The potential for therapeutic efficacy of a melanoma vaccine has been evident preclinically for many years. In melanoma patients, vaccines have resulted in the induction of immune responses, although clinical benefit has not been clearly documented. The recent achievements with immune-checkpoint blockade have shown that immunotherapy can be a powerful tool in cancer therapy. With increased understanding of tumor immunity, the limitations of previous cancer vaccination approaches have become evident. Rapid progress in technologies that enable better vaccine design raise the expectation that these limitations can be overcome, thus leading to a clinically effective melanoma vaccine in the near future.
Key points
- •
The potential for therapeutic efficacy of a melanoma vaccine has been evident preclinically for many years.
- •
In patients with melanoma, vaccines have resulted in the induction of immune responses, although clinical benefit has not been clearly documented.
- •
The recent achievements with immune-checkpoint blockade, such as anti–CTLA-4 and anti–PD-1/PD-L1 in melanoma and other cancers, have illustrated that immunotherapy can be a powerful tool in cancer therapy.
- •
With increased understanding of tumor immunity, the limitations of many previous cancer vaccination approaches have become evident.
- •
Rapid progress in technologies that enable better vaccine design raise the expectation that these limitations can be overcome, thus leading to a clinically effective melanoma vaccine in the near future.
Introduction
Vaccination against melanoma represents an effort to stimulate an antitumor immune response that is directed either against an established tumor in patients with unresectable metastatic disease, or against micrometastatic disease in patients who are at high risk for recurrence after surgical resection. In both situations, the host has already failed the task of cancer immunosurveillance. The reasons for this failure are either the complete lack of an antimelanoma immune response or tumor-mediated immune evasion, caused by numerous mechanisms that cancers can elaborate. A successful antitumor vaccine therefore will induce a de novo immune response or boost an ineffective existing response, reprogramming the (failed) immune response by providing it with new targets and stronger stimulation ( Fig. 1 ). Differences among strategies relate to the vehicle used to deliver the antigenic target, the antigenic target itself, the number of targets provided, and the appropriate immune-stimulating context (adjuvant). Checkpoint blockade, an antigen-independent immunotherapy, is designed to overcome 1 or more mechanisms of immune evasion, and provides exciting synergistic opportunities to strengthen vaccine therapy. Vaccination strategies offer the capacity for potentiated adaptive immune activation, antigen(s)/target specificity, multiarm immune engagement, and the realization of durable, effective immunologic memory, a hallmark of effective immunity.
No other tumor has been more thoroughly investigated with different vaccine approaches than melanoma. This article first summarizes prior efforts undertaken over the last 20 years, which demonstrated promise but were lacking in broad clinical activity. Further described are exciting new approaches that leverage recent technological advances with important biological insights to yield potential advances in this field.
Introduction
Vaccination against melanoma represents an effort to stimulate an antitumor immune response that is directed either against an established tumor in patients with unresectable metastatic disease, or against micrometastatic disease in patients who are at high risk for recurrence after surgical resection. In both situations, the host has already failed the task of cancer immunosurveillance. The reasons for this failure are either the complete lack of an antimelanoma immune response or tumor-mediated immune evasion, caused by numerous mechanisms that cancers can elaborate. A successful antitumor vaccine therefore will induce a de novo immune response or boost an ineffective existing response, reprogramming the (failed) immune response by providing it with new targets and stronger stimulation ( Fig. 1 ). Differences among strategies relate to the vehicle used to deliver the antigenic target, the antigenic target itself, the number of targets provided, and the appropriate immune-stimulating context (adjuvant). Checkpoint blockade, an antigen-independent immunotherapy, is designed to overcome 1 or more mechanisms of immune evasion, and provides exciting synergistic opportunities to strengthen vaccine therapy. Vaccination strategies offer the capacity for potentiated adaptive immune activation, antigen(s)/target specificity, multiarm immune engagement, and the realization of durable, effective immunologic memory, a hallmark of effective immunity.
No other tumor has been more thoroughly investigated with different vaccine approaches than melanoma. This article first summarizes prior efforts undertaken over the last 20 years, which demonstrated promise but were lacking in broad clinical activity. Further described are exciting new approaches that leverage recent technological advances with important biological insights to yield potential advances in this field.
Previous vaccine approaches in melanoma: some promise, but limited clinical activity
Immunogens
Most vaccine approaches to date have used whole proteins or peptide fragments as the immunogen, which must be delivered to professional antigen-presenting cells (APCs) such as dendritic cells (DCs), usually in conjunction with an immune-stimulating adjuvant to serve as an effective vaccine. The objective is antigen uptake, processing, and cross-presentation by APCs to effect priming of naïve T cells or stimulation of memory T cells. The appropriate immune adjuvant will provide the danger signal, promoting immune pathways analogous to those activated in response to bacterial or viral infection. Key considerations when designing an effective vaccine include format (ie, whole protein vs peptide), route of delivery, and choice of adjuvant. Regarding the former, factors include cost (advantage: peptide), in vivo stability (advantage: peptide), and breadth of antigenic selection (advantage: protein). A significant limitation to peptide vaccines is their restriction to specific human leukocyte antigen (HLA) haplotypes. Most vaccine developers to date have focused on peptides that bind to the predominant HLA allele (HLA-A2) to capture the largest target population possible with a single vaccine. Unfortunately, this strategy excludes a vast pool of potentially immunogenic peptides and a considerable proportion of melanoma patients. This drawback could partially be addressed by using longer peptides (containing more than 1 epitope) or the use of multiple peptides, but as yet no such research has been conducted.
Tumor-associated antigens (TAA) are developmental, differentiation, or growth-promoting proteins that are frequently overexpressed or somewhat specifically expressed by tumors. Much of cancer vaccinology for the past 20 years has focused on inducing or identifying T cells that recognize such proteins.
The first TAA for melanoma, MAGE-1, was uncovered in 1991, and the capacity to generate cytotoxic T lymphocytes that recognize the protein was simultaneously demonstrated. Following its discovery, vaccines specifically targeting MAGE-1, in addition to other subsequently identified antigenic targets, rapidly emerged. The first clinical trial targeting a TAA focused on the differentiation antigen glycoprotein 100 (gp100). Popular melanoma targets have since included additional gp100 epitopes, MART-1, MAGE-1, MAGE-3, tyrosinase, and NY-ESO-1.
Tumor-specific antigens (TSAs), derived from viral proteins or tumor-specific genetic mutations found in tumors, provide a recent opportunity to overcome many of the aforementioned immunogen issues, and will be discussed in more detail.
Clinical trials with TAA vaccines
No peptide or protein vaccine has demonstrated a clear improvement in overall survival in melanoma patients to date. For the most part, melanoma vaccines have targeted TAAs such as described above.
Small, single-arm studies
At the National Cancer Institute surgery branch 323 subjects, almost all with metastatic melanoma, received peptide vaccines on a variety of different protocols. The peptides were derived from various TAAs including MART-1, gp100, tyrosinase, TRP-2, NY-ESO-1, MAGE-1/3, Her2/neu, or telomerase proteins. Except for 15 patients who received peptides pulsed on DCs, peptides were emulsified in incomplete Freund adjuvant (IFA). Only 9 of these 323 subjects had a partial response and 2 had a complete response, resulting in an overall objective response rate of 2.9%. Of note, most of the responders had metastatic disease confined to skin and lymph nodes. Peptide vaccine studies in melanoma patients using multiple different epitopes, single peptide and multipeptide strategies, and different adjuvants such as granulocyte-macrophage colony-stimulating factor (GM-CSF), and low-dose interleukin (IL)-2 have produced similar results: low objective tumor response rates, generally from lower than 5% up to 10%, with variable effects on the immune response.
Larger, comparative studies
In a phase 2 cooperative group trial, 121 previously treated metastatic melanoma patients received 3 HLA-A2 restricted peptides (MART-1, gp100M, and tyrosinase) either alone or in combination with GM-CSF or interferon (IFN)-α, respectively. Six objective responses were observed, and a T-cell response measured by IFN-γ ELISPOT against at least 1 of the 3 peptides was seen in 26 of 75 (35%) patients with serial samples available. Patients with immune responses had improved overall survival (21.3 vs 13.4 months; P = .046).
In a phase 3 trial, stage IV melanoma patients were treated with a modified gp100 peptide with increased binding affinity to HLA-A2 (gp100M) in combination with high-dose IL-2 versus IL-2 alone. The objective tumor response rate was higher in the IL-2/gp100M combination arm in comparison with IL-2 alone (16 vs 6%, P = .03), and the progression-free survival was longer (2.2 vs 1.6 months, P = .008). There was also a trend toward improved overall survival (17.8 vs 11.1 months; P = .06). Noteworthy is that the objective response rate of 6% in the IL-2 arm is markedly lower than the 16% reported historically with this drug. Only a few patients in the gp100M + IL-2 arm developed gp100-specific T cells in the peripheral blood, and there was no correlation between gp100-specific peripheral T cells and clinical responses. The lack of correlation of clinical responses and documented immune responses targeting the vaccine suggest lack of vaccine efficacy.
In a series of 3 phase 2 studies conducted by the Cytokine Working Group, 132 HLA-A2–positive advanced melanoma patients received the gp100M peptide combined with high-dose IL-2 given on variable schedules. Given the strikingly similar response rates with gp100 plus IL-2 in the phase 2 and 3 trials and historical data with IL-2 alone (∼15%), it appears that the clinical benefit seen with gp100 plus IL-2 is mostly driven by the high-dose IL-2 and not an effect of the peptide vaccine. This finding is also in line with the phase 3 experience using the anti–cytotoxic T-lymphocyte antigen 4 (CTLA-4) antibody ipilimumab and gp100 in previously treated metastatic melanoma patients. In this trial, no difference in response rate or overall survival was seen between ipilimumab + gp100 when compared with ipilimumab alone. Together, these results suggest that gp100 is an ineffective vaccine as currently administered.
Full-length proteins may provide a more comprehensive spectrum of tumor epitopes for presentation to DCs. MAGE-A3 is a cancer testis antigen overexpressed in approximately 65% of melanomas. A recombinant MAGE-A3 vaccine consists of full-length MAGE-A3 protein fused to protein D (a lipoprotein on the surface of Haemophilus influenzae B) and a polyhistidine tail. This vaccine, given with the immune adjuvant ASO2B, consisting of a saponin/lipid-A emulsion combined with TLR4 and TLR9 agonists, is currently being tested in a prospective, randomized phase 3 study in patients with high-risk resected, MAGE-A3 positive melanoma. The coprimary end point of disease-free survival (DFS) was not met according to a recent announcement by the study’s sponsor, Glaxo Smith Kline.
Recombinant Viral Vector–Based Vaccines
Viral vectors encoding tumor antigens take advantage of immune responses against viral components, resulting in an adjuvant effect that can augment the antitumor-directed T-cell response. In melanoma, a viral-based vaccine approach that has been tested in phase 2 and 3 clinical trials consists of a second-generation herpes virus engineered to selectively replicate in and lyse tumor cells and to express GM-CSF, thereby attracting DCs into the tumor microenvironment (OncoVex GM−CSF ; BioVex, Cambridge, MA). Based on promising clinical activity in a multi-institutional phase 2 study in patients with unresectable stage IIIC or IV melanoma (8 complete and 5 partial responses in 50 patients), 436 patients with unresectable stage IIIB, IIIC, and IV melanoma were randomized 2:1 to receive either intratumoral OncoVex GM−CSF or GM-CSF given subcutaneously. In an interim analysis reported at the annual meeting of the American Society of Clinical Oncology 2013, the primary end point of increased durable response rate of OncoVex GM−CSF over GM-CSF was met, and a trend toward improved overall survival in patients treated with vaccine was observed.
Whole Cell–Based Vaccines
A theoretical advantage of using whole tumor cells for vaccination is the broad spectrum of TAAs and mutated antigens potentially available for recognition and attack by immune cells. Vaccination with autologous tumors appears to be most suitable for this approach; a limitation is the difficulty of harvesting and preparing tumor tissue from individual patients. Irradiated melanoma cells harvested from metastatic lesions and engineered to secrete GM-CSF induced tumor necrosis and infiltration with T lymphocytes and plasma cells in metastatic sites of melanoma patients. Vaccination sites showed infiltration with T cells, DCs, and macrophages. In a follow-up study, patients with advanced melanoma previously vaccinated with irradiated autologous GM-CSF–secreting melanoma cells received ipilimumab after an interval of several years. Of note, metastatic melanoma lesions in 3 of 3 melanoma patients with previous vaccine exhibited extensive tumor necrosis with lymphocyte and granulocyte infiltrates, whereas no tumor necrosis was seen in 4 of 4 melanoma patients previously immunized with defined melanosomal antigens. A separate study, in which patients with metastatic melanoma were treated with ipilimumab 1 to 4 months after treatment with irradiated autologous GM-CSF–secreting melanoma cells, showed a linear relationship between the extent of tumor necrosis in posttreatment biopsies and the ratio of intratumoral CD8 + T cells and FoxP3 + Tregs.
M-Vax (AVAX Technologies, Philadelphia, PA) is an autologous whole-cell melanoma vaccine consisting of irradiated tumor cells treated with the hapten dinitrofluorobenzene (DNP). Six of 97 patients with advanced melanoma who received M-Vax after a low dose of cyclophosphamide in a phase 2 trial had a complete or partial response; 5 patients had a mixed tumor response. The median overall survival was 21.4 months in responders and 8.7 months in nonresponders ( P = .010). Based on these data, a phase 3 trial was initiated randomizing M-Vax versus placebo (2:1) given with cyclophosphamide, low-dose IL-2, and bacillus Calmette-Guérin (BCG).
Allogeneic whole-cell vaccines derived from tumor cell lines or individual tumors from other patients have the advantage that they can be produced for off-the-shelf use. A prominent example is the allogeneic GM-CSF–secreting prostate carcinoma cell vaccine termed GVAX (Cell Genesys, South San Francisco, CA), which showed encouraging tumor activity in phase 1/2 trials, but ultimately failed because of lack of clinical efficacy in 2 phase 3 trials. Similarly, an allogeneic whole tumor cell vaccine comprising 3 melanoma cell lines known as Canvaxin appeared to show an overall survival benefit in a nonrandomized phase 2 trial, in which 150 completely resected stage IV melanoma patients vaccinated with Canvaxin lived longer than 113 matched, nonvaccinated controls. Nevertheless, a prospective phase 3 trial randomizing Canvaxin plus BCG versus BCG alone, enrolling more than 1500 patients with fully resected stage III or stage IV melanoma, was stopped early because of a low likelihood of demonstrating an overall survival benefit in the vaccine arm.
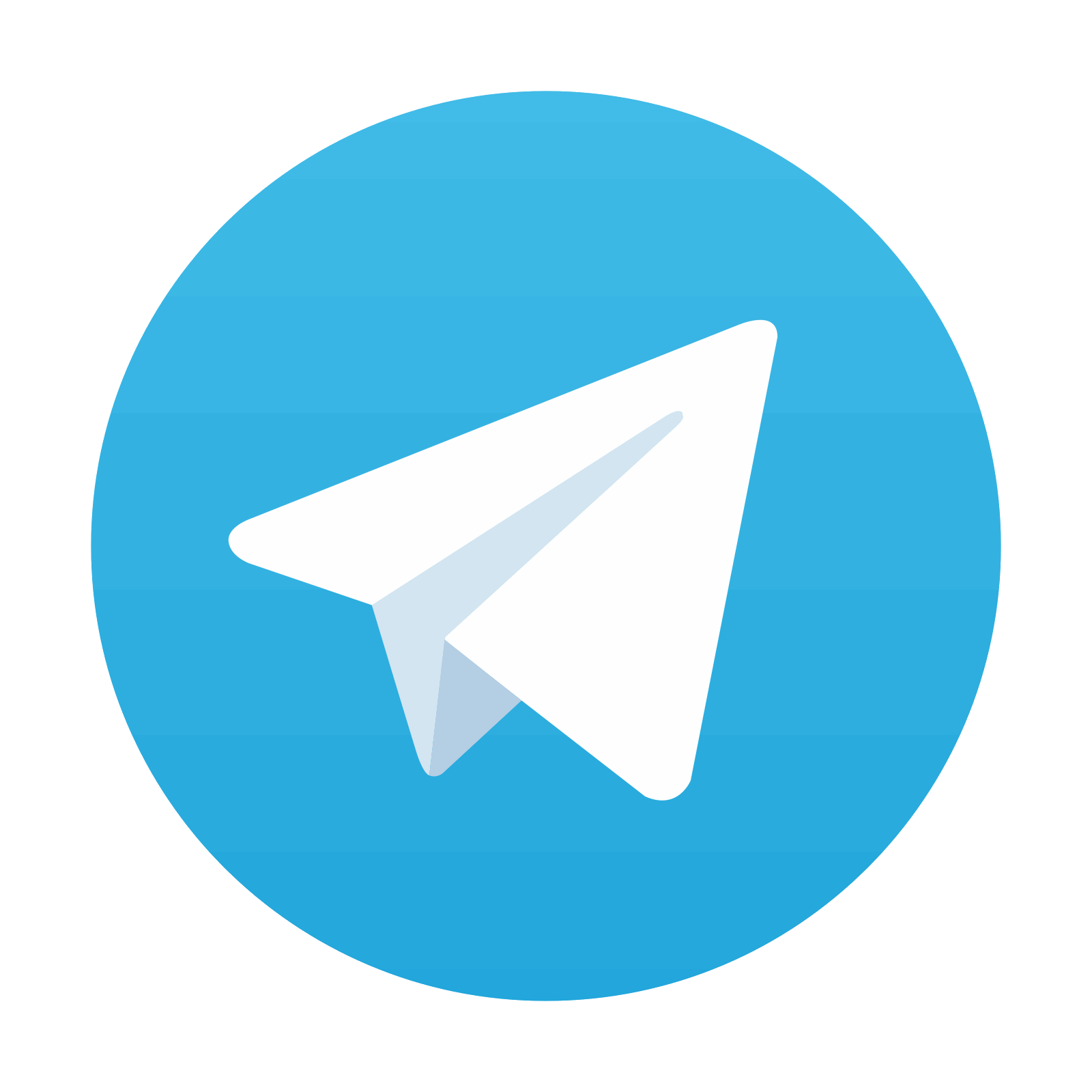
Stay updated, free articles. Join our Telegram channel

Full access? Get Clinical Tree
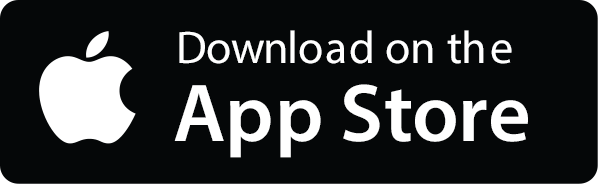
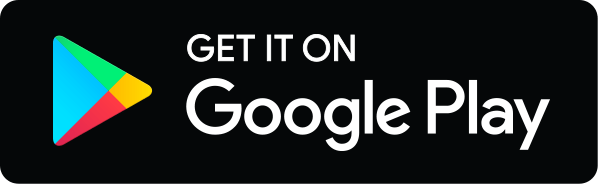