Surgical resection is the first-line treatment for selected patients with hepatobiliary malignancies. With refinements in surgical techniques and perioperative patient care, the safety of liver resection has improved dramatically over the decades. Indeed, the mortality rate after major hepatectomy has been reported to be less than 5% in recent years at high-volume hepatobiliary centers.1–3 The most important factor influencing outcomes after liver resection is the surgeon’s knowledge of the relevant anatomy and of basic principles pertaining to the surgical procedure. In this chapter, we review the general principles of hepatobiliary surgery and describe current approaches for safe liver resection.
Adequate surgical planning is required for safe and curative resection of hepatobiliary malignancies. Preoperative assessment should include evaluation of the resectability of the disease and prediction of surgical risk, which allows surgeons to minimize perioperative morbidity in patients undergoing liver resection.
Imaging studies are used to detect and characterize hepatobiliary lesions. For liver lesions, computed tomography (CT) and magnetic resonance imaging (MRI) have been reported to be the best methods of detecting lesions.4–6 Contrast enhancement with a quadruple-phase liver protocol (i.e., plain CT followed by a three-phase dynamic study comprising an arterial phase, a portal phase, and a late phase) can be used to accurately evaluate the extent of the disease and characterize tumor vascularity for precise mapping of lesions and accurate diagnosis. For biliary lesions, high-resolution CT also accurately predicts resectability.7 However, endoscopic retrograde or percutaneous transhepatic cholangiography is helpful for determining the longitudinal distribution of such lesions, permitting sampling of bile for cytology as well as step biopsy, intraductal ultrasonography, and/or biliary drainage simultaneously.
In addition to assessment of the main hepatobiliary lesions, screening of distant metastases or extrahepatic disease is also inevitably required to determine the oncological resectability of the tumor. Chest CT is the preferred modality for identifying lung metastases. Patients with metastatic liver lesions from the gastrointestinal tract additionally require pelvic CT to exclude peritoneal dissemination and primary status or local recurrence of the primary. In selected patients, positron emission tomography combined with CT (PET-CT) is useful for screening distant metastases and determining the metabolic characteristics of an equivocal lesion detected by CT or MRI. However, routine use of PET-CT is not recommended owing to its cost, the need for local expertise, and its limited sensitivity in detecting small lesions and tumors treated with chemotherapy.8 Additionally, a recent randomized controlled trial showed no benefit of PET-CT in the preoperative assessment of patients with hepatic colorectal metastases compared to standard, high-quality CT imaging.9
To determine the technical resectability of the disease and permit an efficient surgical approach, anatomical characteristics should be evaluated in detail prior to surgery. In general terms, all tumors should be assessed based on Couinaud’s classification of the liver segment anatomy and proximity or involvement of major inflow pedicles and/or hepatic venous outflow. Preoperative evaluation may vary slightly based on tumor type (see below and also disease-specific chapters).
For hepatocellular carcinoma (HCC), anatomic resection of the tumor-bearing portal region is, theoretically, a reasonable approach and has been recommended to reduce the risk of local recurrence and eradicate micrometastases scattered from the main lesion via portal veins.10–12 However, limited resection with adequate surgical margins and major hepatectomy have been used for colorectal liver metastases (CLMs) because a 2- to 4-mm surgical margin has been shown to be required in order to encompass micrometastases around CLM lesions in histopathologic studies.13,14
Second, the relationship between the tumor and surrounding major vascular structures should be evaluated for each tumor. HCC usually grows in an expanding fashion (rather than in an invading fashion) with a thick capsule. Empirically, these tumors can be detached from the vessels without increasing the risk of local recurrence, even though huge HCC lesions will compress the inferior vena cava severely . In contrast, concomitant resection of the vessels should be considered in adenocarcinomas such as CLM and intrahepatic cholangiocarcinoma when a tumor is inseparable from adjacent major vascular structures. Although histopathologic invasion of the vessel wall is relatively uncommon on histopathologic examinations,15 adenocarcinomas lack capsules and easily invade blood vessel walls . Because it is difficult to predict whether histopathologic invasion will occur even after good response to chemotherapy (Fig. 124-1), en bloc resection of adjacent blood vessels with sufficient margins is recommended for adenocarcinomas.
FIGURE 124-1
A. A colorectal liver metastasis is originating in segment VIII of the liver and invading the middle hepatic vein. B. Tumor shrinkage is observed after six cycles of chemotherapy using mFOLFOX6 with panitumumab. However, the tumor is still inseparable from the vein. C. The histopathologic specimen confirmed microscopic invasion of the tumor into the wall of the middle hepatic vein (arrows). MHV: middle hepatic vein.

Third, once the relationship between the lesion and surrounding structures has been assessed, anomalies in vascular structures should be evaluated in detail because they may constrain the surgical procedure. Portal and hepatic venous systems are primitive structures formed at a very early stage in the development of the human liver. Therefore, they have less variation than do the hepatic artery or biliary tree. However, knowledge of major variations is needed to avoid anatomic errors during surgery.
The portal venous system’s ramification patterns at the hepatic hilum include important points of variation. In approximately 80% of patients, the main portal vein bifurcates into left and right branches at the hepatic hilum (known as the “bifurcation type”), whereas in the remainder of patients, trifurcation into the left, right paramedian, and right lateral pedicles (10% of patients) or early branching of the right lateral pedicle (10% of patients) is observed (Fig. 124-2). Although the number of cases is quite limited, right-sided ligamentum teres16 (0.6% to 1.2%), lack of a left portal trunk17 (0.9%), and lack of an intrahepatic portal vein18 (<0.1%) are sometimes encountered in preoperative CT imaging.
FIGURE 124-2
Typical ramification patterns of the portal vein. A. Bifurcation type; the main portal vein bifurcates into left and right branches at the hepatic hilum (80%). B. Trifurcation into the left, right paramedian, and right lateral pedicles (10%). C. Early branching of the right lateral pedicle (10%). LPV, left portal vein; RL, right lateral portal branch; RPM, right paramedian portal branch.

As for the hepatic veins, the drainage pattern of the right posterior sector (i.e., segments VI and VII) is clinically important because accessory right hepatic veins such as the middle right hepatic vein and inferior right hepatic vein are observed in nearly 70% of the cases, regardless of the size of accessory right hepatic veins (Fig. 124-3). Care should be taken when these veins are thick and drain large portions of segment VI or VII (Fig. 124-4) because ligation may cause congestion of the right posterior sector. Moreover, careless handling of the right hemiliver during mobilization may injure these veins incidentally, resulting in massive bleeding.
FIGURE 124-3
Accessory right hepatic veins. A. The right hepatic vein (dominant type) without major accessory right hepatic veins (33%). B. Thick inferior right hepatic vein (IRHV) draining segment VI (33%). C. Thick IRHV and middle right hepatic vein (MRHV) draining segment VII (33%). LHV, left hepatic vein; MHV, middle hepatic vein; RHV, right hepatic vein.

FIGURE 124-4
Accessory right hepatic veins during mobilization of the right hemiliver. The right hemiliver is fully mobilized and the right wall of the inferior vena cave is exposed. Thick accessory right hepatic veins are observed. IRHV, inferior right hepatic vein; MRHV, middle right hepatic vein; RHV, right hepatic vein.

The existence of important variations in hepatic arteries, such as the presence of “accessory” or “replaced” arteries, should also be ascertained. Normally, the right and left hepatic arteries are derived from the proper hepatic artery. However, two variations in the ramification of the left and right hepatic arteries are commonly seen (Fig. 124-5). First, the left hepatic artery sometimes branches from the left gastric artery, running through the lesser omentum and feeding segments II and/or III. Second, the right hepatic artery sometimes originates from the superior mesenteric artery, running behind the portal vein and reaching the right hemiliver. Aberrant arteries that provide the total arterial supply for the left lateral segments (segments II and III) or the right hemiliver are called “replaced” arteries (i.e., “replaced left hepatic artery” or “replaced right hepatic artery”). However, when arterial flow from the proper hepatic artery also presents, the aberrant arteries are called “accessory” arteries.
FIGURE 124-5
Typical anomaly in hepatic arteries. An accessory/replaced left hepatic artery derived from the left gastric artery or accessory/replaced right hepatic artery branched from the superior mesenteric artery is frequently observed. Patterns of arterial supply should be confirmed preoperatively using CT scan on arterial phase. CHA, common hepatic artery; GDA, gastroduodenal artery; LHA, left hepatic artery; PHA, proper hepatic artery; RHA, right hepatic artery; SMA, superior mesenteric artery; SMV, superior mesenteric vein; SpA, splenic artery; SpV, splenic vein.

As with the right and left hepatic arteries, the ramification pattern of the right-side branches of the biliary tree may vary considerably (Fig. 124-6). In contrast, the anatomy of left hepatic duct is rather constant. Thus, a right or extended right hepatectomy can be performed safely with fewer biliary tract complications than would be the case with a left hepatectomy. However, care should be taken to avoid incidental injury to the right-side branches when a left-side major hepatectomy is performed. Preoperative assessment using magnetic resonance cholangiopancreatography (MRCP) or intraoperative direct cholangiography through the cystic ductis sometimes required to confirm identification of the biliary branches of the future liver remnant (FLR). From an oncologic standpoint, anatomic variations in the biliary tree play a large role in determining the surgical procedure to be performed, especially in the case of hilar cholangiocarcinoma. In the treatment of hilar cholangiocarcinoma, the most feasible type of hepatectomy should be determined on the basis of tumor distribution (Fig. 124-7).19 Therefore, precise mapping and anatomic confirmation of the tumor extension are key factors in achieving curative resection.
FIGURE 124-6
Variations in biliary anatomy. The left hepatic duct is long and present in 97% of patients. In patients with types I, II, and IIIa hilar cholangiocarcinomas, an extended right hepatectomy permits placement of a single, safe anastomosis away from the confluence, minimizing the probability of positive margins. BLL, left lateral duct; BLPM, left paramedian duct; BRL, right lateral duct; BRPM, right paramedian duct; LHD, left hepatic duct.

Functional reserve of the liver depends on the quality of the liver parenchyma. The volume of the FLR20–24 and the regenerative capacity of the liver25,26 are important predictors of postoperative morbidity and mortality after extended hepatectomy. Because the risk of postoperative hepatic insufficiency or death from liver failure remains high after extended hepatectomy, accurate estimation of the functional reserve of the liver and preparation for an extensive surgical procedure are needed to secure the safety of hepatobiliary surgery for patients expected to have small FLR volume.
In patients with chronic liver disease, the functional reserve of the liver is primarily assessed using the Child–Pugh score. Postoperative mortality rates are higher for patients with higher Child–Pugh classifications, reaching nearly 50% for those with Child–Pugh class C.27 Therefore, only patients classified as Child–Pugh class A (with the lowest mortality rates) or selected populations classified as Child–Pugh class B are candidates for liver resection. In addition, because the presence of portal hypertension results in high morbidity and mortality rates, care should be taken when a patient presents clinical signs suggestive of portal hypertension such as ascites, splenomegaly, esophageal varices, or thrombocytopenia.
Evaluation of FLR volume is currently the most reliable means of predicting outcomes for patients who are candidates for major liver resection. At the University of Texas MD Anderson Cancer Center, we calculate the patient’s estimated total liver volume (TLV) using a formula that relies on the linear correlation between the TLV and body surface area (BSA): TLV (cm3) = −794.41 + 1,267.28 × BSA (m2). The standardized FLR (sFLR) is then calculated as the ratio of the FLR volume to the estimated TLV. In a large cohort study seeking optimal cut-off values for the minimum sFLR required to avoid postoperative hepatic insufficiency or death from liver failure, it was estimated that an sFLR ≥20% is needed for normal liver,20 although an sFLR ≥ 30% is needed for patients who have received extensive chemotherapy (i.e., ≥3 months) prior to surgery.22 For patients with liver cirrhosis, sFLR should be ≥40%. Current clinical evidence based on a large number of series is summarized in Fig. 124-8.
FIGURE 124-8
Minimum requirement of standardized future liver remnant according to the status of the underlying liver. Standardized future liver remnant (sFLR) ≥20% is needed for normal liver, although an sFLR ≥30% is needed for patients who have received extensive chemotherapy (i.e., ≥3 months) prior to surgery. For patients with liver cirrhosis, sFLR should be ≥40%.

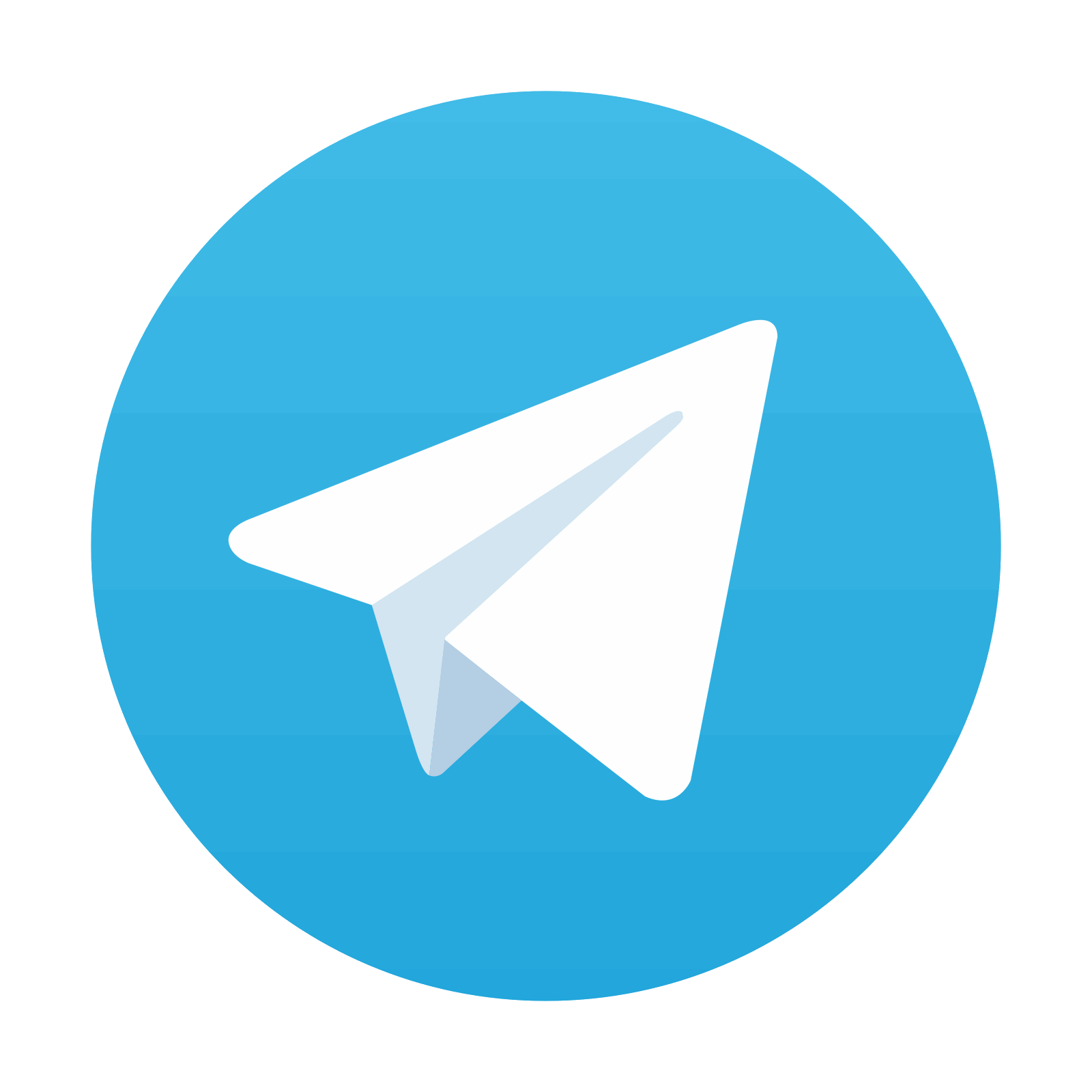
Stay updated, free articles. Join our Telegram channel

Full access? Get Clinical Tree
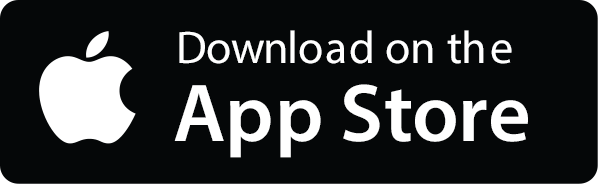
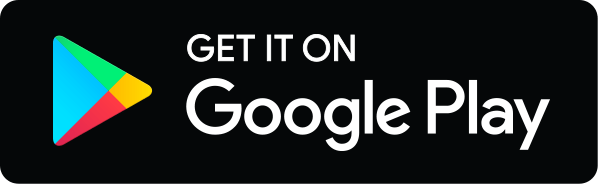