Chapter 18 Vaccination
• Vaccination applies immunological principles to human health. Adaptive immunity and the ability of lymphocytes to develop memory for a pathogen’s antigens underlie vaccination. Active immunization is known as vaccination.
• A wide range of antigen preparations are in use as vaccines, from whole organisms to simple peptides and polysaccharides. Living and non-living vaccines have important differences, living vaccines being generally more effective.
• Adjuvants enhance antibody production, and are usually required with non-living vaccines. They concentrate antigen at appropriate sites or induce cytokines.
• Most vaccines are still given by injection, but other routes are being investigated.
• Vaccine efficacy needs to be reviewed from time-to-time.
• Vaccine safety is an overriding consideration. When immunization frequencies fall, the population as a whole is not protected. Fears over the safety of the MMR vaccine resulted in measles epidemics and increases in incidence of rubella.
• Vaccines in general use have variable success rates. Some vaccines are reserved for special groups only and vaccines for parasites and some other infections are only experimental.
• Passive immunization can be life-saving. The direct administration of antibodies still has a role to play in certain circumstances, for example when tetanus toxin is already in the circulation.
• Non-specific immunotherapy can boost immune activity. Non-specific immunization, for example by cytokines, may be of use in selected conditions.
• Immunization against a variety of non-infectious conditions is being investigated.
• Recombinant DNA technology will be the basis for the next generation of vaccines. Most future vaccines will be recombinant subunit vaccines incorporated into viral or bacterial vectors. This should provide enhanced efficacy and safety.
Vaccination
Vaccines apply immunological principles to human health
• priming of specific lymphocytes to expand the pool of memory cells;
• use of harmless forms of immunogen – attenuated organisms, subcellular fragments, toxoids or vectors;
• use of adjuvants to enhance immune responses; and
• production of safe, affordable vaccines to promote herd immunity.
Vaccines can protect populations as well as individuals
• if the organism is highly infectious so that one individual can rapidly infect several non-immune individuals, as is the case for measles, a high proportion of the population must be immune to maintain herd immunity;
• if the infection is less readily transmitted, immunity in a lower proportion of the population may be sufficient to prevent disease transmission.
Antigen preparations used in vaccines
A wide variety of preparations are used as vaccines (Fig. 18.1). In general, the more antigens of the microbe retained in the vaccine, the better, and living organisms tend to be more effective than killed organisms. Exceptions to this rule are:
• diseases where a toxin is responsible for the pathology – in this case the vaccine can be based on the toxin alone;
• a vaccine in which microbial antigens are inserted into vector and expressed in a host cell.
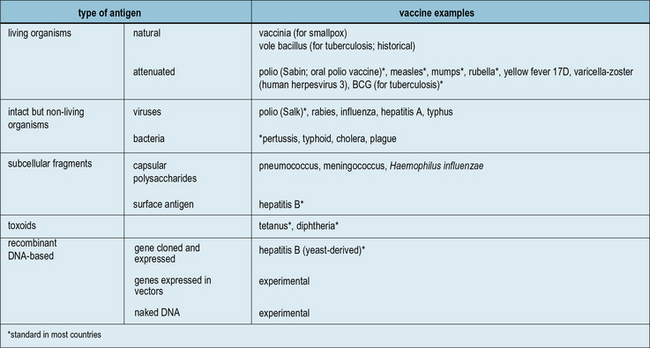
Fig. 18.1 The main antigenic preparations
A wide range of antigenic preparations are used as vaccines.
Live vaccines can be natural or attenuated organisms
Natural live vaccines have rarely been used
Apart from vaccinia, no other completely natural organism has ever come into standard use. However:
• bovine and simian rotaviruses have been tried in children;
• the vole tubercle bacillus was once popular against tuberculosis, and
• in the Middle East and Russia Leishmania infection from mild cases is reputed to induce immunity.
The new rotavirus vaccines should prevent many infants from dying in developing countries
Greater knowledge of the viral molecular biology and techniques manipulating the virus in vitro, have now led to the development of two new and highly effective vaccines against rotavirus infection. These vaccines are marketed under the names Rotarix™ and RotaTeq™. The former is a live attenuated virus produced by repeated passage in animal cell lines in the laboratory (see below). The latter is a complex of 5 different hybrid viruses providing immunity to the 5 most prevalent viral strains. These viruses are based on the bovine rotavirus which is naturally attenuated in human hosts. Into this backbone have been incorporated the human rotavirus viral capsid proteins VP4 or VP7, which are known to be the targets of natural immunity in human rotavirus infection (Fig. 18.w1).
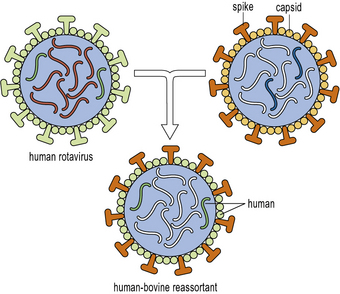
Fig. 18.w1 Reassortment to generate an oral live rotavirus vaccine
(Reprinted by permission from Macmillan Publishers Ltd: Nature Medicine (Buckland BC: The process development challenge for a new vaccine. Nature Medicine 2005;11:S16–S19.).
Attenuated live vaccines have been highly successful
The real successes were with viruses, starting with the 17D strain of yellow fever virus obtained by passage in mice and chicken embryos (1937), and followed by a roughly similar approach with polio, measles, mumps, and rubella (Fig. 18.2)
Just how successful the vaccines for polio, measles, mumps, and rubella are is shown by the decline in these four diseases between 1950 and 1980 (Fig. 18.3).
Attenuated microorganisms are less able to cause disease in their natural host
• type 1 polio has 57 mutations and has almost never reverted to wild type;
• types 2 and 3 vaccines depend for their safety and virulence on only two key mutations – frequent reversion to wild type has occurred, in some cases leading to outbreaks of paralytic poliomyelitis.
Killed vaccines are intact but non-living organisms
Killed vaccines are the successors of Pasteur’s killed vaccines mentioned above:
• some are very effective (rabies and the Salk polio vaccine);
• some are moderately effective (typhoid, cholera, and influenza);
• some are of debatable value (plague and typhus); and
• some are controversial on the grounds of toxicity (pertussis).
Figure 18.4 lists the main killed vaccines in use today. These are gradually being replaced by attenuated or subunit vaccines. However, in the case of polio, some countries are reverting to the use of killed vaccine which is safer than the attenuated vaccine, even though it is less effective. This choice only becomes relevant when the risk of contracting the disease is low in comparison with the risk of developing adverse reactions to the vaccine.
Inactivated toxins and toxoids are the most successful bacterial vaccines
The most successful of all bacterial vaccines – tetanus and diphtheria – are based on inactivated exotoxins (Fig. 18.5), and in principle the same approach can be used for several other infections. An inactive, mutant form of diphtheria toxin (CRM197) has been used as the basis for a number of newly generated conjugate vaccines (see below).
Subunit vaccines and carriers
Aside from the toxin-based vaccines, which are subunits of their respective microorganisms, a number of other vaccines are in use which employ antigens either purified from microorganisms or produced by recombinant DNA technology (Fig. 18.6). For example, a recombinant Hepatitis B surface antigen synthesized in baker’s yeast, has been in use since 1986.
Conjugate vaccines are effective at inducing antibodies to carbohydrate antigens
Starting with Haemophilus influenzae (Hib) in the early 1990s, conjugate vaccines for Neisseria meningitis strains A, C, Y and W-135 are also now in widespread usage. In the UK up until 1992 when the vaccine was introduced, Hib was the major cause of infantile meningitis leading to many hundreds of cases per year. The introduction of the vaccine led to a very rapid decline making Hib meningitis now a very rare occurrence (Fig. 18.7).