Introduction
This chapter provides an introduction to the ‘principles and practice of geriatric medicine’, the topic of this textbook. Underlying the need for geriatric medicine are the biological changes with time that we call ageing. The purpose of this chapter is to summarize what we know about these changes, how they relate to the diseases and conditions that require medical intervention and why they even occur at all.
This chapter develops several themes. Biological ageing progresses differently in different cells, tissues and organisms. Age theories focusing on one concept are common, for example, free radical, genetic, neuroendocrine, and immune, but they are incomplete in themselves. And finally, there are multiple gaps in our knowledge concerning the causes of ageing.
Ageing Populations
In talking about biological ageing, it is useful to talk about the ageing of whole populations. Although the age at death of an individual varies widely, the survival of whole populations is fairly predictable, and the curves have a similar shape across species. Figure 1.1 shows human survival curves for people in the United States. Each curve represents survival data (percentage surviving) at each age for the calendar years indicated. The survival curves are sigmoidal, and they are characterized by very few deaths in the early years, a short period with a large number of deaths and a tail of old survivors. The age at which the number of survivors starts to decrease rapidly is usually referred to as the onset of senescence. The slope of the curve during the decrease is the rate of senescence, and the age where there are no survivors is the maximal lifespan.
Figure 1.1 Human survival curves. Percentage surviving versus age is plotted for each of the calendar years indicated. Data redrawn from the National Center for Health Statistics (US Bureau of the Census). The dashed line represents a theoretical shift in the data for 2006. The data are shifted such that the age of onset of senescence and maximal lifespan are increased, but there is no change in the rate of senescence.
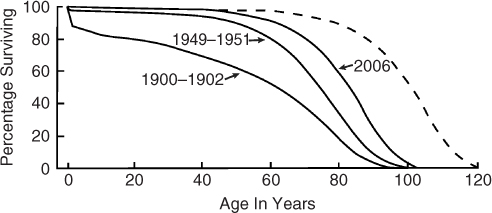
In 1900–1902, there was an early drop in survival, due to infant mortality, followed by a somewhat gradual decline, and ending with a steeper decline between age 70 and 90 years. As the calendar years progress from 1900–1902 to 1949–1951 to 2006, there were many more survivors at each age and a delay in the onset of senescence. This is sometimes referred to as the squaring off of the survival curve. Much of the difference between 1949–1951 and 2006 was due to a delay in the onset of senescence with little change in the rate of senescence (the slope of the decline). Also, the maximum lifespan was similar for all curves. The net effect is that the average lifespan has increased largely through a delay in the onset of senescence with little change in the rate of senescence or in maximal lifespan.
The dotted line represents a theoretical population where the onset of senescence has been further delayed but with an increase in maximal lifespan. This is the type of shift that is seen with caloric restriction of animal models.1 To see such a change in human populations would most likely require a fundamental change in our biological ageing. It would not arise from more effective treatment of the diseases of ageing, which would tend to square off the existing survival curve.2, 3
Although survival curves are useful, they show only one rather dramatic endpoint—death. As more people live longer, there is greater interest in quality of life rather than just the length of life. This has given rise to the concept of ‘functional lifespan’, which can be assessed by various clinical measures. One challenge in the biology of ageing is to develop markers that reflect physiological age rather than just chronological age. Such markers could be used for early intervention with the goal of extending functional lifespan.
Why do We Grow Old?
The question of why we grow old can be answered at many levels—philosophical, religious and biological—and, of course, becomes more relevant personally the older we become. Recent research and thinking form a framework in which to wrestle with the question of why we grow old biologically. This question was proposed as an ‘unsolved problem of biology’ by Medawar in 1952.4 However, recent concepts have led two prominent gerontologists to conclude that ‘aging is no longer an unsolved problem in biology’.2, 5 The reason for this change is that since Medawar first posed the question there has been a large amount of descriptive gerontology—a cataloguing of the physiological and biochemical changes that characterize ageing in many different organisms. As a result, within about the last 20 years biologists have begun to look at the big picture of why we age.
The question of why we grow old is really tied into a fundamental characteristic of life itself—reproduction. Simply put, if the purpose of a particular species is to maintain itself through reproduction, there is no reason to invest biological resources in maintaining a member of that species after the birth and nurturing of the next generation. Hence, after reproduction, species generally decline physiologically and eventually die. This broad perspective is usually referred to as ‘the evolutionary theory of ageing’, and it makes important predictions about the biology of ageing.
The Evolutionary Perspective of Ageing—Key Concepts
The evolutionary perspective of ageing is made up of three interrelated concepts3, 5—mutation accumulation, antagonistic pleotrophy and disposable soma (Table 1.1).
Table 1.1 Evolutionary perspective of ageing.
Mutation accumulation | Genes may accumulate that act late in life and contribute to the physiological decline of an organism |
Antagonistic pleotrophy | Some genes may have positive effects early in life but then have negative effects later in life after reproduction |
Disposable soma | There is a competition for resources between reproductive (germ line) cells and maintenance (somatic) cells, and after reproduction the somatic cells are no longer needed (disposable) |
Mutation Accumulation
The ‘mutation accumulation’ concept was postulated by Medawar in an attempt to answer his own question about why we age.4 It states that genes may accumulate that are expressed only after reproduction. These genes may have deleterious effects that contribute to the physiological decline of the organism. These late-acting genes are not selected against because they do not affect reproduction and nurture of the next generation.
Antagonistic Pleotrophy
An extension of the mutation accumulation concept is the idea that these late-acting genes may exist in the gene pool because they have positive effects early in life. Thus, the same gene may have positive effects early in life but negative effects after reproduction. This concept is called ‘antagonistic pleotrophy’. An example would be the fact that a particular apolipoprotein (ApoE4), which is a significant risk factor for Alzheimer’s disease, maintains itself in the population. Since Alzheimer’s disease arises very late in life after reproduction, there is no selective pressure to remove it from the gene pool. The fact that ApoE4 is still found in the gene pool may mean that it gives some positive advantage early in life.
Disposable Soma
For a species to survive, it must reach reproductive age and nurture the next generation. Since organisms have only a limited amount of biological resources at their disposal, these resources must be allocated carefully between maintenance and reproduction. This is sometimes looked at as a competition between the cells involved in maintenance (the somatic cells) and the cells involved in reproduction (the germ line cells). In such a competition, the most important thing is for the germ line cells to be passed on to the next generation. The somatic cells (the vast majority of the cells in the body) are only a means to that end. In that sense, somatic cells are ‘disposable’ after they have supported the organism through reproduction. Hence this concept has been named the ‘disposable soma’ theory. There is no incentive to maintain the stromal cells past the reproductive age, and a long post-reproductive lifespan could be viewed as wasteful.
Changing Concepts in the Biology of Ageing
The descriptive work and the evolutionary perspective have changed the way in which we look at a number of important concepts in the biology of ageing. In a sense, the fields of geriatrics and gerontology have been around long enough so that there has been an ‘ageing’ of the concepts related to biological ageing (Table 1.2)
Table 1.2 Changing concepts in the biology of ageing.
Older concepts | Newer concepts |
There is a genetic programme that controls the ageing process much like there is a developmental programme | Ageing is not the result of a ‘programme’ but rather the result of ‘biological neglect’ after reproduction |
Biological ageing is a singular process that affects all tissues and organisms equally | Biological ageing has many components that affect tissues and organisms in diverse ways |
There is one ‘theory of ageing’ that explains the ageing process in biological terms | Biological ageing is complex change with time that is described by multiple perspectives (theories) at multiple levels |
Ageing is due either to our environment (extrinsic) or to our genes (intrinsic) | Ageing is roughly 25–35% genetic with the rest influenced by our environment and lifestyle |
Maximal lifespan is characteristic of an organism and is fixed | Maximal lifespan can vary within limits in response to the nutritional and reproductive needs of the organism |
Ageing is the sum of all of the diseases of ageing | Ageing results in an increase in disease, but it is separate from the diseases of old age |
There is No ‘Genetic Programme’ for Ageing
Previously, ageing was viewed as a ‘process’ or even a ‘programme’. Much like a developmental programme, it had its own intrinsic clock that moved inexorably towards universal senescence and death. At various times, this clock has been postulated to be hormonal (hypothalamus–pituitary axis), immune (involution of the thymus) or cellular (cell senescence). However, from an evolutionary perspective, ageing is not a genetic program but rather the result of biological neglect (not necessarily benign) that occurs after reproduction is complete.
Biological Ageing Varies with Tissue and Organism
Since there is no programme, there is no reason to expect that biological ageing will progress the same in all organs of an individual or in all species. The death certificate of a mouse may look very different from that of a human. For example, laboratory rodents often die of kidney disease, but this is a much lesser cause of mortality in humans.
There is No One ‘Theory of Ageing’
Another result of this lack of post-reproductive maintenance is that different organ systems will fail at different rates across tissues and organisms. That is, biological ageing is probably not due to a single universal deficit in all organisms. Put another way, there is no one mechanism or ‘theory of ageing’ that explains everything. However, the classical theories of ageing describe important aspects of biological ageing (see below).
Ageing has Both Genetic and Environmental Components
Some researchers have made a sharp distinction between ageing due to environmental factors and ageing due to genetic factors.2 They classified theories of ageing as either extrinsic (environmental) or intrinsic (genetic). It is becoming clearer that ageing is influenced by both environment and genetic makeup, and it is sometimes difficult to untangle the two. There definitely is a genetic component, as the best predictor of lifespan is the lifespan of one’s parents. Studies of twins suggest that in humans about 25–35% of ageing is genetic and the rest is environmental. Recently, studies of centenarians have found certain genes that seem to be associated with long life. These are sometimes referred to as ‘longevity assurance genes’. The idea is that these genes contribute to long life, but they are not part of a genetic mechanism to regulate lifespan.
Maximal Lifespan can Vary Within Limits
The lifespan of a group of genetically related animals (inbred laboratory rodents under laboratory conditions) is remarkably constant, even though it is not directly regulated. This has led to the concept that lifespan, especially in higher organisms, is fixed. Yet the lifespan of lower organisms (worms, flies, yeast) can be markedly altered by a variety of manipulations. These include restricting diet, boosting antioxidant defences and modulating insulin/growth signalling pathways (see below). However, modulating the lifespan of higher organisms such as mammals seemed difficult, except through caloric restriction.1 Recent studies have suggested that higher organisms have ‘longevity assurance pathways’ that parallel those in lower organisms.6 Modifying these pathways in various ways has made it possible to extend life in mice. Thus, even higher organisms have the ability to modulate their lifespan based on reproductive needs and environmental conditions.
Ageing is not the Sum of the Diseases of Ageing
The relationships between ageing and the diseases that accompany ageing are still the subject of some debate.3 Previously there was a tendency to identify ageing with the diseases of ageing. More recently, the emphasis has been that these diseases may be due to underlying biological changes with age. In other words, increased vulnerability to disease is a characteristic of biological ageing, but ageing is not just the sum of all of the diseases of ageing. The distinction between ageing and the diseases of ageing is very important in terms of research strategies. If ageing is mostly the diseases of ageing, then these diseases will have to be slowly conquered one by one. On the other hand, if there are underlying biological processes driving disease, then understanding and modifying these could possibly reduce the vulnerability to many diseases at once.
The ‘Theories of Ageing’
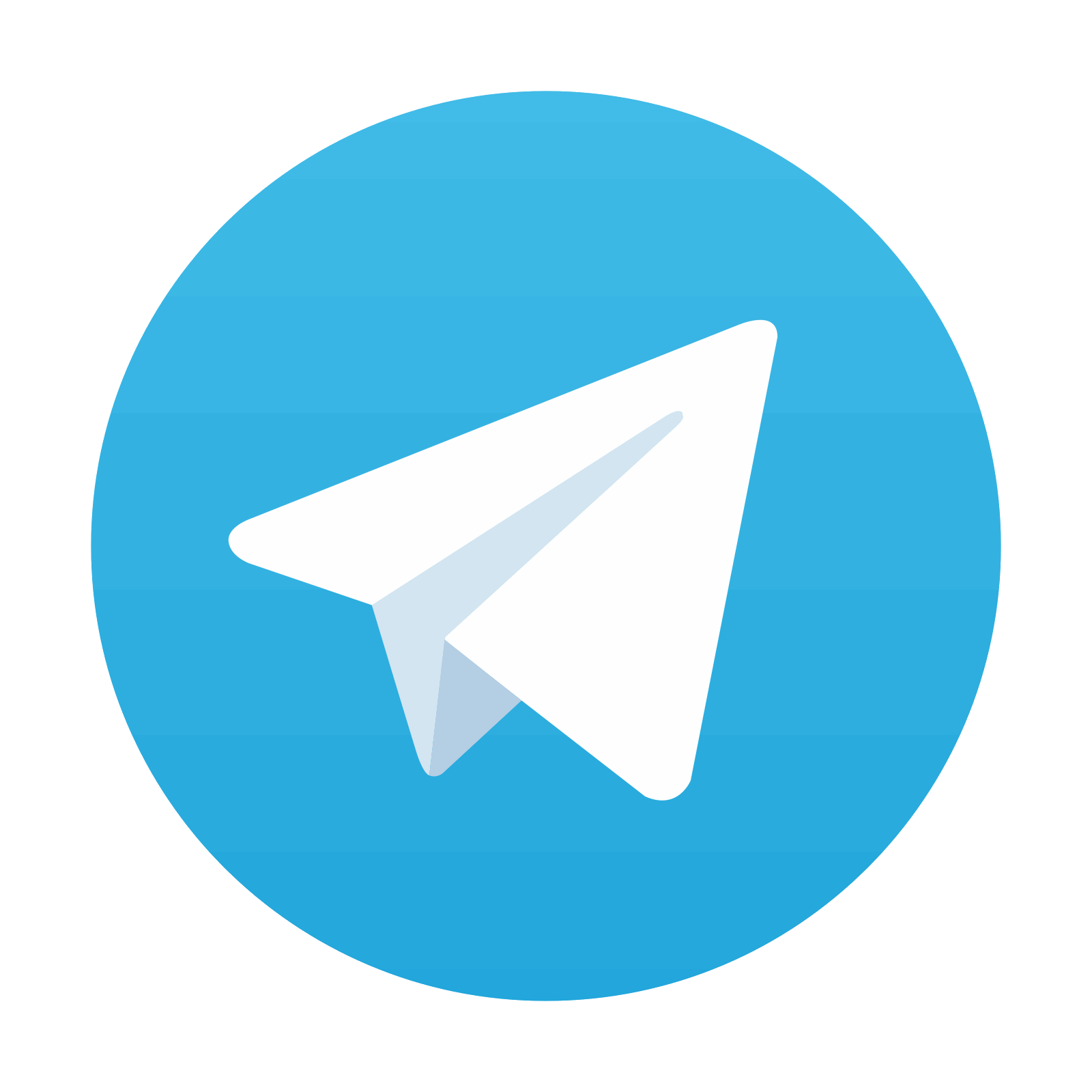
Stay updated, free articles. Join our Telegram channel

Full access? Get Clinical Tree
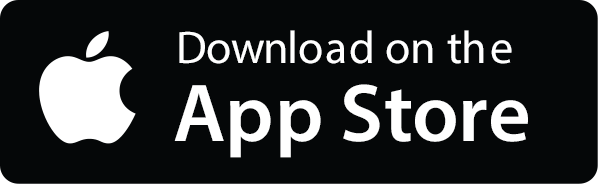
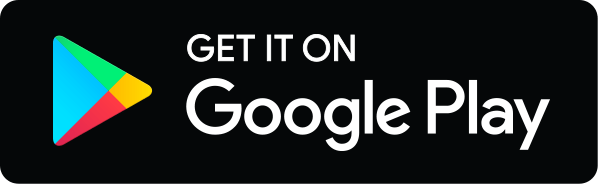