gains the benefit of synergy. In cases of endocarditis, gentamicin should be administered using multiple daily dosing. However, for other types of infections there are several advantages of once-daily administration over multiple daily dosing. Animal studies have shown a lower rate of drug-related toxicities as well as a more robust PAE with once-daily dosing. Studies in humans have also demonstrated that once-daily dosing is efficacious, causes less nephrotoxicity, and is more cost-effective compared to multiple daily dosing regimens. The toxicities of aminoglycosides are well established and include nephrotoxicity (incidence 5%–10%), ototoxicity, vestibular toxicity, and neuromuscular blockade. Therefore, these agents should be used cautiously in patients with myasthenia gravis, electrolyte abnormalities such as hypocalcemia and hypomagnesemia, or with concurrent drugs that interfere with neuromuscular transmission, such as calcium channel blockers. Renal failure associated with aminoglycosides is usually reversible and often resolves once the drug is discontinued. Ototoxicity is more likely to be permanent.
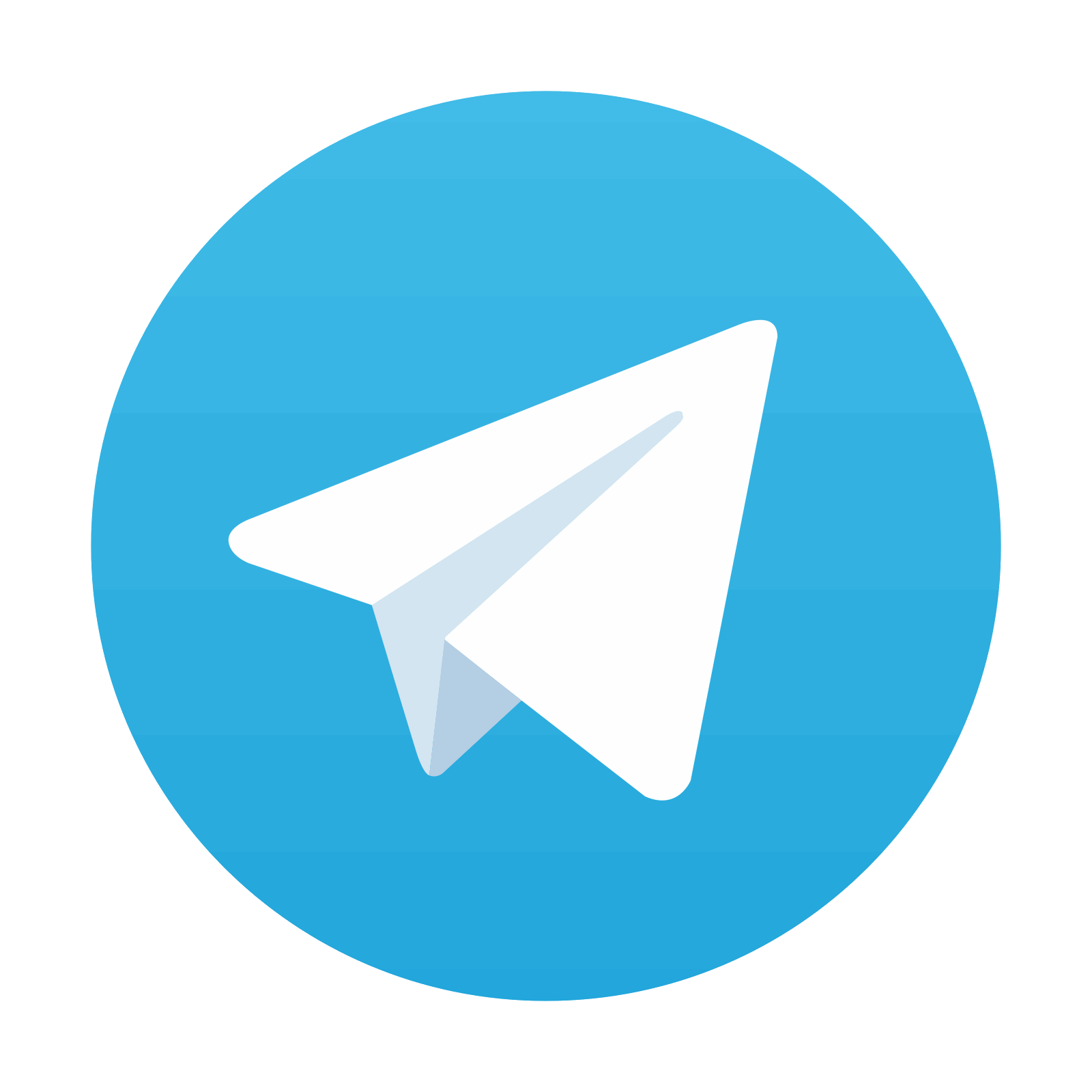
Stay updated, free articles. Join our Telegram channel

Full access? Get Clinical Tree
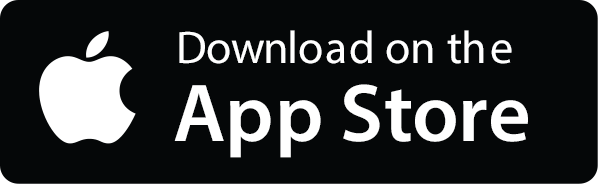
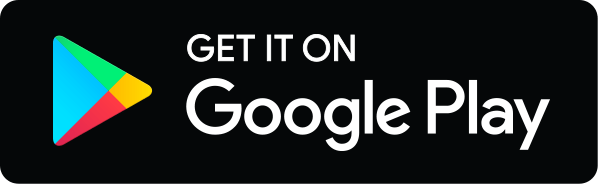