Allergens and Other Factors Important in Atopic Disease
G. Daniel Brooks
Robert K. Bush
An allergen is an antigen that produces a clinical allergic reaction. In atopic diseases, allergens are antigens that elicit an immunoglobulin E (IgE) antibody response. Sensitivity to an allergen can be demonstrated by a wheal-and-flare reaction to that antigen in a skin test, or by in vitro immunoassays such as the radioallergosorbent test (RAST), or enzyme-linked immunosorbent assay (ELISA), which measures antigen-specific IgE in serum. Other methods, usually restricted to research laboratories, also may be used to demonstrate the presence of specific IgE antibodies. These include crossed radioimmunoelectrophoresis (CRIE), immunoblotting technique, and leukocyte histamine release assay. When assessing the contribution of a particular antigen to an observed symptom, the nature of the immune response must be clarified. The clinician must differentiate the allergic (or atopic) response from the irritant response. The immediate Type I IgE-mediated allergic response is distinctly different from the Type 1Va pathophysiologic mechanism mediating the delayed hypersensitivity reactions, which result from contact antigens, such as poison ivy or nickel.
Allergens most commonly associated with atopic disorders are inhalants or foods, reflecting the most common entry sites into the body. Drugs, biologic products, insect venoms, and certain chemicals also may induce an immediate-type reaction. In practice, however, most atopic reactions involve pollens, fungal spores, house dust mites, animal epithelial materials, and other substances that impinge directly on the respiratory mucosa. They cross-link IgE antibodies attached to mast cells or basophils, initiating a series of intracellular process that result in mediator release and allergic symptoms. This chapter is confined to the exploration of these naturally occurring inhalant substances; other kinds of allergens are discussed elsewhere in this text. Aeroallergens are airborne proteins that can cause respiratory or conjunctival allergy. It is common for a single airborne particle, such as a mold spore or a pollen grain, to contain multiple allergens.
Aeroallergens
Certain aeroallergens, such as animal danders, may be localized to individual homes. Others may be associated with occupational exposures, as is the case in bakers who inhale flour. Some sources of airborne allergens are narrowly confined geographically, such as the mayfly and the caddis fly, whose scales and body parts are a cause of respiratory allergy in the eastern Great Lakes area in the late summer. In addition, endemic asthma has been reported in the vicinity of factories where castor beans are processed.
Several methods can be used to determine whether a protein is an allergen. The most clinically related method is an allergen challenge. In a conjunctival or nasal challenge, the extract is introduced directly to the affected mucosa to look for typical allergy symptoms. In a bronchoprovocation challenge, the allergen is inhaled and pulmonary function is deemed significantly different if there is a 20 % decline in FEV1. These methods are generally too impractical to perform in an office setting. Most often a skin test is performed to determine whether an extract can elicit the typical wheal-and-flare response. Finally, tests to estimate allergen-specific IgE can be performed with patient sera. Although most tests are performed with crude extracts, specific IgE tests can be performed on serum to examine individual allergenic proteins within an extract. From a practical standpoint, it is the presence of specific IgE to a protein in the sera of clinically allergic patients that defines it as an allergen.
The chemical nature of certain allergens has been studied intensively, although the precise composition of many other allergens remains undefined. For an increasing number of allergens, the complementary DNA (cDNA) sequence has been derived. For others, the physiochemical characteristics or the amino acid sequence is known. Still other allergens are known only as complex mixtures of proteins and polypeptides with varying amounts of carbohydrate. Details of the chemistry of known allergens are described under their appropriate headings.
The methods of purifying and characterizing allergens include biochemical, immunologic, and biologic techniques. The methods of purification involve techniques such as chromatography, immunoprecipitation, and molecular biology. All of these purification techniques rely on sensitive and specific assay techniques for the allergen as reviewed below.
Allergen Nomenclature
To be recognized as an allergen by the International Union of Immunological Societies (IUIS), a protein must have evidence of allergenicity in at least five individuals and 5% of the population studied (1). Allergens to a specific source such as ragweed pollen or cat dander, can be classified as either major or minor allergens. Major allergens are those that result in IgE greater than 50% of the population sensitized to the source. Minor allergens are those that result in specific IgE in less than 50% of those individuals sensitized to the specific source, be it a pollen or dust mite. Sometimes authors refer to allergens that result in specific IgE in about 50% of the sensitized population as intermediate allergens.
The nomenclature for individual allergen proteins has been established by the IUIS: the first three letters of the genus, followed by the first letter of the species and an Arabic numeral. For example, the primary allergen in cat ( Felis domesticus ) is Fel d 1 . Prior to the adoption of this nomenclature system, grass allergens, ragweed allergens, cockroach allergens, and dust mite allergens all had separate naming systems, that are now only of historical interest. The numbering given to the allergen is often adjusted to account for proteins in separate species that are either cross-reactive or structurally similar. The nomenclature of the German cockroach (Blatella germanica ) and the American cockroach (Periplaneta americana) allergens illustrates this principle well (Table 6.1). Notice that Bla g 6 and Per a 6 are both members of the
Troponin C family of molecules. Bla g 1 and Per a 1 have similar attributes, though they do not have an identified function. There has not yet been an allergen identified in the American cockroach that is similar to Bla g 2, so there is no Per a 2. As allergenic proteins are matched by number, they often must be assigned new names, which can lead to confusion when reading even relatively recent journal articles. An updated list of all established allergens with reference to obsolete names is maintained by the IUIS (1).
Troponin C family of molecules. Bla g 1 and Per a 1 have similar attributes, though they do not have an identified function. There has not yet been an allergen identified in the American cockroach that is similar to Bla g 2, so there is no Per a 2. As allergenic proteins are matched by number, they often must be assigned new names, which can lead to confusion when reading even relatively recent journal articles. An updated list of all established allergens with reference to obsolete names is maintained by the IUIS (1).
Table 6.1 Cockroach Allergens | ||||||||||||||||||||||||||||||||
---|---|---|---|---|---|---|---|---|---|---|---|---|---|---|---|---|---|---|---|---|---|---|---|---|---|---|---|---|---|---|---|---|
|
Isoallergens are proteins within a species that have similar immunologic properties and/or molecular structures, but differ in some way such as isoelectric point, carbohydrate content, or amino acid composition. For example, the ragweed allergen Amb a 1 has four isoallergenic variants based on biochemical studies and cDNA analyses (1). The Amb a 1 isoallergen sequences all have the same first 25 proteins, but vary in the rest of their structure.
Sampling Methods for Airborne Allergens
Patients commonly seek out daily reports of pollen or mold spore levels from the newspaper, television, or Internet. They often use these levels to correlate and predict their allergy symptoms. It is important to understand that all of the current methods for reporting these levels involve averaging pollen levels from the day before. Thus the levels may be helpful in correlating previous symptoms but are of limited use in correlating current symptoms or predicting future symptoms. There are commercial companies that claim to have computer models that predict pollen counts. However, there are no publications that have prospectively determined the value of computer models in predicting pollen counts (2,3).
Aerobiological sampling attempts to identify and quantify the allergenic particles in the ambient atmosphere, both outdoors and indoors. Commonly, an adhesive substance is applied to a microscope slide or other transparent surface, and the pollens and spores that stick to the surface are microscopically enumerated. Devices of varying complexity have been used to reduce the most common sampling errors relating to particle size, wind velocity, and rain. Fungi also may be sampled by culture techniques. Although many laboratories use various immunoassays to identify and quantify airborne allergens, the microscopic examination of captured particles remains the method of choice. Two types of sampling devices are most commonly used: impaction and suction. Gravitational samplers were used historically, but are rarely used today because they provide qualitative data not quantitative.
Impaction Samplers
Impaction samplers are the most common outdoor allergen samplers. Rotating arm impaction samplers have two vertical, adhesive-coated collecting arms mounted on a crossbar, which is rotated by a vertical motor shaft. Small particles, particularly pollen grains, are prone to blowing in the wind in a way that interferes with gravitational settling. They become more likely to impact on an adhesive surface. The sampler rotates up to several thousand revolutions per minute to overcome the effects of wind. However, at this speed turbulence may “push” the pollen away and decrease sampling. For this reason the sampling surface is small (1 to a few millimeters) to get the highest rate of impaction. Small surface areas, however, are rapidly overloaded, causing a decrease in the efficiency of capture. These samplers usually are run intermittently (20 to 60 seconds every 10 minutes) to reduce overloading. In some models, the impacting arms are retracted or otherwise protected while not in use. The Rotorod sampler (Fig. 6.1) is a commercially available impaction sampler and has been shown to be over 90% efficient at capturing pollen particles of approximately 20 μm diameter. It is much less efficient at capturing smaller particles, especially those <5 μm diameter.
Suction Samplers
Suction samplers employ a vacuum pump to draw the air sample into the device. Although suitable for pollens, they are more commonly used to measure smaller particles such as mold spores. Disorientation with wind direction and velocity skews the sampling efficiencies of particles of different sizes. For example, if the wind
velocity is less than that generated by the sampler, smaller particles are collected in greater concentrations than exist in the ambient air. The reverse is true for greater wind velocities. The Hirst spore trap is an inertial suction sampler with a clock mechanism that moves a coated slide at a set rate along an intake orifice. This enables discrimination of diurnal variations. A wind vane orients the device to the direction of the wind. The Burkard spore trap collects particles on an adhesive-coated drum that takes 1 week to make a full revolution around an intake orifice. Both of these spore traps are designed to measure nonviable material. Spore traps are the most flexible devices for sampling particles over a wide range of sizes.
velocity is less than that generated by the sampler, smaller particles are collected in greater concentrations than exist in the ambient air. The reverse is true for greater wind velocities. The Hirst spore trap is an inertial suction sampler with a clock mechanism that moves a coated slide at a set rate along an intake orifice. This enables discrimination of diurnal variations. A wind vane orients the device to the direction of the wind. The Burkard spore trap collects particles on an adhesive-coated drum that takes 1 week to make a full revolution around an intake orifice. Both of these spore traps are designed to measure nonviable material. Spore traps are the most flexible devices for sampling particles over a wide range of sizes.
The Anderson sampler is another suction device, but it is unique in its adaptability for enumerating viable fungal spores. Air passes through a series of sieve-like plates (either two or six), each containing 400 holes. Although the air moves from plate to plate, the diameter of the holes decreases. The larger particles are retained by the upper plates and the smaller ones by successive lower plates. A Petri dish containing growth medium is placed beneath each sieve plate, and the spores that pass through the holes fall onto the agar and form colonies. This method has value for identifying fungi whose spore morphologic features do not permit microscopic identification. In general, however, nonviable volumetric collection techniques more accurately reflect the actual spore prevalence than do volumetric culture methods. The volume of air sampled is easy to calculate for suction devices because the vacuum pumps may be calibrated. In the case of rotation impaction samplers, there are formulas that depend on the surface area of the exposed bar of slide, the rate of revolution, and the exposure time. After the adherent particles are stained and counted, their numbers can be expressed as particles per cubic meter of air. Gravitational samplers cannot be quantified volumetrically.
The location of samplers is important. Ground level is usually unsatisfactory because of liability, tampering, and similar considerations. Rooftops are used most frequently. The apparatus should be placed at least 6 m (20 ft) away from obstructions and 90 cm (3 ft) higher than the parapet on the roof.
Fungal Culture
Fungi also may be studied by culture techniques. This is often necessary because many spores are not morphologically distinct enough for microscopic identification. In such cases, characteristics of the fungal colonies are required. Most commonly, Petri dishes with appropriate nutrient agar are exposed to the air at a sampling station for 5 to 30 minutes. The plates are incubated at room temperature for about 5 days, and then inspected grossly and microscopically for the numbers and types of colonies present. Cotton-blue is a satisfactory stain for fungal morphologic identification. Potato-dextrose agar supports growth of most allergenic fungi, and rose bengal may be added to retard bacterial growth and limit the spread of fungal colonies. Specialized media such as Czapek agar may be used to look for particular organisms (e.g., Aspergillus or Penicillium).
The chief disadvantage of the culture plate method is a gross underestimation of the spore count. This may be offset by using a suction device such as the Anderson or Burkard sampler. A microconidium containing many spores still grows only one colony. There may be mutual inhibition or massive overgrowth of a single colony such as Rhizopus nigricans. Other disadvantages are short sampling times, as well as the fact that some fungi (rusts and smuts) do not grow on ordinary nutrient media. Furthermore, avoiding massive spore contamination of the laboratory is difficult without precautions such as an isolation chamber and ventilation hood.
Immunologic Methods
Numerous immunologic methods of identifying and quantifying airborne allergens have been developed. In general, these methods require more sophisticated instruments and thus are unlikely to replace the physical pollen count. The immunologic assays do not depend on the morphologic features of the material sampled, but on the ability of eluates of this material collected on filters to interact in immunoassays with human IgE or IgG or with mouse monoclonal antibodies (4,5). Studies at the Mayo Clinic have used a high-volume air sampler that retains 95% of particles larger than 0.3 μm on a fiberglass filter. The antigens, of unknown composition, are eluted from the filter sheet by descending chromatography. The eluate is dialyzed, lyophilized, and reconstituted as needed. This material is analyzed by RAST inhibition for specific allergenic activity or, in the case of antigens that may be involved in hypersensitivity pneumonitis, by interaction with IgG antibodies. The method is extremely sensitive. An eluate equivalent to 0.1 mg of pollen produced 40% to 50% inhibition in the short ragweed RAST. An equivalent amount of 24 μg of short ragweed pollen produced over 40% inhibition in the Amb a 1 RAST (6). The allergens identified using this method have correlated with morphologic studies of pollen and fungal spores using traditional methods and with patient symptom scores. The eluates also have produced positive results on prick skin tests in sensitive human subjects (7). These techniques demonstrate that with short ragweed, different-sized particles from ragweed plant debris can act as a source of allergen in the air before and after the ragweed pollen season. Unexpectedly, appreciable ragweed allergenic activity has been associated with particles less than 1 μm in diameter (8).
Use of low-volume air samples that do not disturb the air and development of a sensitive two-site
monoclonal antibody immunoassay for the major cat allergen (Fel d 1) have made accurate measurements of airborne cat allergen possible (5). These studies confirm that a high proportion of Fel d 1 is carried on particles smaller than 2.5 μm. During house cleaning, the amount of the small allergen-containing particles in the air approached that produced by a nebulizer for bronchial provocation (40 ng/m3). The results indicate that significant airborne Fel d 1 is associated with small particles that remain airborne for long periods. This is in contrast to prior studies with house dust mites (9) in which the major house dust mite allergen Der p 1 was collected on large particles with diameters greater than 10 μm. Little of this allergen remained airborne when the room was undisturbed.
monoclonal antibody immunoassay for the major cat allergen (Fel d 1) have made accurate measurements of airborne cat allergen possible (5). These studies confirm that a high proportion of Fel d 1 is carried on particles smaller than 2.5 μm. During house cleaning, the amount of the small allergen-containing particles in the air approached that produced by a nebulizer for bronchial provocation (40 ng/m3). The results indicate that significant airborne Fel d 1 is associated with small particles that remain airborne for long periods. This is in contrast to prior studies with house dust mites (9) in which the major house dust mite allergen Der p 1 was collected on large particles with diameters greater than 10 μm. Little of this allergen remained airborne when the room was undisturbed.
Many pollen grains may be difficult to distinguish morphologically by normal light microscopic study. Immunochemical methods may permit such distinctions. Grass pollen grains collected from a Burkard trap were blotted onto nitrocellulose; then, by using specific antisera to Bermuda grass, a second antibody with a fluorescent label, and a fluorescent microscope study, Bermuda grass pollen grains could be distinguished from grass pollens of other species (10). These newer methods show promise because they measure allergenic materials that react with human IgE. Currently, immunochemical assays to quantify the major house dust mite allergens Der p 1 and Der f 1 and the major cat allergen Fel d 1 in settled dust samples are commercially available. Further studies with these techniques may lead to a better understanding of exposure–symptom relationships.
Standardization of Allergenic Extracts
The need to standardize allergenic extracts has been recognized for many years. Variability in antigen composition and concentration is a major problem in both allergy testing and allergen immunotherapy. Without standardization of extracts, there is no accurate system of quality control. The clinician often is forced to alter immunotherapy schedules with each new vial of extract because of lot-to-lot variability. Each allergen extract supplier uses its own assays and rarely compares specific antigen concentrations with competitors. The result of this disparity is that the clinician must bring more art than science to the field of allergen immunotherapy. Fortunately, this is changing, with the requirement for standardization of ragweed pollen, house dust mite, cat dander, and grass pollen extracts. The development of purified and even cloned allergens that can be expressed in bacteria or yeast hosts have allowed the production of vast quantities of allergen extract with little or no variance between batches (11). With investigators, clinicians, and government agencies that license extracts demanding improved standardization, it is expected that more progress in this area of allergy will be made in the near future.
Quantification of Allergens
The traditional method of preparing and labeling allergens for clinical use is to extract a known weight of defatted pollen or other allergenic particle in a specified volume of fluid. For example, 1 g in 100 mL of fluid would yield a 1% (1:100) solution. This weight per volume (w/v) system still is one of the most commonly used in clinical practice. This solution can be concentrated or diluted as needed.
Another system of measurement, used by some extract manufacturers, is the protein-nitrogen unit (PNU). The basis of the PNU system is the fact that most allergenic moieties of pollens are proteins, and that the ratio of protein to dry weight of pollen varies from plant to plant. In this method, nitrogen is precipitated by phosphotungstic acid and measured by the micro-Kjeldahl technique. One PNU is 0.0l μg of protein nitrogen.
Both of these methods are used for nonstandardized inhalant and food allergens; clinicians generally must communicate in terms of these measurements. Unfortunately, neither the w/p nor the PNU truly measures allergenic activity, because not all measured proteins and extractable components in the solution are allergenic. In addition, many complex allergens are destroyed during the harsh extraction procedure. Such problems have been circumvented through the use of biologic assays of “functional” allergen reactivity. Currently, ragweed pollen, grass pollen, house dust mite, and cat allergen extracts are standardized, and their activity is expressed in allergen units or biologic allergenic units. Other allergen extracts may be added to this list in the future. It is essential for anyone devising immunotherapy regimens to have an appreciation for the biologic assays of allergenicity, which are described later.
Characterization of Allergens
While any methods are available to characterize an allergen, many of these, such as the determination of protein content, molecular weight, and isoelectric point, are not unique to the study of allergenic compounds. These are simply methods of describing any protein. Several categories of tests, however, are restricted to studying molecules responsible for IgE-mediated symptoms.
Radioallergosorbent Test
The RAST is described elsewhere in this text. Although primarily used in the quantification of antigen-specific IgE, the test may be adapted to determine antigen concentrations. To measure potency, the unknown allergen is immobilized onto solid-phase supports (cellulose
disks or beads) and reacted with a known quantity of antigen-specific IgE in a standard test system. For comparison, the extracts are compared with a reference standard, which should be carefully chosen. The quantity of extract required to obtain a specified degree of reactivity is determined. The greater the binding of IgE to the antigen, the greater the allergenicity.
disks or beads) and reacted with a known quantity of antigen-specific IgE in a standard test system. For comparison, the extracts are compared with a reference standard, which should be carefully chosen. The quantity of extract required to obtain a specified degree of reactivity is determined. The greater the binding of IgE to the antigen, the greater the allergenicity.
RAST Inhibition Assay
The most widely used assay for in vitro potency of allergenic extract is the RAST inhibition method. This test is a variation of the direct RAST. Serum from an allergic individual (containing IgE) is first mixed with the soluble unknown allergen. Next, a standard amount of the solid-phase (immobilized) allergen is added. The more potent the fluid phase allergen, the less IgE is free to bind to the solid-phase allergen. The technique and its statistical analysis have been standardized. RAST inhibition usually is the key technique to assess total allergenic activity of an extract and is used by manufacturers to calibrate new batches by comparison with the in-house reference preparation. Some have raised concern regarding the continued use of RAST inhibition as a standard technique. The arguments concern the fact that the choice of antigen for the solid-phase reaction is variable and may influence results. In addition, the finite supply of allergenic reference sera limits reproducibility: without identical reference sera and immobilized allergen, comparisons are impossible. Further development of monoclonally derived IgE and recombinant allergens may help with these concerns.
Assessment of Allergenicity
Biochemical methods for analyzing allergens, such as protein composition and concentration, are practical but tell nothing about the allergenicity of the extract. Immunologic reactivity with IgE antibodies as assessed in vitro and in vivo provides this information. Preparations of inhalant allergens contain more than one antigen. Of the several antigens in a mixture, usually one or more dominate in both frequency and intensity of skin reactions in sensitive persons. It is inferred from this that these antigens are the most important clinically. Not all persons allergic to a certain pollen allergen react to the same antigens from that pollen allergen extract, however. The antigens of tree, grass, and weed pollens are immunologically distinct, and this agrees with the clinical and skin test data. As more allergens are isolated and purified, correlations between immunogenicity and biochemical structure have emerged.
There are two methods of determining the allergenicity of an individual protein. One method is to look at how many people make an IgE response against a certain allergen. A major allergen has been defined as one that binds IgE in 50% or more of sensitized patients (12). A second method of determining the allergenicity of a protein is to determine how much of the IgE is bound to that protein. For example, in experiments involving the major cat allergen, Fel d 1, it has been reported that 90% of the IgE antibodies against cat are reacting against Fel d 1 (1).
Theories of Allergenicity
What characteristics determine whether a particle or a protein can become an important allergen? For a particle to be clinically significant as an aeroallergen, it must be buoyant, present in significant numbers, and allergenic. In general, the insect-pollinated plants do not produce appreciable amounts of airborne pollen, as opposed to wind-pollinated plants, which, by necessity, produce particles that travel for miles. However, being present in high concentrations is not enough. For example, pine pollen is abundant in certain regions and is buoyant, but because it does not readily elicit IgE antibodies, it is not a significant aeroallergen. The characteristics of allergenic particles have been examined and theories relating to their allergenicity are described below.
Structural Properties of Aeroallergens
Some protein structures do seem to be more likely to be associated with allergenicity. One factor that may be important is the simultaneous exposure of multiple allergenic epitopes on a single structure to promote cross-linking of IgE. The major birch allergen, Bet v 1, has been compared to a naturally occurring, nonallergenic protein with significant sequence homology using three-dimensional computer modeling. The nonallergenic protein appears to have fewer epitopes on the exposed surface of the molecule and is more likely to be a monomer than Bet v 1 (13). Computer models that predict allergenicity suggest that the presence of multiple allergenic motifs on a protein makes it more likely to be allergenic (14). There is also evidence from computer modeling of known allergen sequences that a number of diverse allergens have a common structural motif, a groove inside an alpha-beta motif, which is also found in some toxins and defensins (15).
Do specific carbohydrate determinants promote allergenicity? Serum from allergic patients often has IgE that interacts with cross-reacting carbohydrate determinants. Many of these cross-reacting carbohydrate determinants are present in a wide variety of proteins and across very different species. There may be some in vivo effects of these epitopes on an immunologic level. However, cross-reacting carbohydrate epitopes often appear to be a source of positive serologic results without clinical significance (16). Carbohydrates have recently been identified in IgE-mediated reactions, and further investigations are underway.
It has been hypothesized that proteins might be more allergenic because of their structural similarity to invasive organisms. Helminths are classically associated with high IgE levels and intuitively might be associated with allergy, but studies in animal models and human populations suggest that helminthic infection is generally protective against the development of allergies (17). An exception, the fish parasite Anisakis, is associated with allergenic symptoms during infection and has been reported as an occupational respiratory allergen in fish-processing factories (18).
Chemical Properties of Allergens and Immune Interactions
It has been known for some time that the major house dust mite allergen, Der p 1 , has structural similarity to cysteine protease enzymes (19). The enzymatic activity of this allergen may have a role in the development of atopic sensitization and asthma. A series of experiments have been done in which mice are sensitized using enzymatically active or inactive Der p 1. In the presence of the active enzyme, the mice create more total IgE and Der p 1-specific IgE (20). Active Der p 1 also has been used as an adjuvant to increase production of ovalbumin-specific IgE (21). A third experiment involved nasal sensitization with either active or inactive Der p 1 . The active enzyme group had increased cellular inflammatory cells in the lungs and increased total IgE levels (22). Thus, the enzymatic activity appears to contribute to the allergenicity of the molecule.
Several mechanisms have been proposed to explain the relation between enzymatic function and the development of sensitization. Enzymatically active Der p 1 can disrupt tight junction between respiratory epithelia in cell cultures (23). This has been proposed as a mechanism by which the allergen can be delivered through the epithelium to the immune cells. Other dust mite allergens, Der p 3, 6, and 9, have been categorized as serine proteases. These allergens can also disrupt the tight junctions in respiratory epithelium (24).
Several immunologic mechanisms have also been proposed to explain the apparent allergenic effects of dust mite enzymes. The enzymatic activity of Der p 1 has been shown to cleave the low-affinity IgE FcR CD23 from human B cells, which may augment IgE synthesis. (25,26). It has also been shown that dendritic cells incubated with enzymatically active Der p 1 generate less IL-12, which would favor TH2 cell responses (27).
The role of allergenic enzymes in activating protease activated receptors (PARs) has also been investigated. It appears that serine proteases, but not cysteine proteases are involved in the activation of protease activated receptors. The major mold allergen from penicillium, Pen c 13, is also a serine protease. Incubation of a respiratory epithelial cell line with Pen c 13 induces IL-8 release through a pathway that is dependent on activation of both PAR-1 and PAR-2 (28,29). Several other major mold allergens have been shown to increase IL-8 and IL-6 secretion in epithelial cell lines. Crude cockroach extracts also activate PAR-2 and stimulate IL-8 production in respiratory epithelial cell lines (30,31). Cysteine proteases, such as Der p 1, can activate respiratory epithelial cells to secrete IL-8, but the mechanism does not involve PAR-2 (32).
Properties of Pollen Grains
It is important to remember that pollen grains are complex structures designed to deliver plant reproductive material. They have many chemicals and proteins that are presented to the respiratory mucosa at the same time as the most allergenic proteins. The biochemical properties of these pollen grains also contribute to their allergenicity. It has been shown that birch, grass, and ragweed pollens contain both serine proteases and cysteine proteases and that these proteases can also disrupt epithelial tight junctions (33). Type 1 grass pollen allergens appear to migrate into the stratum corneum skin via the hair follicles as soon as 15 minutes. This has been proposed as a mechanism of sensitization in atopic dermatitis (34).
Pollen extracts also appear to have direct effects on the immune system. In cell culture, birch pollen extracts have been found to direct dendritic cells toward a more TH2 type of antigen presentation and to recruit more TH2 cells for antigen presentation (35).
Effects of Particle Size
Aeroallergen particle size is an important element of allergic disease. Airborne pollens are in the range of 20 μm to 60 μm in diameter; mold spores usually vary between 3 μm and 30 μm in diameter or longest dimension; house dust mite particles are 1 μm to 10 μm. Protective mechanisms in the nasal mucosa and upper tracheobronchial passages remove most of the larger particles, so only those 3 μm or smaller are thought to reach the alveoli of the lungs. Hence, the conjunctivae and upper respiratory passages receive the largest dose of airborne allergens. Despite this conventional wisdom, examination of tracheobronchial aspirates and surgical lung specimens has revealed whole pollen grains in the lower respiratory tract (36). These are considerations in the pathogenesis of allergic rhinitis and bronchial asthma as well as the effects of chemical and particulate atmospheric pollutants.
The development of asthma after pollen exposure is enigmatic because pollen grains are thought to be deposited in the upper airways as a result of their large particle size. Experimental evidence suggests that rhinitis, but not asthma, is caused by inhalation of whole pollen in amounts encountered naturally (37). Asthma caused by bronchoprovocation with solutions of pollen extracts
is easily achieved in the laboratory, however. Pollen asthma may be caused by the inhalation of pollen debris that is small enough to access the bronchial tree.
is easily achieved in the laboratory, however. Pollen asthma may be caused by the inhalation of pollen debris that is small enough to access the bronchial tree.
Ragweed asthma supports this hypothesis. The major ragweed allergen, Amb a 1, has been found in ambient air, even in the absence of whole pollen (7). Extracts of materials collected on an 8-μm filter that excludes ragweed pollen grains still appear to contain ragweed allergen based on skin testing and ragweed-IgG inhibition (38).
In Melbourne and London, severe outbreaks of asthma have been reported during some thunderstorms. This phenomenon has been referred to as thunderstorm asthma. People who had asthma exacerbations during a thunderstorm were more likely to be sensitive to grass pollen (39). Grass pollen is generally considered to be too large to access the smaller airways of the lungs. However, exposure of grass pollen grains to water creates rupture into smaller, respirable-size starch granules with intact allergens (40). These starch granules have been found to increase 50-fold during a rainstorm and thunderstorm asthma patients are more likely to be sensitive to the starch granules than other asthma patients (39,41). There is evidence for a similar effect of Alternaria spores. Thunderstorm asthma patients were more likely to be sensitive to Alternaria , and counts of broken Alternaria spores correlate with hospital admissions during a thunderstorm (42).
Pollen Allergens
Pollen grains are living male gametophytes of higher plants (gymnosperms and angiosperms). Each grain has an internal limiting cellulose membrane, the intine, and a two-layered external covering, the exine, composed of a durable substance called sporopollenin. Sporopollenin is primarily a high molecular weight polymer of fatty acids.
Morphologic studies of pollens using the scanning electron microscope disclose an intricate infrastructure. The morphologic structure varies in relation to size, number of furrows, form and location of pores, thickness of the exine, and other features of the cell wall (spines, reticulations, an operculum in grass pollens, and air sacs [bladders] in certain conifers). Ragweed pollen is about 20 μm in diameter, tree pollens vary from 20 μm to 60 μm, and grass pollens, which are all morphologically similar, are usually 30 μm to 40 μm. The identification of pollens important in allergic disease is not difficult and is certainly within the capabilities of the physician with no special expertise in botany (43,44).
Some plants produce prodigious amounts of pollen. A single ragweed plant may expel 1 million pollen grains in a single day. Trees, especially conifers, may release so much pollen that it is visible as a cloud and may be scooped up by the handful after settling. The seasonal onset of pollination of certain plants (e.g., ragweed) is determined by the duration of light received daily. Pollination occurs earlier in the northern latitudes and demonstrates little year-to-year variation in terms of date. In the belt from the central Atlantic to the north-central states, August 15 is a highly predictable date for the onset of ragweed pollination. Most ragweed pollen is released between 6:00 AM and 8:00 AM, and release is enhanced by high temperature and humidity. Extended dry spells in early summer inhibit flower development, reduce ragweed pollen production, and thus result in lower counts in August and September.
Most brightly colored flowering plants are of little clinical importance in inhalant allergy because their pollen generally is carried by insects (entomophilous plants) rather than the wind (anemophilous plants). Entomophilous plants have relatively scant, heavy, and sticky pollen. Roses and goldenrod are examples of plants that often are erroneously thought to cause pollinosis because of the time they bloom. Nevertheless, in isolated cases, the pollens of most entomophilous plants can sensitize and then cause symptoms if exposure is sufficient. Of the pollens of anemophilous plants, ragweed has a long range, having been detected 400 miles out at sea. The range of tree pollens is much shorter. Thus, an individual living in the center of a city is more likely to be affected by weed and grass pollens than by trees. Local weed eradication programs, more often legislated than accomplished, are futile in light of the forgoing information. Air conditioners significantly reduce indoor particle recovery because windows are shut when they operate and they largely exclude outdoor air.
Classification of Allergenic Plants
The botanical considerations and taxonomic scheme given here are not exhaustive (43,44). Individual plants, their common and botanical names, geographic distributions, and relative importance in allergy are considered elsewhere in this book.
Anatomy
Seed-bearing plants produce their reproductive structures in cones or flowers. Gymnosperms (“naked seeds”; class Gymnospermae) are trees and shrubs that bear their seeds in cones. Pines, firs, junipers, spruces, yews, hemlocks, savins, cedars, larches, cypresses, retinisporas, and ginkgoes are gymnosperms. Angiosperms produce seeds enclosed in the female reproductive structures of the flower. Angiosperms may be monocotyledons, whose seeds contain one “seed leaf” (cotyledon), or dicotyledons, with two seed leaves. Leaves of monocotyledons have parallel veins, whereas leaves of dicotyledons have branching veins. Grasses are monocotyledons; most other allergenic plants are dicotyledons.
The flower has four fundamental parts:
Pistils (one or more) are the female portion of the plant and consist of an ovary at the base, a style projecting upward, and a stigma, the sticky portion to which pollen grains adhere.
Stamens, which are the male portions of the plant, are variable in number and consist of anthers borne on filaments. Pollen grains are produced in the anthers.
Petals, the colored parts of the flower, vary from three to many in number.
Sepals, the protective portion of the flower bud, are usually green and three to six in number.
The phylogenetically primitive flower had numerous separate parts, as typified by the magnolia. Fusion of flower parts and reduction of their number is a characteristic of phylogenetic advancement. As a group, dicotyledons are more primitive than monocotyledons.
A “perfect” flower contains both male and female organs; an “imperfect” flower contains only stamens or only pistils. Monoecious (“one house”) plants bear both stamens and pistils; the individual flowers may be perfect or imperfect. Dioecious (“two houses”) plants have imperfect flowers, and all flowers on a particular plant are the same type (male and female). Ragweed is a monoecious plant with perfect flowers; corn is a monoecious plant with imperfect flowers; willows are dioecious plants. Like the flowering plants, gymnosperms may be either monoecious (pines) or dioecious (cypresses and ginkgoes).
Taxonomy
Plants are classified in a hierarchical system. The principal ranks, their endings, and some examples are as follows:
Class (-ae): Angiospermae, Gymnospermae
Subclass (-ae): Monocotyledonae, Dicotyledonae
Order (-ales): Coniferales, Salicales
Suborder (-ineae)
Family (-aceae): Asteraceae, Poaceae
Subfamily (-oideae)
Tribe (-eae)
Genus (no characteristic ending; italicized): Acer
Species (genus name plus “specific epithet”): Acer rubrum
Trees: Gymnosperms
Trees may be gymnosperms or angiosperms. The gymnosperms include two orders, the Coniferales (conifers) and the Ginkgoales. Neither is of particular importance in allergy, but because of the prevalence of conifers and the incidence of their pollens in surveys, some comments are in order.
Conifers grow mainly in temperate climates. They have needle-shaped leaves. The following three families are germane to this discussion.
Pinaceae (Pines, Spruces, Firs, and Hemlocks)
Pines are monoecious evergreens whose leaves are arranged in bundles of two to five and are enclosed at the base by a sheath (all other members of the Pinaceae family bear leaves singly, not in bundles). The pollen grains of pines are 45 μm to 65 μm in diameter and have two bladders (Fig. 6.2). This pollen occasionally has been implicated in allergy. Spruces produce pollen grains morphologically similar to pine pollen but much larger, ranging from 70 μm to 90 μm exclusive of the bladders. Hemlock pollen grains may have bladders, depending on the species. The firs produce even larger pollen grains, ranging from 80 μm to 100 μm, not including the two bladders.
Cupressiaceae (Junipers, Cypresses, Cedars, and Savins)
Most of these trees are dioecious and produce large quantities of round pollen grains 20 μm to 30 μm in diameter with a thick intine (internal membrane). The mountain cedar is an important cause of allergic rhinitis in certain parts of Texas and has proliferated where the ecosystem has been disturbed by overgrazing of the grasslands.
Taxodiaceae (Bald Cypress and Redwood)
The bald cypress may be a minor cause of allergic rhinitis in Florida.
Trees: Angiosperms
Most allergenic trees are in this group. The more important orders and families are listed here with relevant notations. Other trees have been implicated in pollen allergy, but most of the pollinosis in the United States can be attributed to those mentioned here.
Order Salicales, Family Salicaceae (Willows and Poplars)
Willows are mainly insect pollinated and are not generally considered allergenic (Fig. 6.2). Poplars, however, are wind pollinated, and some (e.g., species of Populus) are of considerable allergenic importance. Poplar pollen grains are spherical, 27 μm to 34 μm in diameter, and characterized by a thick intine (Fig. 6.2). The genus Populus includes poplars, aspens, and cottonwoods. Their seeds are borne on buoyant cotton-like tufts that may fill the air in June like a localized snowstorm. Patients often attribute their symptoms to this “cottonwood,” but the true cause usually is grass pollens.
Order Betulales, Family Betulaceae (Birches)
Betula species are widely distributed in North America and produce abundant pollen that is highly allergenic.
The pollen grains are 20 μm to 30 μm and flattened, generally with three pores, although some species have as many as seven (Figs. 6.2 and 6.3). The pistillate catkins may persist into winter, discharging small winged seeds.
The pollen grains are 20 μm to 30 μm and flattened, generally with three pores, although some species have as many as seven (Figs. 6.2 and 6.3). The pistillate catkins may persist into winter, discharging small winged seeds.
Order Fagales, Family Fagaceae (Beeches, Oaks, Chestnuts, and Chinquapins)
Five genera of Fagaceae are found in North America, of which only the beeches (Fagus) and oaks (Quercus) are wind pollinated and of allergenic importance. The pollens of these two genera are morphologically similar but not identical. They are 40 μm in diameter, with an irregular exine (outer covering) and three tapering furrows (Figs. 6.2 and 6.4). Both produce abundant pollen; oaks in particular cause a great deal of tree pollinosis in areas where they are numerous.
Order Urticales, Family Ulmaceae (Elms and Hackberries)
About 20 species of elms are in the Northern Hemisphere, mainly distributed east of the Rocky Mountains. They produce large amounts of allergenic pollen and continue to be a major cause of tree pollinosis despite the almost total elimination of the American elm by Dutch elm disease. Elm pollen is 35 μm to 40 μm in diameter with five pores and a thick, rippled exine (Fig. 6.2). Hackberries are unimportant for this discussion.
Order Juglandales, Family Juglandaceae (Walnuts)
Walnut trees (Juglans) are not important causes of allergy, but their pollen often is found on pollen slides. The pollen grains are 35 μm to 40 μm in diameter, with about 12 pores predominantly localized in one area and a smooth exine (Fig. 6.5).
The Hickories (Carya)
These trees produce large amounts of highly allergenic pollen. Pecan trees in particular are important in the etiology of allergic rhinitis where they grow or are cultivated. The pollen grains are 40 μm to 50 μm in diameter and usually contain three germinal pores.
Order Myricales, Family Myricaceae (Bayberries)
Bayberries produce windborne pollen closely resembling the pollen of the Betulaceae. The wax myrtles are thought to cause pollinosis in some areas.
Order Urticales, Family Moraceae (Mulberries)
Certain members of the genus Morus may be highly allergic. The pollen grains are small for tree pollens, about 20 μm in diameter, and contain two or three germinal pores arranged with no geometric pattern (neither polar nor meridial).
Order Hamamelidales, Family Platanaceae (Sycamores)
These are sometimes called “plane trees.” The grains of their plentiful pollen are oblate (flattened at the poles), about 20 μm in diameter, and without pores. There are three or four furrows on the thin, granular exine (Fig. 6.2). Regionally, sycamores may be of allergenic significance.
Order Rutales, Family Simaroubaceae (Ailanthus)
Only the tree of heaven (Ailanthus altissima) is of allergenic importance regionally. Its pollen grains have a diameter of about 25 μm and are characterized by three germinal furrows and three germinal pores.
Order Malvales, Family Malvaceae (Lindens)
One genus, Tilia (the linden or basswood tree), is of allergenic importance, although it is insect pollinated. The pollen grains are distinct, 28 μm to 36 μm, with germ pores sunk in furrows in a thick, reticulate exine.
Order Sapindales, Family Aceraceae (Maples)
There are more than 100 species of maple, many of which are important in allergy. Maple pollen grains have three furrows but no pores (Fig. 6.2). Box elder, a species of Acer, is particularly important because of its wide distribution, its prevalence, and the amount of pollen it sheds.
Order Oleales, Family Oleaceae (Ashes)
This family contains about 65 species, many of which are prominent among the allergenic trees. Pollen grains have a diameter of 20 μm to 25 μm, are somewhat flattened, and usually have four furrows (Fig. 6.6). The exine is coarsely reticulate.
Grasses (Poaceae)
Grasses are monocotyledons of the family Poaceae (or Gramineae). The flowers usually are perfect (Figs. 6.7 and 6.8). Pollen grains of most allergenic grasses are
20 μm to 25 μm in diameter, with one germinal pore or furrow and a thick intine (Fig. 6.9). Some grasses are self-pollinated and therefore noncontributory to allergies. The others are wind-pollinated, but of the more than 1,000 species in North America, only a few are significant in producing allergic symptoms. Those few, however, are important in terms of the numbers of patients affected and the high degree of morbidity produced. Most of the allergenic grasses are cultivated and therefore are prevalent where people live.
20 μm to 25 μm in diameter, with one germinal pore or furrow and a thick intine (Fig. 6.9). Some grasses are self-pollinated and therefore noncontributory to allergies. The others are wind-pollinated, but of the more than 1,000 species in North America, only a few are significant in producing allergic symptoms. Those few, however, are important in terms of the numbers of patients affected and the high degree of morbidity produced. Most of the allergenic grasses are cultivated and therefore are prevalent where people live.
![]() Figure 6.7 Timothy grass (Phleum pratense). Morphologic features of the flowering head. (Courtesy of Arnold A. Gutman, M.D., Associated Allergists Ltd., Chicago, IL.) |
![]() Figure 6.8 June grass or bluegrass (Poa pratensis). Morphologic features of the flowering head. (Courtesy of Arnold A. Gutman, M.D., Associated Allergists Ltd., Chicago, IL.) |
The grass family contains several subfamilies and tribes of varying importance to allergists. The most important are listed here.
Subfamily Festucoideae, Tribe Festuceae
The tribe Festuceae contains meadow fescue (Festuca elatior), Kentucky bluegrass (Poa pratensis), and orchard grass (Dactylis glomerata) (Fig. 6.9), which are among the most important allergenic grasses. The pollens are 30 μm to 40 μm in diameter.
Tribe Argostideae
The Argostideae tribe includes timothy (Phleum pratense) (Fig. 6.8) and redtop (Agrostis alba), two particularly significant grasses in terms of the amount of pollen shed, their allergenicity, and the intensity of symptoms produced. Both are cultivated as forage, and timothy is used to make hay. Other species of Agrostis immunologically similar to redtop are used for golf course greens. Timothy pollens are 30 μm to 35 μm in diameter. Redtop pollens are 25 μm to 30 μm.
Tribe Phalarideae
Sweet vernal grass (Anthoxanthum odoratum) is an important cause of allergic rhinitis in areas where it is indigenous. In the total picture of grass allergy, however, it is not as important as the species previously mentioned. The pollen grains are 38 μm to 45 μm in diameter.
Tribes Triticaceae (Wheat and Wheat Grasses), Aveneae (Oats), and Zizaneae (Wild Rice)
The Triticaceae, Aveneae, and Zizaneae tribes are of only minor or local importance in allergy because they are self-pollinating or produce pollen that is not abundant or readily airborne.
Subfamily Eragrostoideae, Tribe Chlorideae
Bermuda grass (Cynodon dactylon) is abundant in all the southern states. It is cultivated for decorative and forage purposes. It sheds pollen almost year round and is a major cause of pollen allergy. The pollen grains are 35 μm in diameter.
Weeds
A weed is a plant that grows where people do not intend it to grow. Thus, a rose could be considered a weed if it is growing in a wheat field. What are commonly called weeds are small annual plants that grow without cultivation and have no agricultural or ornamental value. All are angiosperms and most are dicotyledons. Those of interest to allergists are wind pollinated, and thus tend to have relatively inconspicuous flowers.
Family Asteraceae (Compositae)
The composite family is perhaps the most important allergenic weed group. Sometimes called the sunflower family, it is characterized by multiple tiny flowers arranged on a common receptacle and usually surrounded by a ring of colorful bracts. There are many tribes within this family; only those of allergenic or general interest are mentioned.
Tribe Heliantheae includes sunflower, dahlia, zinnia, and black-eyed Susan. The flowers cause pollinosis mainly among those who handle them.
Tribe Ambrosieae, or the ragweed tribe, is the most important cause of allergic rhinitis and pollen asthma in North America. Other common weeds in this tribe are the cocklebur and marsh elder. Ambrosia trifida, giant ragweed, may grow to a height of 4.5 m (15 ft) (Fig. 6.10). The leaves are broad with three to five
lobes. The staminate heads are borne on long terminal spikes, and the pistillate heads are borne in clusters at the base of the staminate spikes. The pollen grains, 16 μm to 19 μm in diameter, are slightly smaller than those of Ambrosia artemisiifolia, short ragweed. Short ragweed grows to a height of 120 cm (4 ft) (Fig. 6.11). Its leaves are more slender and usually have two pinnae on each side of a central axis. Pollen grains range from 17.5 μm to 19.2 μm in diameter and are almost indistinguishable from those of giant ragweed (Figs. 6.9, 6.12, and 6.13). There is no practical reason, however, for distinguishing between the two. Ambrosia bidentia,
southern ragweed, is an annual that grows from 30 cm to 90 cm (1 ft to 3 ft) tall. The pollen grains are 20 μm to 21 μm in diameter and resemble those of giant ragweed. Ambrosia psilostachya, western ragweed, grows to a height of 30 μm to 120 μm (1 ft to4 ft). It has the largest pollen grains of all the ragweeds, ranging from 22 μm to 25 μm in diameter. Franseria acanthicarpa, false ragweed, is found mainly in the South and Southwest, where it may cause allergic symptoms. Franseria tenuifolia, slender ragweed, is another allergenic species of this tribe.
lobes. The staminate heads are borne on long terminal spikes, and the pistillate heads are borne in clusters at the base of the staminate spikes. The pollen grains, 16 μm to 19 μm in diameter, are slightly smaller than those of Ambrosia artemisiifolia, short ragweed. Short ragweed grows to a height of 120 cm (4 ft) (Fig. 6.11). Its leaves are more slender and usually have two pinnae on each side of a central axis. Pollen grains range from 17.5 μm to 19.2 μm in diameter and are almost indistinguishable from those of giant ragweed (Figs. 6.9, 6.12, and 6.13). There is no practical reason, however, for distinguishing between the two. Ambrosia bidentia,
southern ragweed, is an annual that grows from 30 cm to 90 cm (1 ft to 3 ft) tall. The pollen grains are 20 μm to 21 μm in diameter and resemble those of giant ragweed. Ambrosia psilostachya, western ragweed, grows to a height of 30 μm to 120 μm (1 ft to4 ft). It has the largest pollen grains of all the ragweeds, ranging from 22 μm to 25 μm in diameter. Franseria acanthicarpa, false ragweed, is found mainly in the South and Southwest, where it may cause allergic symptoms. Franseria tenuifolia, slender ragweed, is another allergenic species of this tribe.
![]() Figure 6.10 Giant ragweed (Ambrosia trifida). Arrangement of staminate heads. (Courtesy of Arnold A. Gutman, M.D., Associated Allergists Ltd., Chicago, IL.) |
![]() Figure 6.12 Scanning electron photomicrograph of ragweed pollen. Notice the pore on the pollen grain (lower right). (Courtesy of D. Lim, M.D., and J.I. Tennenbaum, M.D.) |
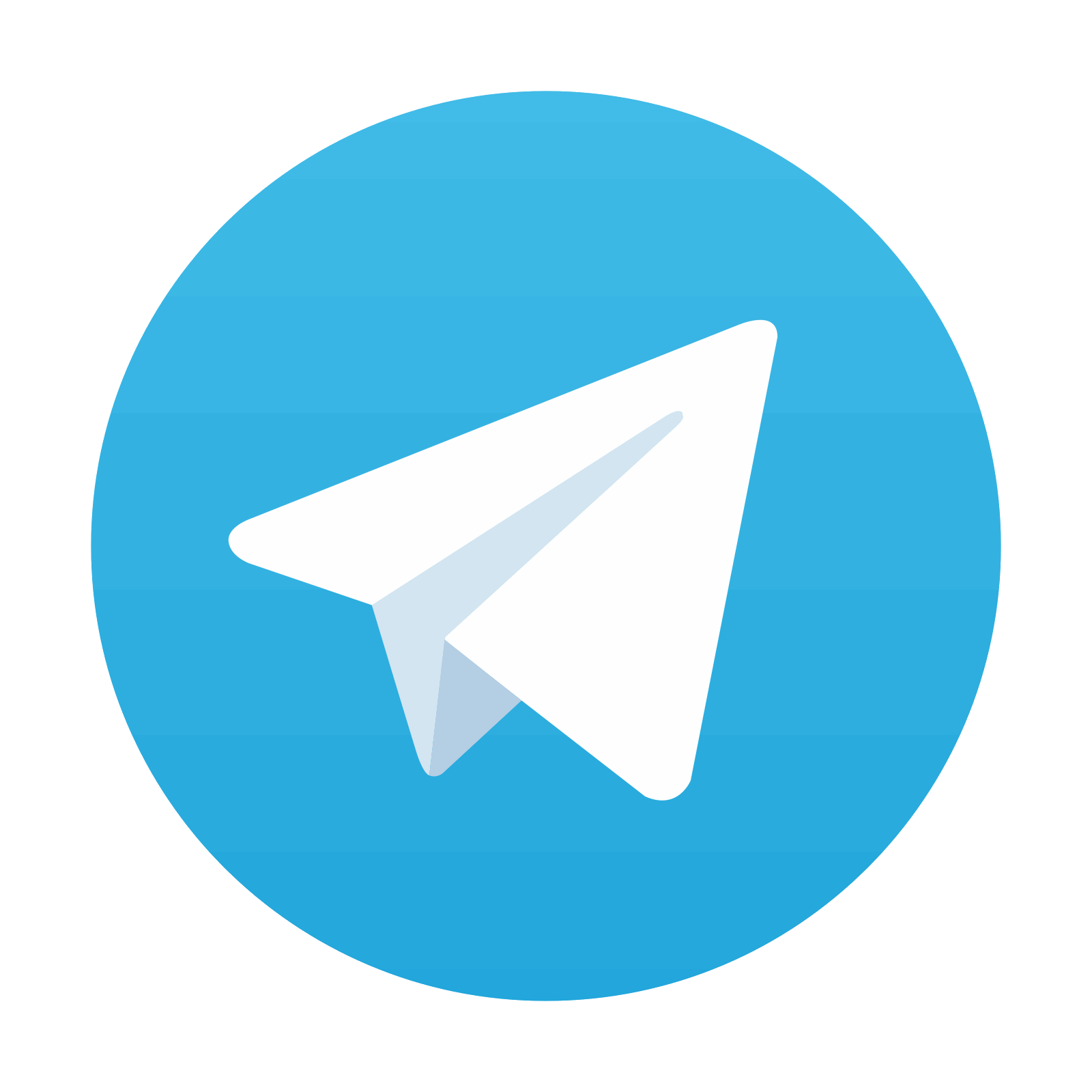
Stay updated, free articles. Join our Telegram channel

Full access? Get Clinical Tree
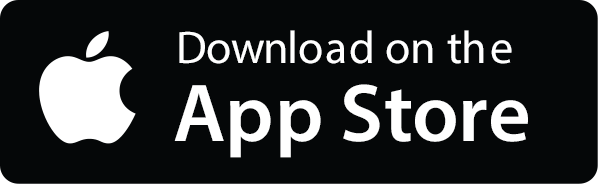
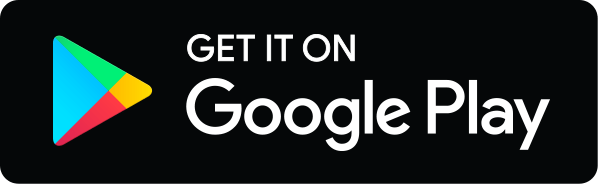
