Chapter 2
A Peek Inside
Your Genes at Work
FEW AREAS OF SCIENCE HAVE CHANGED THE WORLD of medicine more in the past fifty years than genetics, the study of hereditary characteristics and variations passed from parent to child. With capabilities previously unimagined, scientists use genetic processes to trace ancestry, examine fossils, and make foods more disease resistant. Because most diseases, including breast and ovarian cancers, are caused at least in part by changes in the genes, this science is the key to understanding the origins of disease. Blood tests can now detect genetic changes that can cause diseases, from cystic fibrosis in a newborn to breast and ovarian cancer in an adult. Knowing about these genetic disorders can be life changing, because it provides opportunities to reduce the likelihood of developing the disease or to manage it early on. These discoveries are amazing, yet they only scratch the genetic surface. The more scientists understand what causes genetic abnormalities that lead to cancer, the closer we move toward better prevention, detection, treatment, and cure.
The Evolution of Genetic Discovery: From Peas to BRCA
In 1866, Austrian monk Gregor Mendel, considered the father of genetics, made a stunning declaration based on his experiments crossbreeding pea plants: that “factors” (now called genes) determine traits that are passed unchanged to descendants, and for each trait, individuals inherit one gene from each parent. Science ultimately proved Mendel correct and his conclusions became the tenets of modern genetics. Genes pass from parent to child, along with abnormalities within the genes that raise a person’s risk for disease.
In the same year Dr. King announced her discovery, the Human Genome Project was launched to identify the entire set of human genes. By 2003, researchers had successfully labeled all 20,000 or so genes and created a genetic road map showing where each could be found. The massive effort was akin to translating every book ever written into a universal language: the genetic code that enables researchers to study what each gene does and how abnormalities cause disease. Even though it will be several years before we have all the answers, the increasing pace of genetic discoveries is rapidly advancing our knowledge of disease. Because genes appear in exactly the same order in all of us, knowing the location of a particular disease-causing gene is a huge medical leap forward. We now know where to find more than four thousand genes related to diseases, including many cancers.
Discovering BRCA1/2 was an important step in identifying high-risk individuals and finding ways to reduce their breast and ovarian cancer risk. As we learn more about BRCA mutations, our arsenal of risk management tools and cancer treatments grows. We still have much to learn about hereditary cancer. We don’t know why some people with mutations get breast cancer and others develop ovarian cancer, or why some never have either disease. We can’t predict when cancer will develop or how individuals will respond to treatment. And there may be as-yet undiscovered gene mutations that also raise cancer risk. Future genetic discoveries will provide those answers, ultimately leading to methods that will eradicate these diseases.
Your Genetic ABCs … and a D
Genetics is a complex science with its own unique language. Understanding the basics helps to clarify how changes in BRCA genes can lead to increased cancer risk.
A is for All of Us
Geraniums, worms, poodles, and humans—all living organisms have the same fundamental microscopic cells that keep us operating. Humans have trillions of cells, each with the same basic structure, yet programmed for very specific tasks like breathing, converting food into energy, and sending messages to and from the brain. You have an almost unimaginable assortment of cells, and most of them contain a complete set of your genetic material sufficient to create an exact copy of you.
B is for the Basics
Within each of our cells (except red blood cells) is a genetic control center called the nucleus. Every nucleus has twenty-three pairs of chromosomes: one of each pair comes from your mother; the other is from your father. Chromosomes are composed of snippets of genetic material that store and transmit the operating instructions that keep our bodies functioning. Genes also come in pairs (except those in egg and sperm cells), one from each parent. Genes work together to determine specific human characteristics, such as height or hair color, that pass from one generation to the next. When someone says, “It’s in your genes,” they’re referring to a characteristic that’s very much like one of your parent’s. They might mean the curly hair both you and your mother have or the artistic ability you share with your father. Some genetic characteristics, such as eye color or the shape of your hairline, don’t affect your health or well-being. Others may cause traits like color blindness or make you more likely to develop diseases such as diabetes or breast cancer.
C is for Cells
We all begin life as a single cell that grows and divides. Those two cells then divide and become four, and so on and so on, until our bodies are formed. This replicating life cycle is normally carried out in an orderly process controlled by genes: some tell cells when to divide, and others put the brakes on cell growth.
D is for DNA
You’ve probably heard of DNA, which stands for deoxyribonucleic acid, the unique genetic material used to link suspects to crime scenes, prove someone’s paternity, or screen for certain mutations that lead to disease. All humans share about 99.9 percent of our DNA. Yet, except for identical twins, no two people are exactly alike. That’s because our individual one-tenth of a percent variation determines our inherited traits, like eye color and hair texture. Although you and your sister have genes that determine eye color, your variation of those genes may have resulted in your eyes being brown, while your sister’s unique genetic variation gave her hazel eyes.
A genetic portrait of your DNA
Genes perform an incredibly important job. These tightly coiled sections of thin DNA fibers issue instructions for making the thousands of proteins that build and support the body’s elaborate operations—move muscles, digest food, repair cell damage. We hear a lot these days about genes, but proteins—often referred to as the body’s building blocks—are the chemicals of life, and every cellular function depends on them. Genes instruct cells which proteins to make based on the type of cell and its needs. Some, like BRCA genes, tell our bodies how to repair damage from sun exposure, chemicals, and other influences. Others maintain just the right number of cells—enough to keep the body healthy, but not too many to encourage tumor growth. Cells, chromosomes, DNA, genes, proteins—mix these elements together uniquely and the result is: you.
Mutations: Spelling Errors in Your DNA Cookbook
Think of DNA as your body’s cookbook, where your genes are recipes for proteins made by your body. DNA recipes are written in a sort of biological shorthand using various combinations of a four-letter alphabet, each representing a different chemical base: A (adenine), C (cytosine), G (guanine), and T (thymine). By combining different variations of these four “letters” into three-letter “words,” DNA issues instructions for all the proteins our bodies need. The unique order of these letters spells out recipes for making everything from hormones to heart valves. Under the microscope, DNA molecules look like the rungs of a ladder made up of A-T and C-G pairs.
The DNA ladder
Table 3. Genetic spelling errors
If you use a cake recipe that includes a typo, your dessert might not turn out the way it should. The same thing happens when genetic changes called mutations create “spelling errors” that garble a DNA recipe for a specific protein. Different types of spelling errors may occur (see table 3).
Some spelling errors completely change the recipe and make it unreadable. Insertions and deletions cause words to shift, resulting in nonsense. Substitution errors can create sentences made up of real words that have different meanings: adding a “bug” egg instead of a “big” egg ruins the recipe. Sometimes, even though all the right letters are there, they end up in the wrong place, or words become scrambled and create meaningless sentences, creating mutations called rearrangements.
Most mutations aren’t harmful. Some play an important evolutionary role, like changing an animal’s appearance or behavior in ways that better adapt it to its surroundings. In some cases, the end result of mutations is disease. Sickle cell anemia, for instance, occurs when a mutation disrupts the instructions for hemoglobin, a protein made by red blood cells to move oxygen through the bloodstream. Mutations can also develop naturally as we grow older, as the wear and tear of living takes its toll on our recuperative abilities, and our genetic repair mechanisms don’t work as effectively as they used to. Cells often develop mutations when they divide, and other mutations occur as a result of environmental and lifestyle influences, such as chemical exposure, radiation, smoking, and alcohol. The body frequently repairs genetic damage before the mutation is copied to new cells. Damage that cannot be repaired creates a bit of a vicious cycle: when a damaged cell divides, it passes all its DNA code, including any unrepaired mutations, on to the next generation of new cells. That error is then copied every time the new cell duplicates itself. If an error occurs in the egg or sperm, it may pass from parent to child in successive generations.
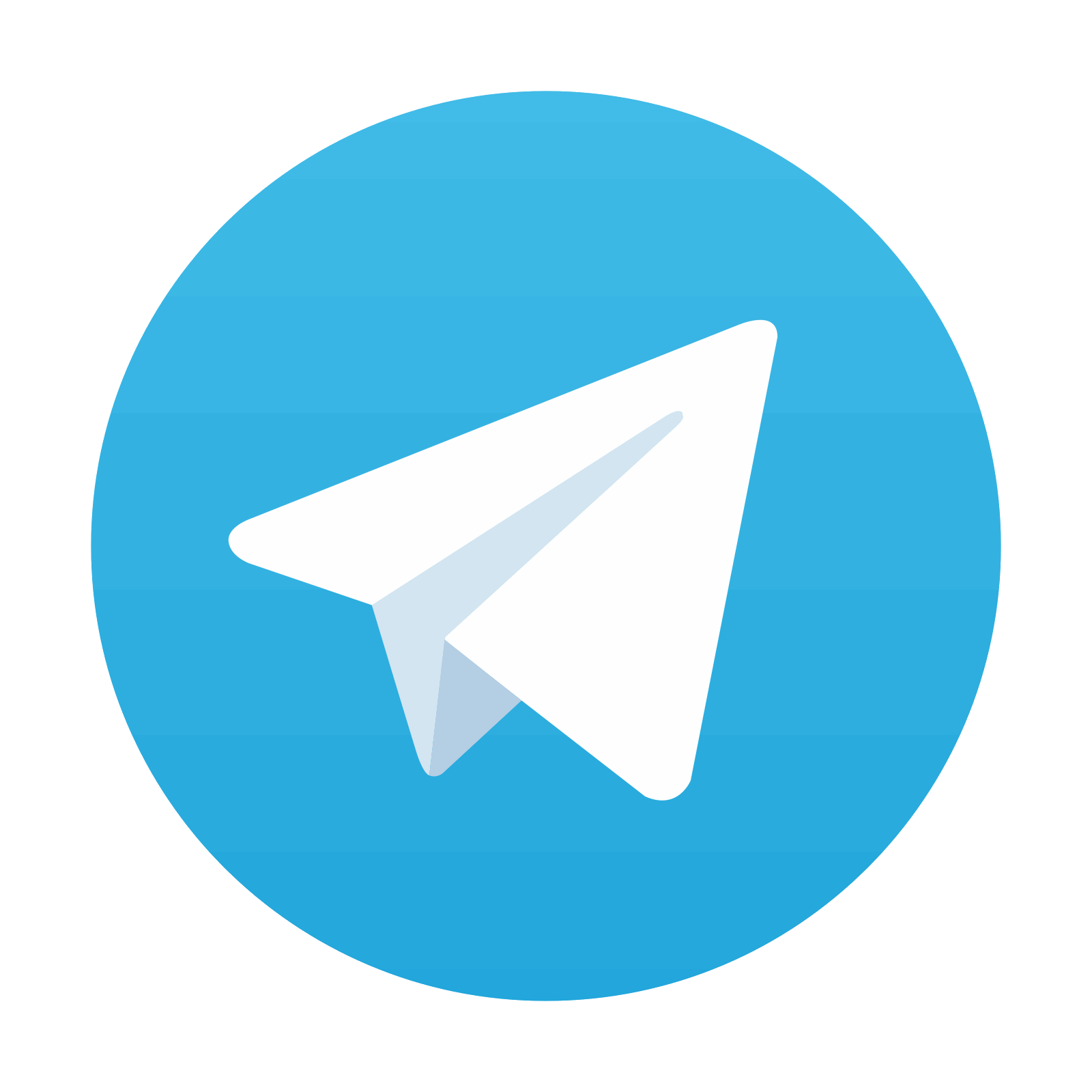
Stay updated, free articles. Join our Telegram channel

Full access? Get Clinical Tree
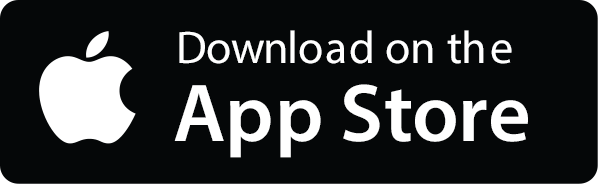
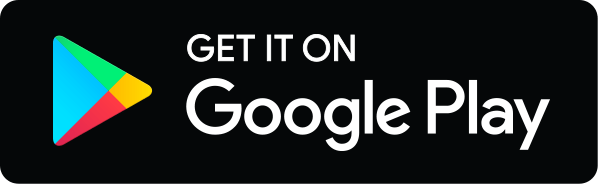