© Springer Nature Singapore Pte Ltd. 2017
Jia Wei and Baorui Liu (eds.)Personalized Management of Gastric Cancer10.1007/978-981-10-3978-2_1313. Use of Nanomedicine in the Diagnosis of Gastric Cancer
(1)
The Comprehensive Cancer Center of Drum-Tower Hospital, Medical School of Nanjing University & Clinical Cancer Institute of Nanjing University, Nanjing, 210008, China
Gastric cancer is one of most common cancers and is a leading global cause of cancer-related deaths [1]. This is particularly true in the eastern regions of China [2, 3]. Unfortunately, gastric cancer is difficult to cure, in large part because most patients are diagnosed at advanced stages of the disease. Apart from the need for early diagnosis, clinically important practices are also the diagnosis for tumor staging, planning for surgical resection, and determining prognosis. It should be noted that gastric cancer is considered as a more “localized tumor,” which is slightly different from the “systemic tumors” such as breast and lung cancers. With this in mind, locoregional metastasis is the most important negative prognostic factor of gastric cancer [4–6]. As a result, the diagnosis of gastric cancer includes (1) its early detection; (2) its systemic imaging; (3) its local imaging, especially that of regional lymphatic imaging; and (4) detection of its circulating tumor cells (CTC).
Thanks to its unique, nanoscale material composition, nanomedicine provides many benefits for the diagnosis and treatment of cancer. To this end, a number of nano-drugs have been clinically used, including liposomal doxorubicin (Doxil) [7–9], pegylated liposomal doxorubicin [10–12], liposomal daunorubicin (DaunoXome) [13–15], liposomal paclitaxel [16], liposomal cytarabine (DepoCyt) [17, 18], and albumin-bound paclitaxel (Abraxane) [19–21] ], etc. Collectively, such nano-drugs promote the efficacy and reduce side effects of the chemotherapeutics with which they are loaded. Ultimately, these benefits act to increase their effectiveness in gastric cancer treatment.
Nanomedicine also has a promising role in cancer diagnosis [22–25], with some having gotten as far as clinical trials [26]. However, nanodiagnostics are only currently used in a limited number of situations [23]. Beginning with tumor imaging contrast, nanotechnology has also been applied to lymphatic imaging, circulating tumor cell (CTC) detection, and early cancer diagnosis. Moreover, theranostic based on nanomedicine have also attracted increasing attention, due to their construction as multifunctional, nanosystems with integrated diagnostic and therapeutic capabilities in a single nanoparticle [27–29]. With this in mind, in the following section, we will focus on the interface between gastric cancer and nano-diagnosis.
13.1 Nanomedicine in the Early Diagnosis of Gastric Cancer
Apart from occult blood test of stools, endoscopy is the most important and effective diagnostic method for the early detection of gastric cancer. That being said, conventional white-light endoscopy offers only structural information of the gastrointestinal tract without any attendant biochemical data. To enhance the sensitivity of endoscopy, a non-contact, fiber optic-based Raman spectroscopy device was reported by Zavaleta et al. [30]. This device has the potential to provide real-time, multiplexed functional information during routine endoscopy and thus is ideally suited for the detection of functionalized, surface-enhanced Raman scattering (SERS) nanoparticles as molecular imaging contrast agents. As a result, certain cellular subgroups (e.g., cancer cells) are able to be identified. A good case of this is the work of Wang et al. [31, 32], who applied monoclonal antibodies against epidermal growth factor receptor (anti-EGFR mAb) and human epidermal growth factor receptor-2 (anti-HER-2 mAb) SERS NPs to the lumenal surface of the rat esophagus. Their results showed that EGFR- and HER-2-expressing tumor cells were not only precisely located, but that biomarker visualization and expression quantification were in agreement with both immunohistochemistry and flow cytometry data. Obviously, this technique is based on the idea that one or more molecules are highly and specifically expressed on the surface of cancer cells. For gastric cancer, such molecules include carcinoembryonic antigen (CEA), cancer-related antigen 19-9 (CA19-9), cancer-related antigen 72-4 (CA72-4), HER-2, and EGFR. Although none of the aforementioned markers are 100% sensitive and specific, the combination of routine optical endoscopy and SERS nanoparticles would still provide a more sensitive and specific means for early gastric cancer detection than is currently available.
The detection of gastric cancer-related biomarkers is also an important part of early diagnosis. Due to their specific properties, the development of nanostructured biosensors with high analytical performance has continuously increased [33, 34]. The use of nanoparticles may increase the sensitivity of a given biosensor and generate higher accuracies, speed, and precision [33]. Nanoparticles can be applied into optical-based nanosensors [35–38], fluorescence-based nanosensors [39], electrical-/electrochemical-based nanosensors [33, 40–43], and magnetism-based nanosensors [44–46], to name a few. Among those nanoparticles used in nanosensors, gold and magnetic nanoparticles-based biosensors as well as quantum dots are the predominant types [34, 35, 47–52]. These nanoparticles have been reported to be used in nanosensors designed to detect CEA [53–56], CA125 [35, 57–59], CA724 [60], and HER-2 [61]. As these nanoparticle-enhanced nanosensors are of greater sensitivity and accuracy, they may also be used to the exploration of new biomarkers for early gastric cancer detection [62].
Some other nanostructures have also been applied to gastric cancer-related biomarkers. For instance, Chen et al. [55] reported an immunosensor for CEA that was based on nanosilver-coated magnetic beads and gold-graphene nanolabels. This nano-biosensor could detect CEA at a concentration as low as 1.0 pg/mL. In another instance, Jokerst et al. [63] integrated semiconductor nanoparticle quantum dots (QDs) into a modular, microfluidic biosensor for the multiplexed quantification of CEA, CA125, and HER-2/Neu. They found that using QD probes in a miniaturized biosensor format leads to signal amplification that was 30 times that of standard molecular fluorophores. Moreover, they also saw a reduction in observed limits of detection by nearly two orders of magnitude relative to an enzyme-linked immunosorbent assay (ELISA).
13.2 Nanomedicine in the Systemic Imaging of Gastric Cancer
The methods used for whole body scanning include computed tomography (CT), magnetic resonance imaging (MRI), positron emission computed tomography (PET), single-photon emission computed tomography (SPECT), and PET-CT. For organ-specific examinations, a fast and low-cost method is ultrasound [23]. The current imaging and therapeutic agents suffer from nonspecific body distribution, not to mention rapid clearance, poor pharmacokinetics, and undesirable side effects [64–68]. Therefore, the development of cancer nanomedicine provides new pathways for enhanced cancer imaging using new types of nanomaterials. A variety of nanoparticles have recently emerged as promising strategies for cancer diagnosis owing to their nanoscale sizes, high agent loading capability, tailorable surface properties, controllable release patterns, and enhanced permeability and retention (EPR) [65, 67, 68]. Although successfully developing safe and effective nanoparticle-based imaging modalities for in vivo gastric cancer targeting imaging remains a large challenge, some studies have made progress in the right direction.
Superparamagnetic iron oxide (SPIO) MRI contrast agents are the first agents to have been clinically approved and include ferumoxides (Feridex in the USA, Endorem in Europe) and ferucarbotran (Resovist). However, both Feridex and Resovist are approved only for MRI of the liver. Nevertheless, magnetic nanoparticles are still the most frequently used in gastric cancer imaging.
Wang et al. [69] reported on SPIO nanoparticles (SPION) coated with SiO2 as core-shell nanoparticles. Nanoparticles were labeled with a near-infrared fluorescence (NIRF) dye in addition to an anti-CD146 monoclonal antibody [70] for magnetic resonance MR/NIRF imaging. The MKN45 xenograft tumor model was easily identifiable in as little time as 30 min postinjection.
BRCAA1 monoclonal antibodies that were conjugated to fluorescent, magnetic nanoparticles for in vivo, targeted gastric cancer imaging were also reported by Wang et al [71]. The nanoparticles could target in vivo gastric cancer tissues in the xenograft of mice and could then be used for gastric cancer imaging using either fluorescence imaging or magnetic resonance imaging.
Zhou et al. [72] reported using folic acid (FA)-conjugated silica-capped gold nanoclusters for gastric cancer imaging. This type of nanoprobe exhibited good biocompatibility and was able to actively target both FA(+) MGC-803 cells and in vivo gastric cancer tissue (5 mm in diameter) in a nude mice model. Importantly, these nanoclusters allowed for excellent red-emitting fluorescence and CT imaging.
Trastuzumab (Herceptin) is a humanized monoclonal antibody targeted to the extracellular domain of HER-2, a tyrosine kinase receptor. Trastuzumab was approved by the Food and Drug Administration (FDA) of the USA for the treatment of HER-2-overexpressing metastatic gastric cancer [73–75]. Several trastuzumab-conjugated, inorganic nanoparticles have been reported for purposes of imaging and diagnosis [73]. These include dextran iron oxide nanoparticles [76], mesoporous silica nanoparticles [77], PLGA nanoparticles [78], and liposome-coated fluorescent magnetic nanoparticles [79]. Although the aforementioned nanoparticles were used in breast cancer, these systems can also be applied to HER-2-overexpressing gastric cancers [73, 80, 81].
Nanomaterials were also used in the area of ultrasound. For instance, Fan et al. [82] reported on nanobubbles, which were used as an ultrasound contrast agent. The nanobubbles exhibited a superior contrast imaging effect over SonoVue® microbubbles in gastric cancer xenografts. Additional studies showed that the nanobubbles could pass through the gaps between the endothelial cells into the tumor vascular system in order to enter the tissue space. Taken together, these findings provide morphological evidence for the applications of nanoparticles in ultrasound imaging of tumors.
Moreover, nanomaterials have been used into nuclear imaging of gastric cancer. To this end, increased expression of cellular membrane bound glucose-regulated protein 78 (GRP78) is considered to be a gastric cancer biomarker. Cheng et al. synthesized glucose-regulated protein 78 binding peptide (GRP78BP) guide111 in labeled polymeric micelles [83]. In vivo studies in murine gastric xenograft revealed that the radioactive intensity measured in subjects treated with GRP78BP-guided111, labeled micelles was statistically higher than in animals receiving only labeled micelles. These results indicated that GRP78 is a useful probing target that can be used for nuclear imaging and gastric cancer diagnosis.
13.3 Nanomedicine in Local Gastric Cancer Imaging
Lymphatic metastasis is one of the most important in dependent prognostic factor for gastric cancer [84, 85]. Consequently, it is undoubtedly helpful for treatment planning to know pretreatment status of lymph nodes of gastric cancer patients. Furthermore, intraoperative diagnosis of lymph node metastasis in patients with gastric cancer is vital for the extent of lymph node dissection [86]. However, to date, no imaging modality has been shown to be capable of effectively detecting gastric cancer lymphatic metastasis [87].
Ferumoxtran-10 (Combidex) is an MRI lymphotropic contrast agent for the detection of metastatic lymph nodes in various types of cancers. For instance, Tatsumi et al. [88] investigated the efficacy of ferumoxtran-10-enhanced MRI for the detection of metastases to lymph nodes in gastric cancer. The parameters for predictive accuracy were far superior to those evaluated by either CT or ultrasound alone. Nodes in the retroperitoneal and paraaortic regions were more readily identified on the MR images than those in the perigastric region. Therefore, the use of this modality shows promise in treatment planning and decision-making for gastric cancer patients.
Recently, Qiao et al. [87] reported on a new kind of molecular imaging probe that was based on upconversion nanoparticles with highly sensitive detection of lymphatic metastasis in gastric cancer. The core-shell, structured upconversion nanoparticles were coated with polyethylene glycol (PEG). In vivo studies in an orthotopic mouse model of human gastric cancer were then conducted, with the primary tumor and adjacent lymphatic metastatic sites being clearly differentiated. Moreover, lymphatic metastases that were smaller than 1 mm were successfully detected. These results show the promise that this nanoparticle has a highly effective approach to gastric cancer diagnosis.
Using photosensitive nanoparticles loaded with the indocyanine green (ICG) derivative ICG-loaded lactosome (ICGm), Tsujimoto et al. [86] detected metastatic lymph nodes in the ICGm-, but not ICG-, treated mice. PDT using ICGm was able to induce apoptosis and inhibited the growth of metastatic lymph nodes significantly. These results indicate that ICGm is a promising theranostic modality for lymph node metastasis in gastric cancer.
Image-guided surgery (IGS) has the potential to substantially impact patient treatment. Clinical trials of IGS using the FDA-approved fluorophores indocyanine green (ICG) and methylene blue have already exhibited preliminary successes. Moreover, incorporation of fluorescent nanoparticles will likely improve detection by providing a higher signal-to-noise ratio and reducing false-positive rates by means of active targeting [89]. Several types of fluorescent nanoparticles have been reported, such as liposome-embedded ICG [90, 91], SPIO-phospholipid-PEG-ICG [92], and hyaluronic acid (HLA)-derived nanoparticles loading ICG [93].
13.4 Using Nanomedicine to Detect Circulating Tumor Cells (CTCs) in Gastric Cancer
Circulating tumor cells (CTCs) are cancer cells that break away from either a primary tumor or a metastatic site and circulate in the peripheral blood. Crucially, they are supposed to serve as the cellular origin of metastasis [94]. Blood CTCs have been widely studied as a potential biomarker for diagnosis, prognosis, and molecular testing of metastatic gastric cancer [95, 96]. It is also vital for the real-time diagnosis, treatment planning, and evaluation of patients, leading to its classification as a “liquid biopsy.” However, due to their rarity and heterogeneity, it remains challenging to develop a CTC detection method with clinically significant sensitivity and specificity. This difficulty remains even with the commercialization of some devices such as CellSearch [97, 98] (it should be noted that CellSearch has not been approved for use in gastric cancer). With the recent advances in nanotechnology, a series of new and promising nanomaterials have been reported to enhance CTC detection [95, 99]. These include microfluidic chips [99, 100], nanoroughened structures [99], NanoVelcro chips [94], nanofibers [101], as well as nanoparticles [95, 102].
Microfluidics integrates physical, chemical, and biological technologies at the micro- and nanoscale levels, thus providing a miniaturized and portable tool for efficient CTCs separation [103]. In the latest decade, a great number of microfluidic chips have been shown to be capable of separating CTCs of prostate [104], breast [105–107], esophageal [107], and lung cancers [108] in addition to melanoma [109].
To this end, Galletti et al. [106] reported a novel HER-2 (human epidermal receptor-2)-based microfluidic device for the isolation of CTCs from the peripheral blood of patients with HER-2-expressing gastric cancers. They applied the HER-2 microfluidic device into the detection of CTCs from blood of metastatic gastric cancer patients and found that circulating tumor cells were detected not only in HER-2 high-expressing cancer patients but also in HER-2 low-expressing patients. As HER-2 is expressed in 67% of gastric cancer patients and overexpressed in 13–30% of the cases [106, 110, 111], the microfluidic device based on HER-2 is quite promising for the detection of CTCs in gastric cancer patients.
Based on a negative enrichment technique, Hyun et al. [105] reported a geometrically activated surface interaction (GASI) chip with an asymmetric herringbone structure designed to generate enhanced mixing flows. CD45 antibodies were immobilized inside the channel to capture leukocytes and release CTCs to the outlet. Blood samples from four patients with gastric cancer were then analyzed. CTCs were detected from all four samples, and the number of isolated CTCs varied from 1 to 5 in 1 mL of blood.
The materials used in nanoparticles designed for the detection of CTC have included gold [112], magnetic [113, 114], quantum dots [115], graphenes/graphene oxides [116], dendrimers, and stimuli-responsive polymers [117, 118]. That being said, previous studies focused on CTC detection and isolation using nanoparticles have mostly concentrated on breast, prostate, lung, and colon cancers. Reports on the detection of gastric CTCs using nanoparticles are comparatively little. For instance, He et al. [119] reported CTC isolation from gastric cancer patients’ peripheral blood samples with a biocompatible nano-film composed of TiO2 nanoparticles. Furthermore, 50% of the captured cells could be detached from the substrate and were expected to be of future clinical use.
It is also important to note that some nanoparticles have emerged that are able to detect special gastric cancer or gastric cancer stem cell markers, such as CD [102, 120], HER-2 [79], CD44 [121], and CD146 [69, 70]. These nanoparticles are expected to be promising candidates for gastric CTC detection.
Conclusions
Due to the high morbidity and mortality of gastric cancer, the exploration of more effective modalities for its diagnosis and treatment is critically important. Nanomedicine has shown great potential for increasing the sensitivity and specificity with regard to gastric cancer—not just with early detection. It has also shown promise with systemic and local imaging for the evaluation and treatment determination of gastric cancer, image-guided surgery, and the detection and isolation of CTCs. However, there are also several limitations that must be considered. First, most of the studies currently in the literature are either preclinical or in vitro. As a result, the safety and clinical applicability of most nanomaterials remain unclear. Second, most studies for gastric cancer diagnosis have been based on specific markers or ligands expressed by gastric cancer. However, the specificity of these molecules is usually limited, which restricts nanoparticle applications. Finally, a large proportion of the studies were derived from similar studies about breast, lung, and colorectal cancers. Studies based solely and specifically on the clinical characteristics of gastric cancer are comparatively rare. Therefore, to further promote the development of nanomedicine in the diagnosis of gastric cancer, more in-depth studies and increased interdisciplinary collaboration and information exchange between scientists will be needed [23].
References
1.
Ferlay J, Soerjomataram I, Dikshit R, Eser S, Mathers C, Rebelo M, et al. Cancer incidence and mortality worldwide: sources, methods and major patterns in GLOBOCAN 2012. Int J Cancer. 2015;136(5):E359–86.PubMed
2.
Yang L. Incidence and mortality of gastric cancer in China. World J Gastroenterol. 2006;12(1):17–20.PubMedPubMedCentral
3.
Zhou M, Wang H, Zhu J, Chen W, Wang L, Liu S, et al. Cause-specific mortality for 240 causes in China during 1990–2013: a systematic subnational analysis for the Global Burden of Disease Study 2013. Lancet. 2016;387(10015):251–72.PubMed
4.
Imano M, Yasuda A, Itoh T, Satou T, Peng YF, Kato H, et al. Phase II study of single intraperitoneal chemotherapy followed by systemic chemotherapy for gastric cancer with peritoneal metastasis. J Gastrointest Surg. 2012;16(12):2190–6.PubMed
5.
Ishigami H, Kitayama J, Kaisaki S, Hidemura A, Kato M, Otani K, et al. Phase II study of weekly intravenous and intraperitoneal paclitaxel combined with S-1 for advanced gastric cancer with peritoneal metastasis. Ann Oncol. 2010;21(1):67–70.PubMed
6.
Ishigami H, Kitayama J, Kaisaki S, Yamaguchi H, Yamashita H, Emoto S, et al. Phase I study of biweekly intravenous paclitaxel plus intraperitoneal cisplatin and paclitaxel for gastric cancer with peritoneal metastasis. Oncology. 2010;79(3-4):269–72.PubMed
7.
Yamaguchi N, Fujii T, Aoi S, Kozuch PS, Hortobagyi GN, Blum RH. Comparison of cardiac events associated with liposomal doxorubicin, epirubicin and doxorubicin in breast cancer: a Bayesian network meta-analysis. Eur J Cancer. 2015;51(16):2314–20.PubMed
8.
Khan DR, Webb MN, Cadotte TH, Gavette MN. Use of targeted liposome-based chemotherapeutics to treat breast cancer. Breast Cancer (Auckl). 2015;9(Suppl 2):1–5.
9.
Rivankar S. An overview of doxorubicin formulations in cancer therapy. J Cancer Res Ther. 2014;10(4):853–8.PubMed
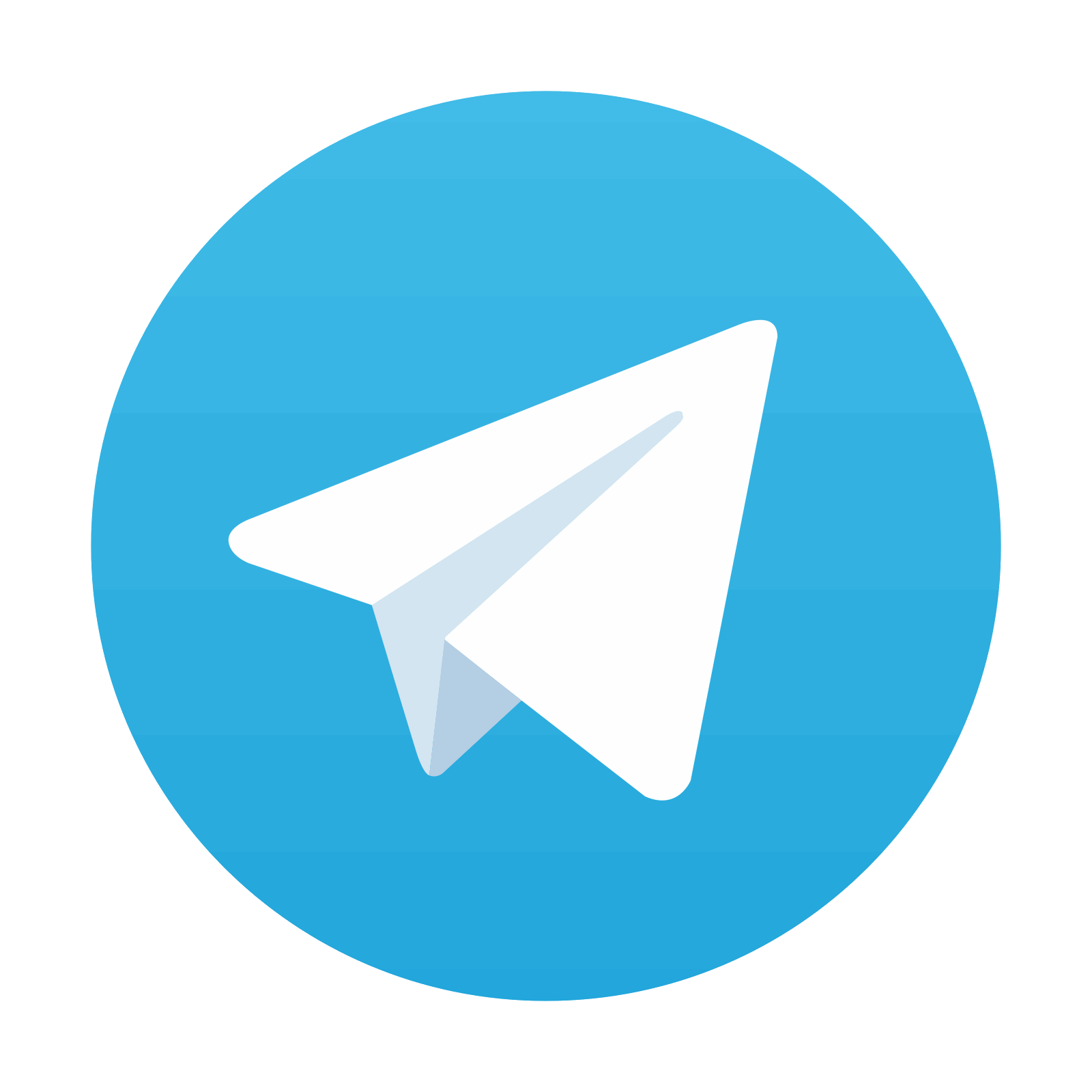
Stay updated, free articles. Join our Telegram channel

Full access? Get Clinical Tree
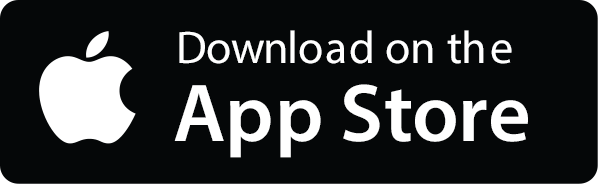
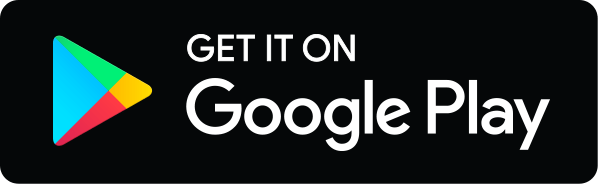
