SUMMARY
Venous thromboembolism, consisting of deep vein thrombosis and/or pulmonary embolism, is a common disorder with an estimated 900,000 patients each year in the United States and more than 1 million each year in the European Union. Approximately one-third of these cases are fatal pulmonary emboli, and the remaining two-thirds are nonfatal episodes of symptomatic deep vein thrombosis or pulmonary embolism. The majority of fatal events occur as sudden death, underscoring the importance of prevention as the critical strategy for reducing death from pulmonary embolism. Of the nonfatal cases, approximately 60 percent present clinically as deep vein thrombosis and 40 percent present as pulmonary embolism. Most clinically important pulmonary emboli arise from proximal deep vein thrombosis of the leg (popliteal, femoral, or iliac vein thrombosis). Upper-extremity deep vein thrombosis also may lead to clinically important pulmonary embolism. The clinical features of deep vein thrombosis and pulmonary embolism are nonspecific. Objective diagnostic testing is required to confirm or exclude the presence of venous thromboembolism. A validated assay for plasma D-dimer, if available, provides a simple, rapid, and cost-effective first-line exclusion test in patients with low, unlikely, or intermediate clinical probability. Compression ultrasonography is highly sensitive and specific for clinically important deep vein thrombosis and is the primary imaging test for symptomatic patients. Compression ultrasonography of the proximal veins performed at presentation, and if normal, repeated once 5 to 7 days later can safely exclude clinically important deep vein thrombosis. In centers with the expertise, a single comprehensive evaluation of the proximal and calf veins with duplex ultrasonography is sufficient. In patients with suspected pulmonary embolism, computed tomographic angiography, with or without additional testing using computed tomographic venography or compression ultrasonography of the legs, provides a definitive basis to give or withhold antithrombotic therapy in 90 percent of patients. Anticoagulant therapy is the preferred treatment for most patients with acute venous thromboembolism. Initial treatment with heparin or low-molecular-weight heparin, followed by long-term treatment with an oral vitamin K antagonist such as warfarin, is highly effective for preventing recurrent venous thromboembolism and has been the traditional standard of care. More recently, the direct oral anticoagulants, including the thrombin inhibitor dabigatran and the factor Xa inhibitors rivaroxaban, apixaban, and edoxaban, have been established to be as effective as and safer than traditional standard anticoagulant therapy. Rivaroxaban and apixaban can be used as a single-drug approach. Dabigatran and edoxaban are preceded by at least 5 days of heparin or low-molecular-weight heparin treatment. The direct oral anticoagulants are preferred over the vitamin K antagonists in most new patients commencing anticoagulant therapy. In cancer patients with venous thromboembolism, treatment with low-molecular-weight heparin for at least 6 months is the recommended approach. Thrombolytic therapy is indicated for patients with pulmonary embolism who present with hypotension or shock and in selected patients who have impaired right ventricular function who are at high risk of hemodynamic collapse. Insertion of a vena cava filter is indicated for patients who have an absolute contraindication to anticoagulant therapy or who have recurrent venous thromboembolism despite adequate anticoagulant treatment. Anticoagulant treatment should be continued for at least 3 months in all patients, and treatment duration of 3 months is sufficient for patients with first episode of venous thromboembolism secondary to a reversible risk factor. Indefinite anticoagulant therapy should be considered for patients with unprovoked (idiopathic) venous thromboembolism and those with recurrent venous thromboembolism.
Acronyms and Abbreviations:
aPTT, activated partial thromboplastin time; CDT, catheter-directed thrombolysis; CI, confidence interval; CT, computed tomography; CTA, computed tomographic angiography; CTV, computed tomographic venography; DOAC, direct-acting oral anticoagulant; DVT, deep vein thrombosis; ELISA, enzyme-linked immunosorbent assay; INR, international normalized ratio; LMW, low molecular weight; PCDT, pharmacomechanical catheter-directed thrombolysis; PE, pulmonary embolism; PIOPED, Prospective Investigation of Pulmonary Embolism Diagnosis; VTE, venous thromboembolism.
Venous thrombosis commonly develops in the deep veins of the leg or the arm or in the superficial veins of these extremities. Venous thrombosis of superficial veins is a relatively benign disorder unless extension into the deep venous system occurs. Confusingly, one of the major deep veins in the leg is called the superficial femoral vein. Thrombosis involving the deep veins of the leg is divided into two prognostic categories: (1) calf vein thrombosis, in which thrombi remain confined to the deep calf veins, and (2) proximal vein thrombosis, in which thrombosis involves the popliteal, femoral, or iliac veins.1
Pulmonary emboli originate from thrombi in the deep veins of the leg in 90 percent or more of patients. Other less common sources of pulmonary embolism (PE) include the deep pelvic veins, renal veins, inferior vena cava, right side of the heart, and axillary veins. Most clinically important PE arise from proximal deep vein thrombosis (DVT) of the leg. Upper-extremity DVT also may lead to important PE.2 DVT and/or PE are referred to collectively as venous thromboembolism (VTE).
VTE is a common disorder.3 The estimated annual incidence of clinically evident VTE ranges between 0.75 and 2.7 per 1000 population based on studies done in North America, Western Europe, Australia, and Argentina.3 The literature indicates a strong and consistent association of increasing incidence of VTE with increasing age. The annual incidence increased to between two and seven per 1000 population among those 70 years of age and to between three and 12 per 1000 population among those 80 years of age or older.3 Although the incidence is lower in individuals of Chinese and Korean ethnicity,3 their disease burden is not low because of population aging. The high incidence of VTE in the elderly likely reflects the high prevalence of comorbid acquired risk factors in these patients, especially malignancy, heart failure, and surgery or hospitalization for medical illness, which account for the majority of the population-attributable risk of VTE in older individuals.
VTE causes a major burden of disease across low-, middle-, and high-income countries. VTE associated with hospitalization was the leading cause of premature death and years lived with disability in low- and middle-income countries and the second-highest cause in high-income countries and is responsible for more premature death and disability than nosocomial pneumonia, catheter-related bloodstream infections, and adverse drug events.3
The direct ascertainment of deaths from VTE is difficult because of the low rate of autopsy in most countries and because autopsy studies have consistently demonstrated that PE is often not diagnosed antemortem. The strongest evidence comes from the study by Cohen and colleagues, who used an incidence-based model in six European countries to estimate that there were 534,454 deaths related to VTE across the European Union in 2004.4 A similar approach applied to the data from the United States suggested approximately 300,000 deaths from VTE each year.5 The majority of deaths from VTE occur as sudden death, underscoring the critical role of prevention for reducing death from VTE.
Effective prophylaxis against VTE is available for most high-risk patients. Use of prophylaxis is more effective for preventing death and morbidity from VTE than is treatment of the established disease. Evidence-based recommendations for prevention are available.6–9 Multifaceted interventions with alerts, such as computerized reminders or stickers on patient charts, are effective for increasing the prescription of appropriate thromboprophylaxis in hospitalized adult medical or surgical patients.10 There is also evidence that inclusion of VTE risk assessment at the time of hospital admission and the provision of appropriate prophylaxis are effective for reducing VTE-related death and readmission with nonfatal VTE.11,12 All hospitals should routinely assess the risk of VTE in all patients at the time of admission and provide thromboprophylaxis appropriate for the patient’s risk.12a Historically, the majority of the disease burden from VTE occurred in hospitalized patients. The burden of illness from VTE has shifted to the community setting such that most patients now present as outpatients to their primary care physician or to the emergency department. The main reason for this shift is the greatly reduced length of hospital stay for most surgical procedures or medical conditions, such that patients are discharged from the hospital before the period of risk of VTE has ended or patients are discharged who have subclinical venous thrombi that subsequently evolve to symptomatic DVT or PE. The shift in burden of illness from the hospital to the community setting has led to an emphasis on effective and safe methods for outpatient prophylaxis, diagnosis, and treatment.
The recently completed APEX trial12b is an important advance toward reducing the community burden of VTE. This randomized trial evaluated extended thromboprophylaxis (35 to 45 days) using the oral factor Xa inhibitor betrixaban with the current standard practice of prophylaxis with low-molecular-weight heparin given for 10 to 14 days among 7513 patients who were hospitalized for acute medical illness and who were at high risk for VTE based on the presence of multiple clinical risk factors or an elevated plasma D-dimer level. Extended thromboprophylaxis resulted in a 24 percent relative risk reduction, from 7.0 percent to 5.3 percent (p = 0.006), in the composite outcome of asymptomatic proximal vein thrombosis, symptomatic DVT or PE, or VTE-related death.12b Symptomatic VTE was reduced from 1.5 percent to 0.9 percent (relative risk reduction 36 percent, p = 0.04) by extended thromboprophylaxis. Major bleeding was not increased with the use of extended thromboprophylaxis compared to prophylaxis given for 10 to 14 days (rates of 0.6 percent and 0.7 percent, respectively). Fatal bleeding was rare and occurred in only one patient of 3716 in each group, whereas VTE-related death occurred in 26 patients (0.7 percent) in the 10- to 14-day prophylaxis group and in 15 patients (0.4 percent) in the extended prophylaxis group. Thus, VTE is a much more common cause of mortality in this population than bleeding. Extended thromboprophylaxis with betrixaban was safe and resulted in an important reduction in clinically important VTE from both an individual patient perspective and from a population health perspective (number needed to treat of 59 to prevent one event of proximal vein thrombosis, symptomatic VTE, or VTE-related death). Given the many millions of patients admitted to hospital each year for acute medical conditions, extended thromboprophylaxis in this population can produce an important reduction in the burden of disease from VTE.
Venous thrombi are composed mainly of fibrin and red blood cells, with variable numbers of platelets and leukocytes. The formation, growth, and breakdown of venous thromboemboli reflect a balance between thrombogenic stimuli and protective mechanisms. The thrombogenic stimuli first identified by Virchow in the 19th century are (1) venous stasis, (2) activation of blood coagulation, and (3) vascular damage. The protective mechanisms are (1) inactivation of activated coagulation factors by circulating inhibitors (e.g., antithrombin and activated protein C), (2) clearance of activated coagulation factors and soluble fibrin polymer complexes by mononuclear phagocytes and the liver, and (3) lysis of fibrin by fibrinolytic enzymes derived from plasma and endothelial cells.
PE occurs in at least 50 percent of patients with documented proximal vein thrombosis.1 Many of these emboli are asymptomatic. The clinical importance of PE depends on the size of the embolus and the patient’s cardiorespiratory reserve. Usually only part of the thrombus embolizes, and 30 to 70 percent of patients with PE detected by angiography also have identifiable DVT of the legs.13,14 DVT and PE are not separate disorders but a continuous syndrome of VTE in which the initial clinical presentation may be symptoms of either DVT or PE. Therefore, strategies for diagnosis of VTE include both tests for detection of PE (e.g., computed tomography [CT] or lung scanning)13–16 and tests for DVT of the legs (e.g., ultrasonography)17–19 (see “Objective Testing for Pulmonary Embolism” and “Objective Testing for Deep Vein Thrombosis” below).
Acquired and inherited risk factors for VTE have been identified20–23 and are shown in Table 23–1 (Chap. 20). Aging is the dominant risk factor for VTE (population attributable risk >90 percent).23 Comorbidities, such as malignancy and heart failure, contribute to a higher population-attributable risk in older patients (≥65 years).23 The risk of VTE increases when more than one risk factor is present.24
Acquired | Hereditary Thrombophilias* |
---|---|
Advancing age (age >40 years) | Activated protein C resistance |
History of prior thromboembolic event | Prothrombin G20210A mutation |
Recent surgery | Antithrombin deficiency |
Recent trauma | Protein C deficiency |
Prolonged immobilization | Protein S deficiency |
Certain forms of cancer | Dysfibrinogenemia |
Congestive heart failure | |
Recent myocardial infarction | |
Paralysis of legs | |
Use of female hormones | |
Pregnancy or postpartum period | |
Varicose veins | |
Obesity | |
Antiphospholipid antibody syndrome** | |
Hyperhomocysteinemia |
Activated protein C resistance is the most common hereditary abnormality predisposing to VTE. The defect results from substitution of glutamine for arginine at residue 506 in the factor V molecule, making factor Va resistant to proteolysis by activated protein C. The gene mutation is commonly designated factor V Leiden and follows autosomal dominant inheritance. Patients who are homozygous for the factor V Leiden mutation have a markedly increased risk of VTE and present with clinical thromboembolism at a younger age (median age: 31 years) than those who are heterozygous (median age: 46 years).20,22 Factor V Leiden is present in approximately 5 percent of the normal population of European descent, 16 percent of patients with a first episode of DVT, and up to 35 percent of patients with unprovoked (idiopathic) DVT.20,22,25 Prothrombin G20210A is another common gene mutation that predisposes to VTE. It is present in approximately 2 to 3 percent of apparently healthy individuals and in 7 percent of those with DVT.22 An inherited abnormality cannot be detected in up to 40 to 50 percent of patients with unprovoked DVT, suggesting that as yet undefined gene mutations are present that have an etiologic role (Chap. 20).
The clinical features of DVT include leg pain, tenderness, and swelling, a palpable cord representing a thrombosed vessel, discoloration, venous distention, prominence of the superficial veins, and cyanosis. The clinical diagnosis of DVT is highly nonspecific because each of the symptoms or signs can be caused by nonthrombotic disorders. The rare exception is the patient with phlegmasia cerulea dolens (occlusion of the whole venous circulation, extreme swelling of the leg, and compromised arterial flow), in whom the diagnosis of massive iliofemoral thrombosis is obvious. This syndrome occurs in less than 1 percent of patients with symptomatic venous thrombosis. In most patients, the symptoms and signs are nonspecific. In 50 to 85 percent of patients, the clinical suspicion of DVT is not confirmed by objective testing.17–19 Patients with minor symptoms and signs may have extensive DVT. Conversely, patients with florid leg pain and swelling, suggesting extensive DVT, may have negative results by objective testing.
Although the clinical diagnosis is nonspecific, prospective studies have established that patients can be categorized as low, moderate, or high probability for DVT using a clinical prediction rule that incorporates signs, symptoms, and risk factors. A systematic review26 of the studies found that the prevalence of DVT in the low, moderate, and high probability categories, respectively, was 5 percent (95 percent confidence interval [95% CI]: 4 to 8 percent), 17 percent (95% CI: 13 to 23 percent), and 53 percent (95% CI: 44 to 61 percent). The prevalence in the pretest category of “low probability” is not sufficiently low to withhold further diagnostic testing and treatment, and the prevalence in the “high probability” category is not sufficiently high to give anticoagulant therapy without performing further diagnostic testing. Consequently, the key role for clinical pretest categorization is for use within integrated diagnostic strategies employing measurement of D-dimer and venous imaging.
The clinical features of acute PE include the following symptoms and signs that may overlap: (1) transient dyspnea and tachypnea in the absence of other clinical features; (2) pleuritic chest pain, cough, hemoptysis, pleural effusion, and pulmonary infiltrates noted on chest radiogram caused by pulmonary infarction or congestive atelectasis (also known as ischemic pneumonitis or incomplete infarction); (3) severe dyspnea and tachypnea and right-sided heart failure; (4) cardiovascular collapse with hypotension, syncope, and coma (usually associated with massive PE); and (5) several less common and nonspecific clinical presentations, including unexplained tachycardia or arrhythmia, resistant cardiac failure, wheezing, cough, fever, anxiety/apprehension, and confusion. All of these clinical features are nonspecific and can be caused by a variety of cardiorespiratory disorders. Patients can be assigned to categories of pretest probability using implicit clinical judgement or clinical decision rules such as the Geneva score or Wells approach.27–30 However, the prevalences of PE in these categories are not sufficiently low or high to withhold further investigation altogether, and the measurement of D-dimer and/or diagnostic imaging is mandatory to exclude or confirm the presence of PE. The assessment of clinical pretest probability is an important first step in integrated diagnostic strategies that employ, for example, D-dimer, computed tomographic angiography (CTA), and objective testing for DVT.27–30
VTE is associated with nonspecific laboratory changes that constitute the acute-phase response to tissue injury. This response includes elevated levels of fibrinogen and factor VIII, increases in leukocyte and platelet counts, and systemic activation of blood coagulation, fibrin formation, and fibrin breakdown, with increases in plasma concentrations of prothrombin fragment 1.2, fibrinopeptide A, complexes of thrombin–antithrombin, and fibrin degradation products. All of these changes are nonspecific and may occur as a result of surgery, trauma, infection, inflammation, or infarction. None of the above reported laboratory changes can be used to establish the diagnosis of VTE or predict its development with high probability. The fibrin breakdown fragment D-dimer can be measured by an enzyme-linked immunosorbent assay (ELISA) or by a latex agglutination assay. Some of these assays have a rapid turnaround time, and some are quantitative. A negative D-dimer result is useful for excluding the diagnosis in many patients with suspected DVT or suspected PE (see “Objective Testing for Deep Vein Thrombosis” and “Objective Testing for Pulmonary Embolism” below).16,27,28,31 A positive result is highly nonspecific.
The differential diagnosis in patients with clinically suspected DVT includes muscle strain or tear, direct twisting injury to the leg, lymphangitis or lymphatic obstruction, venous reflux, popliteal cyst, cellulitis, leg swelling in a paralyzed limb, and abnormality of the knee joint. An alternate diagnosis frequently is not evident at presentation, so excluding DVT is not possible without objective testing. The cause of symptoms often can be determined by careful follow-up once DVT has been excluded by objective testing. In approximately 25 percent of patients, however, the cause of pain, tenderness, and swelling remains uncertain even after careful follow-up.19
Measurement of plasma D-dimer has been extensively evaluated as an exclusion test in patients with clinically suspected DVT.31 The different D-dimer assays (ELISA, quantitative rapid ELISA, latex agglutination, and whole-blood agglutination) have different sensitivities, specificities, and likelihood ratios for DVT. ELISA and quantitative rapid ELISA have high sensitivity (96 percent) and negative likelihood ratios of approximately 0.10 for DVT in symptomatic patients. Thus, for excluding DVT in symptomatic patients with a low or intermediate clinical pretest probability, a negative D-dimer result by a quantitative rapid ELISA technique is as diagnostically useful as a negative result by duplex ultrasonography.31 Measurement of D-dimer using an appropriate assay method can also be combined with ultrasonography imaging. If the two tests are negative at presentation, repeat ultrasonography imaging is unecessary.32 Use of the D-dimer test for patient care decisions depends on the local availability of an appropriate assay that has high sensitivity and has been validated by clinical outcome studies. The use of age-adjusted D-dimer cut-off levels for a negative result enhances the clinical utility of the test. Figure 23–1 shows a practical approach for the diagnosis of suspected DVT.
Figure 23–1.
Diagnosis of patients with suspected first episode of deep vein thrombosis (DVT). *Negative D-dimer can be used to exclude acute DVT, without the need for further diagnostic testing with compression ultrasonography (CUS), if the patient has low, unlikely, moderate, or intermediate clinical probability.26,31 Ultrasonography should be performed in patients with a high clinical probability. A negative D-dimer can also be used with a negative CUS at presentation to exclude acute DVT without the need for a repeat CUS.32,34 **CUS is performed with imaging of the common femoral vein in the groin and of the popliteal vein in the popliteal fossa extending distally 10 cm from midpatella. A repeat CUS is required in 5 to 7 days to detect extending calf vein thrombi.17 In centers with the expertise, a single negative result of full-leg duplex ultrasonography (CUS plus flow evaluation) is sufficient to exclude acute DVT.18,34 ‡CUS that indicates noncompressibility of deep vein segments is highly predictive of DVT (>95 percent) and provides an indication for antithrombotic therapy in most patients. If CUS is positive at a single site isolated in the groin, additional testing with venography, computed tomography, or magnetic resonance imaging should be performed because of the potential for false-positive CUS results from disorders producing vein compression in the groin (e.g., tumor mass).

The objective diagnostic imaging tests that have a role in patients with clinically suspected DVT are ultrasonography and venography. Both of these tests have been validated by properly designed clinical trials, including prospective studies with long-term follow-up that have established the safety of withholding anticoagulant treatment in patients with negative test results.17–19,33 Ultrasonography is the preferred imaging test for most patients. The role of venography is for selected patients, such as those in whom ultrasonography is unavailable or inconclusive. Ultrasonography using vein compression is effective for identifying patients with proximal vein thrombosis. Compression ultrasonography of the proximal veins performed at presentation (and, if normal, repeated once 5 to 7 days later) is a safe approach in symptomatic patients.17 In centers with experienced ultrasonography staff, a single comprehensive evaluation of the proximal and calf veins with duplex ultrasonography is sufficient, and if negative, a repeat test is not required.18 A randomized trial supports the equivalence of a comprehensive whole-leg color-coded Doppler ultrasonography approach to that of an approach using combined D-dimer testing and repeated ultrasonography for the management of suspected DVT.34
The positive predictive value of a positive ultrasonography result isolated to the calf veins may vary among centers based on expertise and thrombosis prevalence. Therefore, the number of repeat ultrasonography evaluations avoided by evaluating the calf veins may be partially offset by an increased number of patients with positive ultrasonography results confined to the calf, for whom additional diagnostic testing and/or anticoagulant treatment is required. Most patients with a negative ultrasonographic result at presentation require a follow-up visit to establish the alternate diagnosis and to guide further care, so the return visit for repeat ultrasonography at 5 to 7 days may have added practical value.17
Diagnosis of acute recurrent DVT is particularly challenging because recurrent symptoms such as pain and swelling are common in patients with DVT despite adequate anticoagulant therapy and because both ultrasonography and venography have limitations for excluding the presence of acute recurrent DVT.35 Compression ultrasonography may remain abnormal for 1 year in 50 percent of patients, and for even longer in some patients,36 because of persistent noncompressibility of the vein caused by fibrous organization of the original thrombus. Venography is of limited value for excluding the diagnosis of recurrent DVT because of obliteration or recanalization of the previously affected venous segments or nonfilled venous segments. Thus, measurement of plasma D-dimer may be particularly useful as an exclusion test in patients with suspected acute recurrent DVT. However, use of D-dimer must be evaluated separately in this patient group because many patients with a past history of VTE are receiving long-term oral anticoagulant therapy, which has the potential to cause a false-negative D-dimer result. Promising initial results were obtained in one study,37 but further studies in larger numbers of patients are needed before using a negative D-dimer alone to exclude acute recurrent DVT can be routinely recommended.
The differential diagnosis in patients with suspected PE includes cardiopulmonary disorders for each of the modes of presentation (see “Pulmonary Embolism” above). For the presentation of dyspnea and tachypnea, they include atelectasis, pneumonia, pleuritis, pneumothorax, acute pulmonary edema, bronchitis, bronchiolitis, and acute bronchial obstruction. For pulmonary infarction exhibited by pleuritic chest pain or hemoptysis, they include pneumonia, pneumothorax, pericarditis, pulmonary or bronchial neoplasm, bronchiectasis, acute bronchitis, tuberculosis, diaphragmatic inflammation, myositis, muscle strain, and rib fracture. For the clinical presentation of right-sided heart failure, they include myocardial infarction, myocarditis, and cardiac tamponade. For cardiovascular collapse, they include myocardial infarction, acute massive hemorrhage, gram-negative septicemia, cardiac tamponade, and spontaneous pneumothorax.
The objective diagnostic imaging tests include CT, CTA, radionuclide lung scanning, selective pulmonary arteriography, and objective testing for DVT. Measurement of plasma D-dimer is useful as an exclusion test in patients with an unlikely or intermediate clinical probability.
The assay for plasma D-dimer is useful as an exclusion test, provided an appropriately validated test is available. A negative result by the rapid quantitative ELISA for D-dimer has a negative likelihood ratio similar to that of a normal perfusion scan.31 A positive D-dimer result is not useful diagnostically. Several management studies have found that PE can be excluded without performing imaging studies in patients with a low, intermediate, or unlikely clinical probability.38 When combined with pretest clinical probability assessment, the use of an age-adjusted D-dimer cutoff value, instead of a fixed D-dimer cutoff of 500 mcg/mL, improves the utility of the test, and enables more patients to have the diagnosis of PE safely excluded.39
CT imaging is the primary imaging test for the diagnosis of PE in most centers. Single-detector spiral CT is highly sensitive for large emboli (segmental or larger arteries) but is much less sensitive for emboli in subsegmental pulmonary arteries16,40; such emboli may be clinically important in patients with severely impaired cardiorespiratory reserve. Therefore, a negative result by single-detector spiral CT should not be used alone to exclude the diagnosis of PE. A filling defect of a segmental or larger artery on single-detector spiral CT is associated with a high probability (>90 percent) of PE.40
The development of multidetector row CT, together with the use of contrast enhancement, has established CT as the preferred diagnostic imaging test in most patients.41–43 Contrast-enhanced CTA has the advantage of providing clear results (positive or negative), with a low rate of nondiagnostic test results, good characterization of nonvascular structures for alternate or associated diagnoses, and the ability to simultaneously evaluate the deep venous system of the legs (computed tomographic venography [CTV]).
The accuracy and clinical utility of multidetector CTA and combined CTA-CTV were evaluated in the Prospective Investigation of Pulmonary Embolism Diagnosis (PIOPED) II study.43 Among 824 patients with a reference diagnosis and a completed CT study, CTA was inconclusive in 51 (6 percent) because of poor image quality. The sensitivity of CTA was 83 percent and the specificity was 96 percent. CTA-CTV was inconclusive in 87 (11 percent) of 824 patients because the image quality of either CTA or CTV was poor. Multidetector CTA-CTV had a higher sensitivity (90 percent) than CTA alone (83 percent), with similar specificity (~95 percent for both testing techniques). Positive results on CTA in combination with a high probability or intermediate probability of PE by the clinical assessment or normal findings on CTA with a low clinical probability had a predictive value (positive or negative) of 92 to 96 percent.43 Such values are consistent with those generally considered adequate to confirm or rule out the diagnosis of PE. Additional testing is necessary when the clinical probability is discordant with CTA or CTA-CTV imaging results.43
Figure 23–2 summarizes the approach to diagnosis of suspected PE using CTA or CTA-CTV as the primary imaging test. A high-quality image by CTA is sufficient to establish or exclude the diagnosis of PE with high predictive value in most patients, and withholding anticoagulant therapy based on a negative CTA alone is associated with a low rate of subsequent VTE on follow-up.44 Objective testing for DVT is useful in patients in whom the CTA image is of poor quality or inconclusive and in patients who also have symptoms suggesting DVT. CTV has the advantage of being easily performed at the time of CTA, but incurs the risk of added radiation exposure for the patient. Compression ultrasonography can also be used and avoids added radiation exposure and can be performed serially if needed.
Figure 23–2.
Integrated strategy for diagnosis of patients with suspected pulmonary embolism (PE) using computed tomographic angiography (CTA) as the primary imaging test. *Negative D-dimer alone can be used as an exclusion test with high negative predictive value (>96 percent) in patients with low or moderate probability by the clinical assessment.27,30,31 Patients with a high clinical probability should undergo imaging with CTA or combined CTA-computed tomographic venography (CTV). **Positive results on CTA or combined CTA-CTV, in patients with a high or moderate probability of pulmonary embolism by the clinical assessment, have positive predictive value of 90% or more for venous thromboembolism. Similarly, abnormal results by compression ultrasonography (CUS) of the proximal deep veins of the legs have high positive predictive value for proximal vein thrombosis and provide an indication to give antithrombotic therapy. If the patient has a low probability by the clinical assessment, positive results by CTA or CTA-CTV in the main or lobar pulmonary arteries are still highly predictive (97 percent) for the presence of pulmonary embolism43; further testing is recommended for patients with low clinical probability and positive CTA results only of segmental or subsegmental arteries, and the options include pulmonary arteriography or serial CUS. ‡Negative results by CTA or by combined CTA-CTV have high negative predictive value (96 percent) in patients with low probability by the clinical assessment.43 For patients with moderate clinical probability, the negative predictive value for combined CTA-CTV is also high (92 percent), but slightly lower for CTA alone (89 percent)43; in CTA-alone group and in patients with a high probability by the clinical assessment, serial CUS and pulmonary arteriography are recommended options.

Radionuclide lung scanning continues to have a role in the diagnosis of suspected PE. A normal perfusion lung scan excludes the diagnosis of clinically important PE.15,45 A normal perfusion lung scan is found in approximately 10 percent of all patients with suspected PE seen at academic health centers or tertiary referral centers. A high-probability lung scan result (i.e., large perfusion defects with ventilation mismatch) has a positive predictive value for PE of 85 percent and provides a diagnostic end point to give antithrombotic treatment in most patients.15,46,47 A high-probability lung scan is found in approximately 10 to 15 percent of symptomatic patients. For patients with a history of PE, careful comparison of the lung scan results to the most recent lung scan is required to ensure the perfusion defects are new. Further diagnostic testing is indicated for patients with a high-probability lung scan who have a “low” pretest clinical suspicion, and in those who are at high risk for major bleeding, to reduce the likelihood of a false-positive diagnosis.
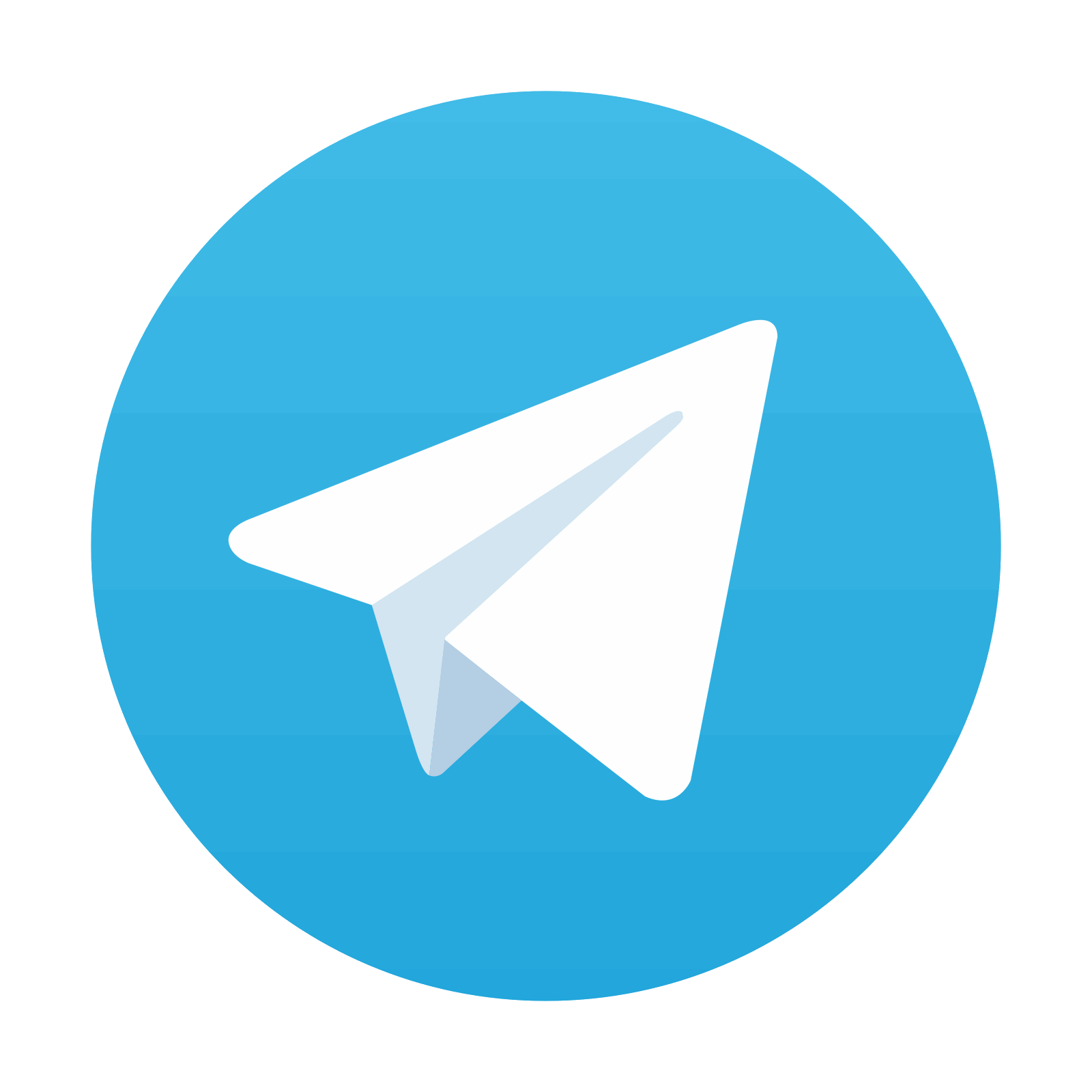
Stay updated, free articles. Join our Telegram channel

Full access? Get Clinical Tree
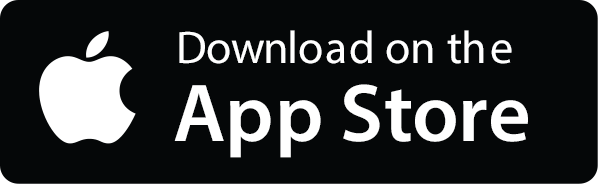
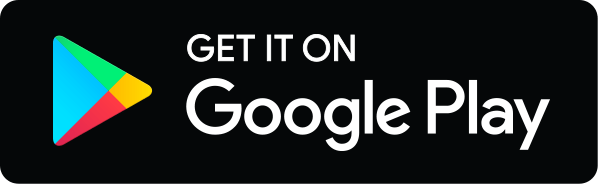