18 Alternative Radiotherapy Approaches
Although it is well established that radiotherapy has efficacy against brain tumors, it is also clear that standard radiotherapy has major shortcomings. Many brain tumors, including most malignant gliomas, recur or progress at some point following radiotherapy, and radiation dose and volume are limited by normal brain toxicity. This chapter describes alternative radiation approaches that have been investigated (mainly in malignant gliomas) to try to improve the results of radiotherapy: altered fractionation, particle therapy, radiation sensitizers, photodynamic therapy (PDT), boron neutron capture therapy (BNCT), brachytherapy, hyperthermia, and stereotactic body radiation therapy (SBRT) for spinal tumors.
Alternative Fractionation Schemes for Radiotherapy
Hyperfractionation
Hyperfractionation involves a larger number of smaller sized fractions to a total dose that is higher than with conventionally administered irradiation in the same overall treatment time. Normal glial and vascular cells limit the total amount of irradiation that can be administered. These cells divide very slowly and are better able to repair sublethal damage than are neoplastic cells. Consequently, there might be an advantage to administering multiple smaller sized fractions to a higher total dose, the theory being that the improved repair of sublethal damage at smaller sized fractions might allow a higher total dose to be associated with the same degree of late sequelae. Neoplastic cells are relatively rapidly dividing cells, and the increased number of daily fractions would increase the chance of radiating them at a more sensitive phase of their cell cycle. At smaller radiation doses per fraction, cell killing is less dependent on oxygen, which might be advantageous given the known areas of hypoxia in these tumors.
There have been six randomized studies of hyperfractionated radiotherapy compared with conventionally fractionated radiotherapy in patients harboring malignant gliomas.1 Five of six studies demonstrated no benefit on the experimental arm, and one study showed a survival advantage for the hyperfractionated arm.2 This latter study had a small number of patients per arm, and the median survival of 27 weeks for the conventionally fractionated arm was significantly worse than all other published data for conventionally fractionated radiotherapy. The largest study on hyperfractionation clearly showed no benefit for the use of hyperfractionated radiotherapy in 712 patients with malignant gliomas.3 In a pooled analysis of a systematic review of all published data on randomized studies of hyperfractionation in patients with malignant gliomas, no benefit of this approach was demonstrated.1
Special Consideration
• Despite a sound radiobiological rationale, hyperfractionated radiotherapy allowing higher total doses to tumor with apparent equivalent effects on normal brain has not resulted in improved survival in patients with malignant gliomas.
Accelerated Fractionation
The aim of accelerated fractionation is to reduce overall treatment time in an effort to reduce the possibility of tumor repopulation during treatment. This is achieved by delivering two or three normal-sized fractions per day. Accelerated fractionation has been evaluated in a randomized study conducted by the European Organization for Research on Treatment of Cancer (EORTC) in patients with malignant glioma.4 In protocol 22803, 340 patients were randomly assigned to conventional radiotherapy or accelerated fractionation with or without misonidazole. Accelerated fractionation consisted of three fractions of 2 Gy per day with a 4-hour gap between fractions to deliver 30 Gy in 1 week. This treatment course was repeated after a 2-week break for a total of 60 Gy in 30 fractions in 4 weeks. There was no difference in survival among the three treatment groups and no increased toxicity with accelerated radiation.
In a randomized phase II dose-escalation study (Radiation Therapy Oncology Group [RTOG] 83–02), a subgroup of 305 patients received 1.6 Gy twice daily to total doses of 48 or 54.4 Gy.5 The results demonstrated no significant survival difference among all dose schemes, and there was a low toxicity rate with accelerated fractionation. A single-arm study of accelerated radiation was reported in 211 patients with malignant astrocytomas.6 Radiation treatment consisted of 55 Gy in 34 fractions (twice daily) delivered to the enhancing tumor and a 3-cm margin. Median survival was 10 months, which was similar to a matched cohort of patients who had received 60 Gy in 30 fractions over 6 weeks. Two other small studies also found no improvement in survival or increased toxicity with accelerated fractionation schemes in malignant glioma.7,8 One study evaluated 40 Gy in 20 fractions in 1 week as part of a randomized phase 2 study,8 whereas the other evaluated 60 Gy in 16 days using a single-arm phase 2 design.7
• Patients with glioblastoma multiforme are not the best population to assess the long-term effects of new fractionation schemes because of their short survival. It is possible that some of the hyperfractionated or accelerated schemes tried in this population would be associated with significant long-term complications if patients survived longer.
A single-arm study was reported in which 10 patients with primary lymphoma of brain received 50 Gy delivered in 25 fractions of 2 Gy given twice a day with a minimum 6-hour gap between fractions.9 Treatment was delivered on weekdays and weekend days, such that the total treatment (50 Gy) was delivered in 13 consecutive days (Fig. 18.1). There was no improvement in survival, with a reported median survival of 17 months. There was some evidence of increased toxicity, with autopsy evidence of radiation necrosis in the pons in one patient at 5 months. There was one long-term survivor at 69 months with evidence of radiation retinopathy and an undiagnosed degenerative neurologic condition.
Particle Therapy
Particle therapy refers to the use of subatomic particles as a form of treatment as opposed to photons. These particles include neutrons, protons, helium ions and heavier nuclei (carbon ions), and negative pi mesons (pions). The use of these particle beams offers two possible advantages over the use of photons: better physical dose localization to the tumor volume and greater biological effect.
Fast neutrons are usually produced in a cyclotron and at higher energies than the spectrum of energies associated with neutrons produced in a nuclear reactor; these latter neutrons are referred to as slow or thermal neutrons. Fast neutrons that have been studied have depth dose characteristics similar to those of a cobalt unit, and as such do not offer any improved dose localization effect but have been studied predominantly for their possible biological advantages over photons. Five randomized trials have evaluated particle therapy for gliomas.10–14 None of these trials detected a significant survival benefit for particle therapy. The first four studies looked at neutrons, and the fifth study randomized 81 patients to either 60 Gy in 30 fractions with photons or pion therapy to 33.0 to 34.5 Gy where the median survival was 10 months in both groups.14
In the randomized, dose-searching study by the RTOG, autopsies were performed on 35 patients at all dose levels.13 Some patients had both radiation damage to normal brain tissue and evidence of viable tumor. No evidence was found for a therapeutic window using this particular treatment regimen. Autopsies performed in the earlier RTOG study revealed actively growing persistent tumor in all photon-treated patients compared with no evidence of actively growing tumor in the majority of neutron-treated patients.12 In one study all patients who died had evidence of residual brain tumor.11 None had signs of radiation-related morbidity. The subsequent trial by the same group was discontinued prematurely as a result of neutron morbidity.10 In this study, four of nine patients treated by neutrons had evidence at autopsy of radiation-induced brain damage and all had residual malignant glioma.
Fig. 18.1a,b (a) Axial T1-weighted gadolinium magnetic resonance imaging (MRI) of a 40-year-old man with a primary lymphoma of brain. (b) Axial T1-weighted gadolinium MRI of the same patient 1 month after having received accelerated radiation therapy of 5,000 cGy in 13 days, demonstrating a near-complete response.
• Neutron therapy provides no survival benefit for glioma patients and may be associated with additional toxicity.
An institutional phase 2 study was reported where 23 selected patients with glioblastoma were treated on an accelerated proton/photon scheme to cobalt 90-Gy equivalent.15 One cannot comment on whether the median survival of 20 months represents an improvement in survival in such a selected cohort of patients, but it appears that most recurrences occurred in areas adjacent to the full 90-Gy volume and not in the 90-Gy volume, suggesting that the doses in the region of 90 Gy were high enough to control gross tumor.
One area where particle therapy may have been of some advantage is in the treatment of skull base tumors, particularly chordomas and low-grade chondrosarcomas. The management of these two neoplasms with higher doses of radiotherapy is associated with better local control rates and improved survival.16 The challenge in delivering these higher doses lies in the critical structures that are immediately adjacent to or involved by these tumors, namely the brainstem, optic pathways, and cranial nerves and vessels. Improved local control and survival were seen in these tumors in the initial reports utilizing protons in the 1980s and 1990s where higher radiation doses in the 70-Gy range could be safely delivered compared with the photon techniques available during that era. However, in the last 15 years there have been significant advances in photon delivery technology, such that equivalent results are now seen with modern photon techniques in terms of local control and survival with the same low risk of toxicity as achieved with protons.17,18
Though photons are able to achieve the same high-dose volumes as with protons, the advantage of protons is in the intermediate- to low-dose volumes. As a result of this difference, protons and photons would be expected to achieve the same tumor control rates, but the potential advantage with protons over photons may lie in the expectation of a decrease in the long-term effects of radiation related to cognition, hormonal deficiency, cerebral vascular events, and carcinogenicity. Children with primary central nervous system (CNS) neoplasms represent the group of patients with CNS neoplasms who might possibly benefit the most from the use of protons.
Special Consideration
• Proton therapy provides no survival benefit for patients with CNS tumors, but is likely to be associated with significantly reduced long-term effects in children.
Boron-Neutron Capture Therapy
Boron-neutron capture therapy (BNCT) was developed in an effort to confine the damaging effects of particle therapy to tumor cells, sparing normal cells altogether. It involves the administration of a boron-containing chemical that theoretically would be preferentially taken up by tumor cells as opposed to normal cells. Boron has a large neutron capture cross section for slow or thermal neutrons, and these neutrons are present in abundance in nuclear reactors. They have an average energy of 0.025 electron volts (eV), far less than the 10 eV required to strip electrons from atoms and ionize tissue. When boron and these slow thermal neutrons interact, nuclear fission occurs, with the fragments sharing 2.4 MeV of energy in the following fashion:
10B + 1n → (11B) → 7Li + 4He + 2.4 MeV
The lithium and helium fragments are heavy and travel at most 10 μm from the site of the capture reaction, which essentially limits the injury to the individual cell in which the reaction takes place. All other elements in tissue have a very low cross-section for neutron capture.
The first clinical experience was reported in 1954 when 10 patients with malignant brain tumors were treated.19 The first group treated in this fashion in the United States included 17 patients with glioblastoma and one patient with a medulloblastoma; they were treated in 1960 and 1961.20 No patient survived 1 year, and the average survival was 5.7 months. At autopsy, brain swelling, perivascular fibrosis, and cerebral necrosis were evident. The authors found that this was due to boron levels three to four times higher in blood vessels than in tumor, causing all subsequent work to cease at that point.
There has been a revival in studying this technology with the use of fast neutrons and newer boron-containing compounds.21,22 Ongoing studies are being done in patients with glioblastoma, but further work will be required before this complex therapy becomes available outside of an experimental setting.
Radiation Sensitizers
Radiosensitizers are chemicals that increase the lethal effects of radiation. Many chemicals have been found to fit this definition; however, only those that have demonstrated a potential differential effect between tumor and normal tissues would warrant further investigation. The two major classes of compounds investigated to date are hypoxic cell sensitizers and halogenated pyrimidines.
Hypoxic Cell Sensitizers
Intraoperative in vivo measurements and examinations of patients using fluorine-18-fluoromisonidazole positron emission tomography (PET) have demonstrated the presence of hypoxic regions in glioblastomas.23,24 It has been well established in the laboratory that hypoxic cells are significantly more resistant to radiation than are euoxic cells by an order of 2.5 to 3. Hypoxic cell sensitizers would thus sensitize the hypoxic tumor cells without increasing the radiation effect on the already well-oxygenated normal tissues. The first study reported a positive effect of metronidazole in a small randomized study in 1976.25 However, the patient numbers were small, and the median survival of 4 months with radiation alone was considerably less than that seen in most other studies. Since then, there have been 11 additional randomized studies (involving 1,605 patients) that have not shown any benefit from the addition of nitroimidazoles to various combinations of radiotherapy and chemotherapy.1
Pitfall
• Hypoxic cell sensitizers may not be effective in malignant gliomas because sensitizing drugs may not add any additional effect over that achieved with the use of fractionated radiotherapy.
Halogenated Pyrimidines
The halogenated pyrimidines 5-bromodeoxyuridine (BUdR) and 5-iododeoxyuridine (IUdR) are similar to the normal DNA precursor thymidine, having a halogen substituted in place of a methyl group. These compounds are incorporated into DNA in place of thymidine in a competitive fashion, which leads to an increased sensitivity of cells incorporating these compounds to the effects of radiation and ultraviolet light. The rationale for using these compounds in the treatment of brain tumors is that mitotically active tumor cells are much more likely to incorporate these compounds than the slowly replicating glial and vascular cells in the normal brain. An increase in median survival was reported for anaplastic astrocytoma patients from 82 weeks in prior studies to 252 weeks in patients treated with radiation, BUdR, and chemotherapy.26 There was no significant improvement seen with the use of BUdR for patients with glioblastoma. As a result of this observation, the RTOG embarked on a randomized study for patients with anaplastic astrocytoma: 60 Gy in 30 fractions with and without BUdR, both arms followed by a chemotherapy regimen known as PCV, consisting of procarbazine, chloroethylcyclohexylnitrosourea (lomustine; CCNU), and vincristine. The study was closed prematurely when the initial 189 patients were analyzed. The 1-year survival rate for radiotherapy, PCV, and BUdR was 68% versus 82% for radiotherapy plus PCV, clearly a negative result.27
Photodynamic Therapy
5-Aminolevulinic acid (5-ALA) is the precursor for heme synthesis in mammalian cells, which is naturally metabolized to protoporphyrin-IX (Pp) prior to conversion to heme.28 Malignant glial cells lack the ability to convert Pp to heme, and hence it accumulates in glial tumors selectively and not in normal glial or neuronal tissue, and Pp is a photoactive compound that absorbs violet blue light and emits red light, a quality that can be utilized to better appreciate the extent of the glioma at surgery and may help in achieving better resections.
Photodynamic therapy refers to the preoperative administration of a hematoporphyrin derivative generally 24 hours prior to taking the patient to the operating room, resecting as much tumor as safely achievable, and inserting a red light source into the center of the lipid-filled balloon expanded to fill the surgical cavity and expose the remaining glioma cells to this red light. When hematoporphyrin derivatives are exposed to red light, this creates singlet oxygen, which is cytotoxic to cells. As the hematoporphyrin selectively accumulate in malignant glial tissue, this should be a selective therapy that spares normal tissue.
As a result of the majority of patients with glioblastoma recurring locally, PDT has most extensively been investigated in this group of patients. There have been several uncontrolled single-arm phase II studies of the use of PDT in both newly diagnosed and recurrent glioblastomas with reported improvement in progression-free and overall median survival, but these uncontrolled reports must always be interpreted with caution as the result of the likelihood of selection of better prognostic patients for inclusion in the study.29 Only one prospective randomized study of PDT only as an addition to surgery and radiotherapy has been performed in 43 patients in the PDT arm and 34 patients in the control arm.30 There was an improvement in median survival from 8 months (95% confidence interval [CI], 3–10 months) to 11 months (95% CI, 6–14 months) for the PDT arm, but as the 95% CI overlapped, this was not a statistically significant result. There was a randomized trial of 5-ALA–guided resection and PDT in newly diagnosed patients presumed preoperatively to have high-grade gliomas, and there was an improvement in 6-month progression-free survival from 21% to 41% in the study arm, but no reported improvement in overall survival.31
Photodynamic therapy has been reported to be highly efficacious in superficial bladder, basal cell skin, and esophageal cancers, but the lack of efficacy in glial tumors of the brain and other solid tumors elsewhere is felt to be related to the lack of strong enough specificity of light sensitizer accumulation in tumor cells and to the inability to adequately reach all regions of tumor involvement with red light.32
Brachytherapy
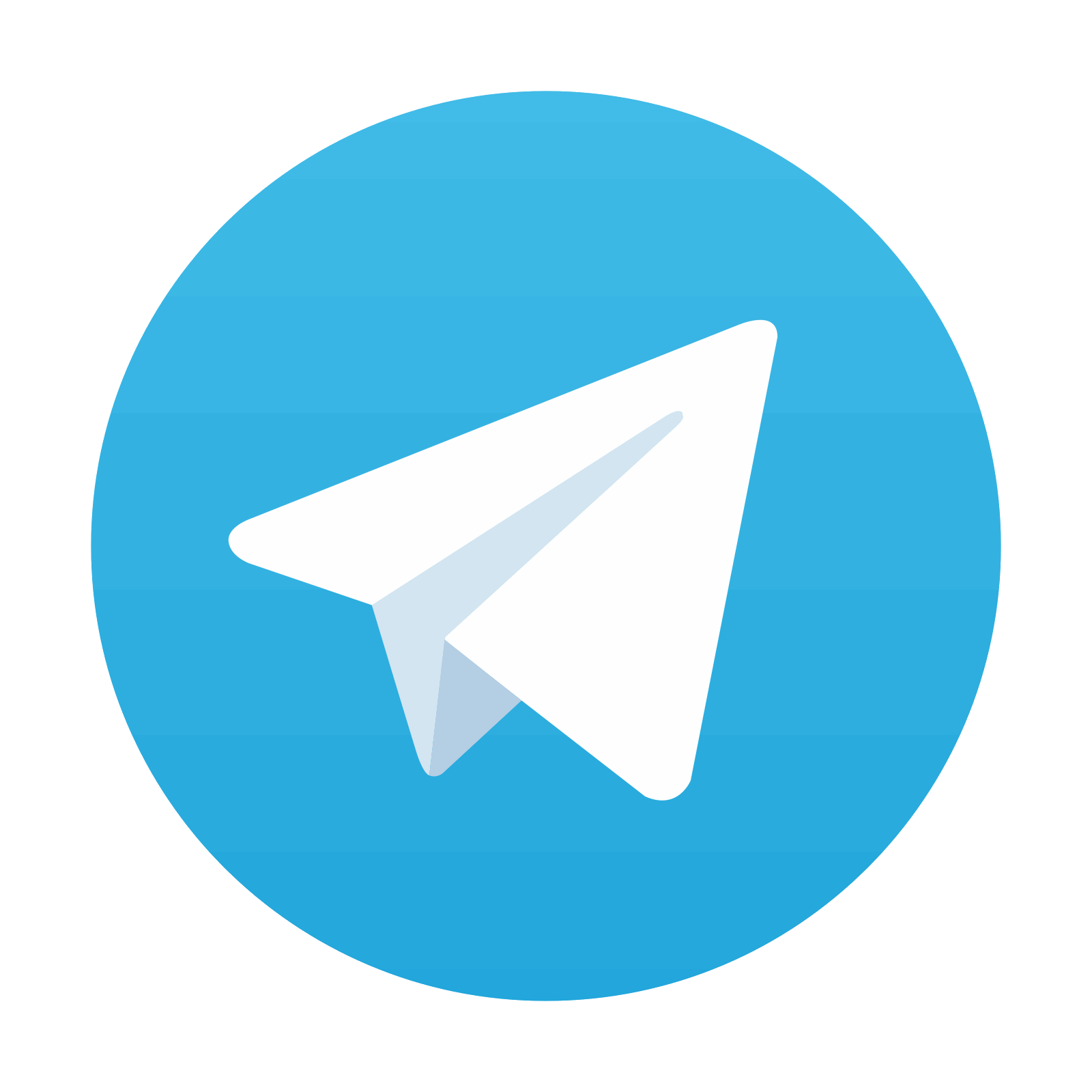
Stay updated, free articles. Join our Telegram channel

Full access? Get Clinical Tree
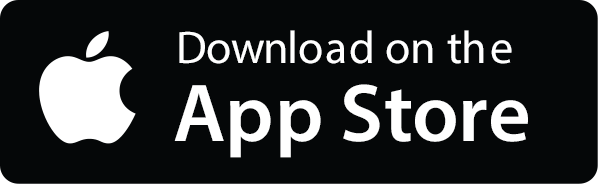
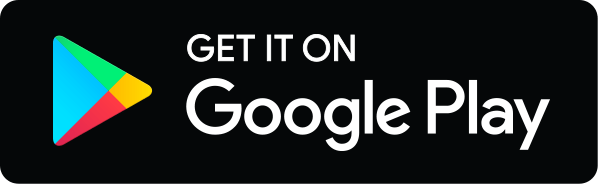