POSTMENOPAUSAL AND AGE-RELATED PRIMARY OSTEOPOROSIS
Part of “CHAPTER 64 – OSTEOPOROSIS“
PATHOPHYSIOLOGY AND ETIOLOGIC CONSIDERATIONS
More than 80% of osteoporosis cases occur among postmenopausal and aging populations. Although some differences in pathogenetic mechanisms have been described,9,10 the overlap between populations seen clinically is sufficient for them to be considered together. Fracture risk at any age is determined primarily by skeletal mass,11,12 which depends on the maximal mass achieved at maturity and on the subsequent rate and duration of bone loss. Various interrelated factors are thought to control the two processes.13 The frequency of falling and factors predisposing to falls are also determinants of fracture risk, particularly for limb fractures.14 Thus, the frail elderly are more likely to sustain fractures than those who maintain their health, coordination, and strength. Reduced bone elasticity and decreased dissipation of force over bone by weaker and less coordinated muscle contractions also add
to fracture risk. The presence of vertebral fractures has been found to be an independent predictor of further vertebral fracture risk,15 and also increases the risk of fractures at other sites, notably the hip.16 The occurrence of any fracture after the age of 40 doubles the risk of further osteoporotic fractures in later life.17
to fracture risk. The presence of vertebral fractures has been found to be an independent predictor of further vertebral fracture risk,15 and also increases the risk of fractures at other sites, notably the hip.16 The occurrence of any fracture after the age of 40 doubles the risk of further osteoporotic fractures in later life.17
DETERMINANTS OF MAXIMUM BONE MASS
Maximum bone mass is achieved some time after puberty (age 18–30) and is primarily determined genetically.18,19 Race, gender, and body size are the principal genetic determinants; consequently, maximum bone mass probably is under polygenic control. The inequality between the sexes is probably not evident prepubertally,20 but is clear after transition through puberty, with total bone mass becoming significantly greater among males.21,22 and 23 The positive correlation between body size and bone mass is well established.23 Bone density is also a function of skeletal size, because the usual two-dimensional techniques employed do not adequately correct for the size of the bone. Gender differences in true density are less than those in bone mass in young adults, and in some studies are found to be insignificant.23 Racial differences in both mass and density are apparent in young adults, with black populations generally having a 5% to 10% greater bone mass.24 In cross-sectional studies these racial differences are maintained with increased age, whereas gender differences become yet greater.
Investigations into the genetic control of bone mass and density have focused on several candidate genes that, by virtue of the known function of their products, have some biologic credibility.19 Thus, investigators have evaluated polymorphisms in the vitamin D receptor; the estrogen receptor (ER-α); the gene for type I collagen, osteocalcin, and the interleukins; and receptors and receptor antagonists. Although all have provided some promising data, each contributes only a small amount to the variability seen in bone density or bone turnover.
Although multiple genetic factors clearly play an important role in determining peak bone mass, other influences are also important. Adequate nutrition, health, and exercise in childhood can modify the likelihood that any individual will reach his or her genetic potential.25,26 Nutritional factors include not only calcium but calories and protein. Furthermore, a normal progression through puberty and the associated growth spurt as well as normal menstrual function are critical to adult skeletal health. Osteoporosis has been dubbed a disease that presents in the geriatric population but often has its roots in the pediatric population.
DETERMINANTS AND PATHOGENESIS OF BONE LOSS
In women, bone loss begins between 20 to 30 years of age, at least in the hip. Bone mass may be more stable in the spine.27 This premenopausal loss of bone in the hip may be related to marginal estrogen deficiency or decreased physical activity.28 Bone loss in the spine probably does not occur until after the fifth decade of life and is more dependent on the decreasing production of estrogen as women approach the menopause.28,29 Bone loss in the spine in men also may begin at this age but occurs at a slower rate and without the accelerated loss that is typically seen in menopausal women.
Net loss of bone may be a universal phenomenon with increasing age, at least in humans.30 Whether such bone loss with increasing age occurs in other animal species is unclear. This is an important issue, because unique facets of human life may have consequences for skeletal health. The rate of bone loss is determined by endocrine, environmental, and various local factors, and probably also by genetic influences. Eskimos, for example, have rapid age-related bone loss and a high frequency of fracture.31 Those traits probably have both environmental and genetic causes.
The adult skeleton undergoes a process of remodeling by which old bone is removed and replaced by new, young bone.32 Before menopause, the bone that is removed is replaced by an equal amount of new bone tissue, and the frequency with which bone remodeling units are initiated is constant. With estrogen deficiency in women, and with increasing age in both sexes, changes occur in the remodeling process.33 Across menopause, an increase is seen in the number of new bone remodeling units initiated per unit time. This creates a transient loss of bone mass. For continued loss to occur, however, an imbalance must exist between formation and resorption within each remodeling unit.34 This can occur for one of several reasons: (a) more bone may be removed by more aggressive osteoclasts, (b) insufficient new bone may be laid down by new osteoblasts, (c) a combination of these two may occur, or (d) aggressive osteoclasts may penetrate trabeculae, removing the template on which new bone may be laid down. Variable combinations of these effects probably occur.
In postmenopausal women, the rate at which new remodeling sites are initiated increases. The suggestion has been made that the osteoclast population is hyperactive, leading to excessive bone resorption.35 A combination of these effects results in a net bone loss within each remodeling site and a stochastic probability of increased trabecular penetration. In glucocorticoid-induced osteoporosis, the same effects occur but are exacerbated by impaired osteoblast activity.36 This remodeling process takes place on the surface of cancellous bone at the endocortical junction and within the cortex of bone where the completed remodeling process is seen as Haversian systems. The fact that the majority of the activity occurs on the surface means that, when situations of imbalance occur, the loss of tissue is greatest at high surface areas and, within sites of equal surface, is highest at sites within red marrow in juxtaposition.37 Consequently, in any situation in which excessive bone loss occurs, bone loss occurs first and most markedly in cancellous bone, which contributes 80% of the surface area of the skeleton but only 20% of the skeletal mass.
In healthy individuals, the process of bone loss is insidious, occurring over many years in an asymptomatic fashion. The average negative calcium balance of 30 to 50 mg per day after three decades can cause as much as 30% to 50% loss of skeletal mass. Superimposed on these slow changes are the endocrine changes at menopause, in which a loss of estrogen produces increased bone remodeling.33,34,35,36,37 and 38 After spontaneous or surgical menopause, vertebral bone loss rates of 3% to 5% per year have been recorded for the first 5 to 10 years. Thereafter, the process of bone loss becomes slower, but it continues in many people until old age. Some indications are found that bone loss may accelerate in the very old individual.39 Menopause-related changes in bone remodeling are clearly triggered by the declining estrogen level.40 The mechanisms by which estrogen deficiency induces bone loss are only now beginning to be understood. Estrogen receptors, both ER-α and ER-β have been detected in a variety of cells within marrow and on cells of osteoblast and osteoclast lineage.41,42 and 43 However, whether the estrogen effects are mediated through ER-α or ER-β in the skeleton is unknown. Multiple target cells for estrogens probably exist within the skeleton, including macrophages, monocytes, lymphocytes, and other cells within marrow, as well as osteoblasts, preosteoclasts, and osteoclasts. In addition, osteocytes may be target cells for estrogen. Consequently, the fact that estrogen actions depend on a variety of cell-cell interactions, and a release of a variety of local cytokines and growth factors, is not surprising.44 Thus, implicated in the estrogen actions on the skeleton are interleukins 1, 6, and 11, prostaglandin E2, tumor necrosis factor-α (TNF-α, transforming growth factor-β (TGF-β, insulin-like growth factor-I (IGF-I), and perhaps also IGF-II.45,46,47,48,49,50,51,52,53,54,55,56 and 57 Moreover, direct effects of estrogen may include control of cell life span for osteoblasts, osteoclasts, and/or osteocytes, and control of the synthesis of new osteoclasts via osteoclast differentiation factor (or RANK ligand), which could be the final effectors of estrogen action in the recruitment process of new osteoclasts.58,59 and 60 Effects on other estrogen-responsive genes (e.g., c-phos and c-jun) have not been integrated into these processes.61
The consequence of the changes in the bone turnover with estrogen deficiency can be detected by the increased mean and
median levels of bone biochemical markers in serum and urine.62,63,64,65,66,67,68,69,70,71,72,73 and 74 The ranges of these marker levels are also increased; many individuals still have levels of biochemical markers within the expected premenopausal range, whereas others have levels that are distinctly elevated. Thus, the suggestion has been made that measurement of biochemical marker levels may provide a useful test for determining the rates of bone loss. However, although several studies have examined the relationship between baseline biochemical marker values and changes in bone mineral density (BMD) in untreated postmenopausal women, in general, the relationships are modest; biochemical marker levels are inadequate for clinical use in determining whether individual patients will lose bone rapidly.70,71 and 72 Although increased levels of biochemical markers do indicate the increased remodeling activity within the skeleton, the inherent intraindividual variability of marker levels probably limits their usefulness.73
median levels of bone biochemical markers in serum and urine.62,63,64,65,66,67,68,69,70,71,72,73 and 74 The ranges of these marker levels are also increased; many individuals still have levels of biochemical markers within the expected premenopausal range, whereas others have levels that are distinctly elevated. Thus, the suggestion has been made that measurement of biochemical marker levels may provide a useful test for determining the rates of bone loss. However, although several studies have examined the relationship between baseline biochemical marker values and changes in bone mineral density (BMD) in untreated postmenopausal women, in general, the relationships are modest; biochemical marker levels are inadequate for clinical use in determining whether individual patients will lose bone rapidly.70,71 and 72 Although increased levels of biochemical markers do indicate the increased remodeling activity within the skeleton, the inherent intraindividual variability of marker levels probably limits their usefulness.73
The consequence of this increased skeletal remodeling is loss of bone mass. As noted, loss of bone occurs earliest and most rapidly in areas of cancellous bone, which can be seen in the vertebral body. The risk of fracture increases with declining bone mass.74,75,76,77,78,79,80 and 81 That women who lose bone at the greatest rate will have the greatest deterioration in skeletal architecture,82 and subsequently be at even higher risk of fracture regardless of bone density, seems logical. This suggests that biochemical markers should correlate with the risk of fracture. Several studies have now indicated that biochemical marker levels do predict the risk of fracture in several populations.83,84,85 and 86 In addition, the suggestion has been made that biochemical markers of bone resorption predict the risk of fracture independently of bone mass and that the two effects are additive.83,87 However, this combination of tests has yet to be applied clinically.
Bone loss continues among postmenopausal women and becomes more apparent in men older than age 60 years.39,88 Some have argued that this process of bone loss in older individuals is related to features other than estrogen deficiency.89,90 Data have shown, however, that rates of bone loss in this population are dependent on endogenous estrogen production and that estrogen intervention prevents bone loss in the elderly, just as it does in women in the early years after the menopause.91 Thus, these findings suggest that at least part of the process of bone loss in elderly individuals is estrogen sensitive.
The report of a man with estrogen resistance due to absence of the estrogen receptor who presented with osteoporosis and continued growth because of failure of the epiphyses to fuse suggests that, in men, estrogen is responsible for control of skeletal homeostasis and may also be important in determining rates of bone loss.92 Declining ability to aromatize androgens to estrogen may also contribute to bone loss in aging men.93
One alternative hypothesis regarding bone loss after menopause is that a primary renal leak of calcium occurs, with increased obligatory loss of calcium that is offset by an increased skeletal remodeling required to maintain serum calcium.94 This hypothesis has some biologic plausibility, because hypercalciuria is a known risk factor for osteoporosis,95 particularly among men.96 Some studies have suggested that the increased renal loss of calcium may be estrogen dependent in women and, thus, a feature of postmenopausal bone loss.94 Thus estrogen deficiency may affect mineral metabolism. The net effect is to increase the likelihood that bone will be resorbed more easily by any stimulus to remodeling.97 Bone is less resistant to the resorbing effects of parathyroid hormone (PTH) infusion in the estrogen-deficient state, confirming this hypothesis.98
OTHER RISK FACTORS
A large number of risk factors have been implicated in post-menopausal osteoporosis (Table 64-2).99 Some of these affect bone mass and its loss, thereby altering fracture risk, whereas others modify the risk of fracture independently of bone mass. Some risk factors may have effects on both bone mass and fracture risk. One example is cigarette use, which may affect bone turnover and the age of menopause, and, through its harmful effects on general health, may independently raise fracture risk.100 More conveniently, from the clinician’s viewpoint, risk factors are usually categorized conceptually into those that are modifiable and those that are not. Although all are important in determining an individual’s likelihood of fracture, it is the former that must be addressed when making treatment decisions.
Nonmodifiable Risk Factors.
Of the factors that influence risk and cannot be changed, age is the single most important.101,102 and 103 In addition, gender, a family history of osteoporosis, and a past history of fracture affect risk.101,102,103 and 105 In most societies, fractures occur more commonly in women, and in white and Asian groups in most Western countries, female gender doubles fracture
risk. The same appears true for the black population, although the absolute risk is significantly lower for blacks than for those of other races.106 In the United States, the risk of fracture among black women is roughly equal to that among white males. Not surprisingly, persons with a family history of hip fracture in a parent are at approximately twice the risk of others for hip fracture.101 Data suggest that those with a family history of osteoporosis have lower peak bone mass, and parental bone mass is correlated with bone mass in offspring.107,108,109 and 110
risk. The same appears true for the black population, although the absolute risk is significantly lower for blacks than for those of other races.106 In the United States, the risk of fracture among black women is roughly equal to that among white males. Not surprisingly, persons with a family history of hip fracture in a parent are at approximately twice the risk of others for hip fracture.101 Data suggest that those with a family history of osteoporosis have lower peak bone mass, and parental bone mass is correlated with bone mass in offspring.107,108,109 and 110
The clinical experience that patients who present with fractures are more likely to fracture in the future has been confirmed by several formal studies.101,111 The view is now generally accepted that those who present with a history of fracture are at approximately twice the risk of future fracture, an increase in risk that is independent of bone mass. The occurrence of vertebral fractures dramatically increases the risk of subsequent vertebral fractures by five-fold for one fracture and by 12-fold for two or more fractures, while doubling the risk for other osteoporotic fractures.111
Modifiable Risk Factors.
Although the results of studies evaluating those factors that might be modifiable in any individual patient have varied, some broad agreement exists regarding evaluations for fracture risk. Furthermore, a large number of chronic diseases and medications have been associated with an increased risk of osteoporosis (see Table 64-2).
NUTRITION.
Good nutrition is as important for skeletal health as it is for general health.112 During growth, an adequate supply of calories, protein, and minerals is a prerequisite for the attainment of peak skeletal mass.18,26,27,112,113 Although the effect has not been proven by long-term studies, undernutrition in childhood leads to a skeletal deficit that cannot be repaired during adult life and presumably, therefore, would lead to an increase in the risk of osteoporotic fracture in later life. During adult life, an adequate intake of calcium is required to ensure that serum calcium can be maintained within the normal range (Table 64-3). Inadequate intake necessitates the use of calcium from the skeleton to maintain levels of serum calcium and leads to a consequent increase in bone turnover that results in a loss of skeletal mass.38 The effects of inadequate calcium intake are likely to be less among premenopausal women with adequate ovarian function than among women in an estrogen-deficient state.114,115 and 116 In the early postmenopausal years, the effects of estrogen deficiency are sufficiently potent that calcium supplementation has only modest effects.117 As individuals age, however, the role of calcium appears to become more important.118 Thus, studies of calcium supplementation conducted among older individuals show significant beneficial effects on bone mass and the incidence of fractures.119,120,121,122,123,124,125,126 and 127 The message to patients must be that calcium intake is important at all ages.
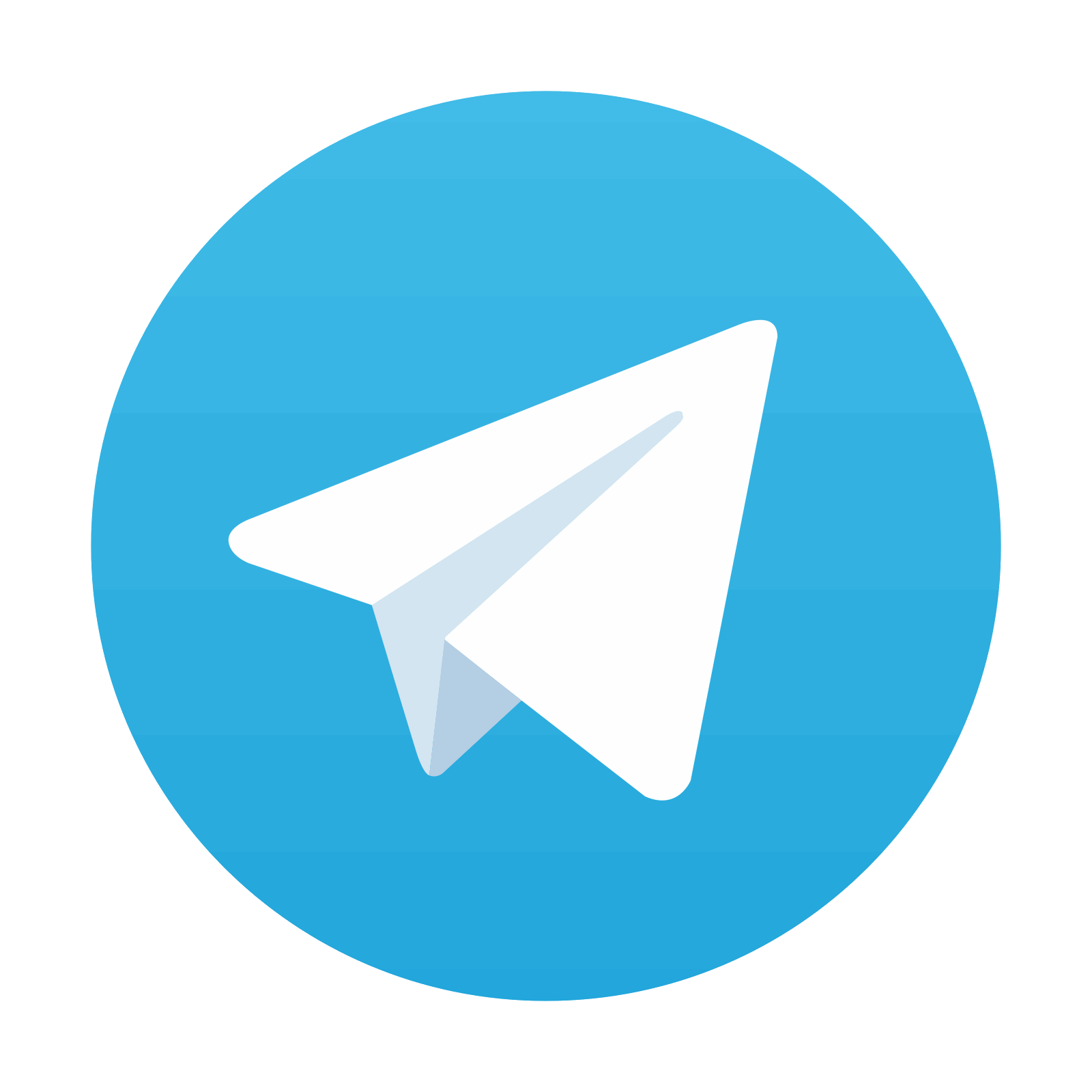
Stay updated, free articles. Join our Telegram channel

Full access? Get Clinical Tree
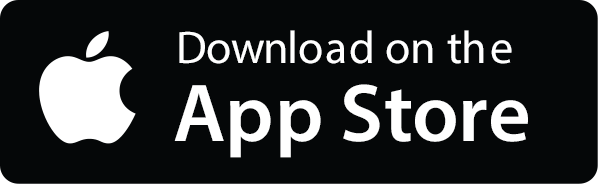
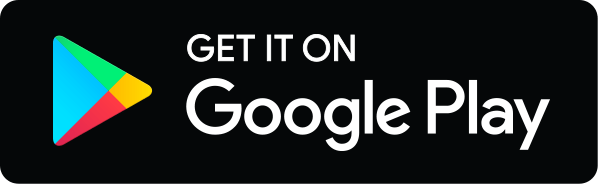
