Fig. 1
Megakaryopoiesis and thrombopoiesis. Differentiation from hematopoietic stem cells (HSCs) to platelets proceeds by a number of intermediate stages. Megakaryopoiesis contains stages from HSC to mature MKs in the bone marrow. Thrombopoiesis contains stages in which mature MKs form cytoplasmic protrusion, called proplatelets, into a vascular lumen to produce platelet progenitors and mature platelets. Abbreviations: BM bone marrow, HSC hematopoietic stem cell, CMP common myeloid progenitor, MEP megakaryocyte-erythroid progenitor, MKP megakaryocyte progenitor, MK megakaryocyte, CLP common lymphoid progenitor, GMP granulocyte-macrophage progenitor, EP erythroid progenitor
1.2 Thrombopoietin
Nearly 1 × 1012 platelets, with a typical average life span of 7–9 days, circulate in the adult human bloodstream [7]. Nevertheless, the platelet number is tightly controlled within narrow physiological ranges [8]. The interaction between thrombopoietin (TPO) and its receptor, myeloproliferative leukemia virus protein (Mpl), is implicated in the proliferation and differentiation of normal HSCs and the bipotential MK precursors [7]. Mice lacking Mpl or JAK2 expression in platelets and MKs show expansion of MK and thrombocytosis, suggesting that TPO/Mpl signaling in MKs is dispensable for platelet production [9, 10]. TPO is produced in the liver hepatocytes [11]. MKs and platelets regulate TPO homeostasis by Mpl receptor-mediated internalization and degradation. Recently, a new feedback mechanism regulating TPO homeostasis was proposed in which the following occur: (1) as platelets age in the circulation, they become desialylated (lose of surface sialic acid); (2) hepatocytes recognize desialylated platelets via the Ashwell-Morell receptor (AMR); and (3) in hepatocytes, AMR signaling leads to JAK2-STAT5 phosphorylation, which leads to upregulation of TPO mRNA expression and subsequent TPO production [12].
1.3 Regulation of Transcription Factors
It has been reported that several transcriptional factors regulate the fate of MK development from HSCs. RUNX-1 functions as a master regulator of hematopoiesis and plays a role in lineage decisions in MEPs by repressing erythroid gene expression via epigenetic repression of the erythroid master regulator KFL-1 [13]. Moreover, mice lacking the transcription factors GATA-1 [14], AML-1 [15], or Fli-1 [16] show markedly reduced platelet numbers, associated with deregulated proliferation and severely impaired cytoplasmic maturation. These transcription factors are thus required for MK maturation in adult hematopoiesis. In addition, GATA-1 is required to cooperate with p45 for normal megakaryopoiesis [17]. Furthermore, NF-E2 is a heterodimeric transcription factor consisting of p45 and small Maf subunits [18–20]. Mice lacking NF-E2 show profound thrombocytopenia and fail to produce proplatelets, the microtubule-based precursors of blood platelets, from MKs [18–20]. The expression of beta1 tubulin is exquisitely restricted to platelets and MKs, where it appears late in differentiation and localizes to microtubule shafts and coils within proplatelets. Restoring NF-E2 activity in NF-E2-deficient MKs rescues the expression of beta1 tubulin [21]. These data suggest that NF-E2 determines the quantity and quality of platelets by activating genes in MKs that mediate platelet production and function [22, 23].
1.4 MK Maturation
One characteristic cellular feature of MK development is endomitosis, a unique form of mitosis in which DNA is repeatedly replicated in the absence of nuclear and cytoplasmic division [24, 25]. Polyploidization, which is considered as incomplete mitosis that is aborted in anaphase, occurs via endomitosis [25]. MKs with polyploidization enable functional gene amplification and experience an increase in protein and lipid synthesis in parallel with cell enlargement [26]. MKs generate a variety of secretory granules, including α-granule, which acquire their molecular contents both from endogenous protein synthesis and by uptake and packing of plasma proteins [27]. Moreover, a demarcation membrane system (DMS) begins as invaginations of plasma membrane that develop into an extensive complex of cisternae and tubules distributed throughout the MK cytoplasm [28]. The DMS is thought to exist as a membrane reservoir for proplatelet and platelet membranes, and it has been proposed that the term “invagination system” may be more appropriate [29, 30] (Fig. 2c, e).


Fig. 2
Megakaryocytes in bone marrow niche. (a, b) Reconstructed 3D image of BM from FLK-1-EGFP mice stained with anti-GP1b. Green, megakaryocytes; red, sinusoid endothelial cells. (c, d, e) Transmission electron microscopy of BM from wild-type mice. Abbreviations: DMS demarcation membrane system, IVP intravascular protrusion, MK megakaryocyte, PT platelet territory, S sinusoid lumen, SEC sinusoid endothelial cell
2 Platelet Formation
2.1 Genesis of the Basic Concept
After commitment, differentiation, and maturation, MKs migrate from the osteoblastic niche to the vascular niche (Fig. 2a), where they mature and eventually form individual platelets in the bloodstream (Fig. 2b, d) [31]. Although knowledge has accumulated for over a century, the mechanism by which platelets form and are released from MKs remains controversial.
In 1906, Wright first suggested that platelets arise from bone marrow (BM) MKs on the basis of histological sections of BM; he further described the formation of platelets by the “pinching off” of small projections from MK protrusions or of longer segments of MK protrusions in the sinusoid vascular lumen [32]. After this discovery, there have been several aspects of platelet formation that have been studied (Fig. 3).


Fig. 3
Schematic view of platelet formation in each model. (a) Schema of MK in vascular niche. (b) PT model. (c) Early proplatelet model. (d) Recent proplatelet model. (e) Immature large fragment. Abbreviations: DMS demarcation membrane system, EC sinusoid endothelial cell, ECM extracellular matrix, MK megakaryocyte. Red line indicates microtubules
In the 1950s, the platelet territory (PT) model was developed using transmission electron microscopy (TEM); in this model, MK cytoplasm is divided by the DMS into future platelets, fracturing into platelets in the BM parenchyma [28, 33, 34]. This model is based on the notion that individual platelets are already delineated by DMS in the PT (Fig. 3b). Because MKs reside within the platelet agonist-rich extracellular matrix (ECM) [35], MKs are required to release nascent platelets into the sinusoid vascular lumen without activation by the ECM (Fig. 3a). However, the transport of nascent platelets from the extravascular site through the sinusoid endothelial cells to the vessel lumen has not been elucidated in the PT model [28, 33, 34]. In addition, there are no reports regarding existence of the marginal band composed of microtubule bundles, the cytoskeletal hallmark of definitive platelets [36, 37], in the territories of MK cytoplasm.
2.2 Proplatelet Theory
From the 1970s, morphological studies using scanning electron microscopy (SEM) have predicted that platelets or segments of proplatelets are released from MKs via proplatelets, which have a tandem array of platelet-sized swellings with a beaded appearance at the distal end [38, 39]. At that time, it was thought that proplatelet extensions were already delineated by the DMS in the PT and that proplatelet morphogenesis was accomplished in the PT (Fig. 3c).
In the 1980s, it was suggested that microtubules running parallel with the long axis of proplatelets (a cytoskeletal feature of proplatelets) have a role in either the formation or maintenance of proplatelets [40, 41]. In addition, it was proposed that the DMS functions as a reservoir to supply membrane elements to extend the proplatelet in what is known as the synonymized flow model [29]. After the discovery of TPO in the 1990s, studies using cultured MKs in vitro successfully demonstrated the cytoskeletal hallmark, i.e., the marginal band-like structure at the distal end of proplatelets [42–44]. These findings suggest that MKs remodel their cytoplasm into proplatelets that transport products and organelles in the appropriate abundance into the vasculature and release fragments of proplatelets (Fig. 3d). Thus, this has led to the proplatelet theory becoming the unifying and accepted model.
2.3 Dynamic Analysis of Platelet Formation in Native BM
There have been reports of many unclassified snapshots of proplatelet and nascent platelet morphogenesis in BM. For example, intravascular thick protrusions and fragments were reported in early histological studies [32, 45, 46], and they were considered as a sequential stage of proplatelet formation without further dynamic evaluation [29]. However, there were technical limitations regarding performance of temporal and spatial analysis of the sequential stages of platelet formation in living BM tissue.
In contrast, recent studies using multiphoton microscopy, which can visualize platelet formation from MKs in BM of living mice, necessitate a revision of these prior concepts. For instance, Junt et al. captured images of MKs forming large “proplatelet-like protrusions” which routinely released heterogeneous large particles into the sinusoids [47]. They concluded that these large particles might represent multiple intertwined or single immature proplatelets. However, it remained unclear whether all intravascular protrusions translate into proplatelets or whether MKs release cytoplasmic particles without proplatelet formation.
Our group analyzed MKs forming intravascular protrusions using intravital imaging, TEM, and SEM [48]. In analysis of intravital imaging, MKs formed proplatelet processes and released proplatelet fragments that were originally defined as thin cytoplasmic extensions forming a tandem array of platelet-sized swellings with a beaded appearance at the distal end [29, 38–41]. In addition, MKs formed a “thick protrusion,” released the cell fragments that preserve the abundant DMS and ER, and randomly organized microtubules; this implies that the fragments have not yet accomplished the intracellular remodeling that was seen in proplatelets. These data suggest that MKs have an ability to release contents of nascent platelets without proplatelet formation (Fig. 3e). Notably, this immature type of MK fragment release may be regulated by platelet demand.
Recently, Nishimura et al. reported rapid platelet release from the MK cytoplasm which they termed “MK rupture” [49]; in intravital images, this seems to represent platelet release as per both the PT model (Fig. 3b) and early proplatelet model (Fig. 3c); however, ultrastructural verification by TEM was not reported. MK rupture also seems to be associated with sinusoid vessel damage, because soon after this event, vascular tracer signals leaked into BM parenchyma. Interestingly, as the mechanism appears to be regulated by elevated levels of interleukin-1α, the authors proposed the importance of balance between TPO and interleukin-1α levels, which determines platelet production mechanism used by MKs.
2.4 Direct Stimulation of Proplatelet Formation
TPO stimulates MK precursors, but suppresses proplatelet formation from MKs in vitro [50–52]. In addition, TPO signaling in MKs is dispensable for platelet production in vivo [9, 10]. These findings indicate that other factors, including interleukin-1α, regulate platelet production from BM MKs. It has been reported that one of the direct regulators of platelet production via proplatelet formation is the thrombin/antithrombin complex included in high-density lipoprotein [53]. Notably, both thrombin and antithrombin are included in the coagulation cascade associated with platelet consumption. Recently, CCL5, a member of the interleukin-8 cytokine superfamily, may have a direct stimulatory effect on platelet production by MKs by inducing more rapid platelet production than that accomplished through steady-state regulation via TPO [54, 55].
3 Platelet Progenitors and Nascent Platelets
Detached cell fragments from MKs are heterogeneous population of cells in regard to their size, shape, or structure in the circulating blood. Large fragments are regarded as platelet progenitors which have the ability to convert into mature platelets. Platelet progenitors are described in terms of barbell-shaped proplatelets [42, 56, 57], preplatelets [56, 58], and giant platelets [48]. After leaving the BM sinusoids, these platelet progenitors may convert into individual platelets in the bloodstream, especially in microcapillaries in the lung, which is the first capillary bed to be encountered [56–59].
In summary, megakaryopoiesis depends on the proliferation and differentiation of HSCs to be committed to the MK lineage and their maturation to large MKs. These processes are regulated by many different factors including cytokines, chemokines, and BM microenvironments. Platelets are formed from MK cytoplasm via their protrusions and fragments into the vessel lumen. Although these processes are biologically complex phenomenon, it has been becoming clear how individual platelets are formed and eliminated in the vessel lumen by recent researches.
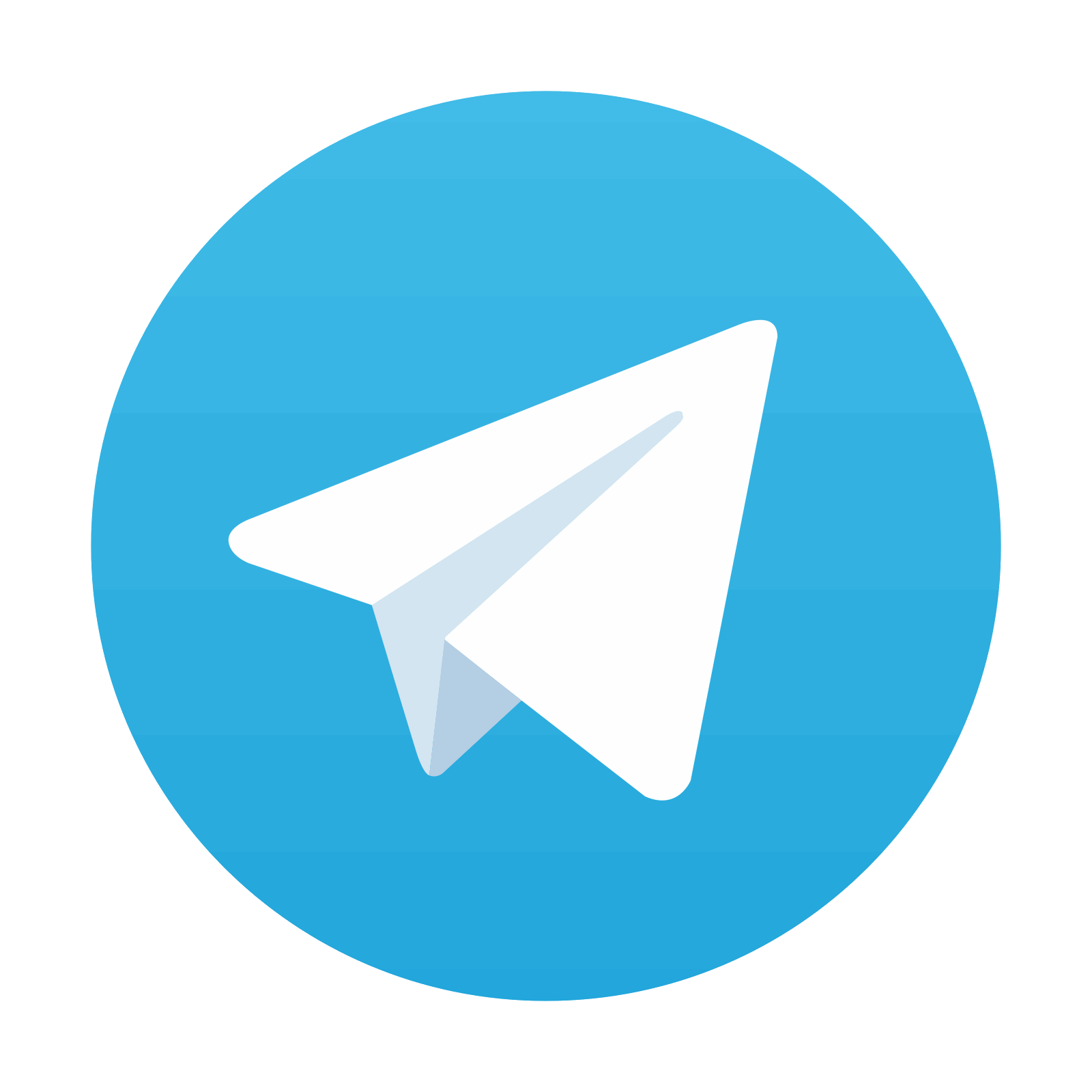
Stay updated, free articles. Join our Telegram channel

Full access? Get Clinical Tree
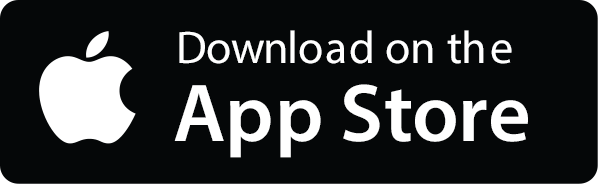
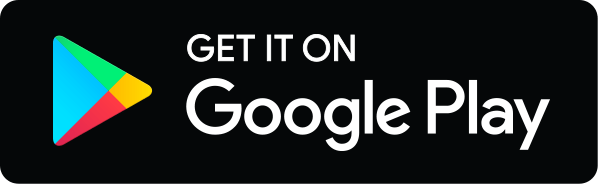