9 Kurt Zatloukal,1 Pieter van’t Veer,2 Christian Viertler,1 Marc-Jeroen Bogaardt3 and Peter Hollman2 1 Institute of Pathology, Medical University of Graz 2 Division of Human Nutrition, Wageningen University 3 DLO Foundation, Agricultural Economics Research Institute, Wageningen University and Research Centre Biobanks for medical research are collections of essentially any type of human biological sample, such as blood, tissues (e.g. liver biopsies, visceral and subcutaneous adipose tissue, intestinal biopsies, tumours), urine or saliva, and data on the sample characteristics as well as on the health/disease status of the sample donor (Box 9.1). On the one hand, biobanks have been established in the context of prospective population cohort studies comprising hundreds of thousands of samples and data from a certain population that is followed up on disease incidence and outcome for many years to decades. On the other hand, biobanks emerged from collections established in the context of health care (e.g. collection of serum or tissue samples from patients treated at hospitals; Figure 9.1). Figure 9.1 Biobank containing a large collection of human formalin-fixed paraffin-embedded tissues representing a broad spectrum of human diseases that were established in the context of health care (BioBank Graz). Historically, valuable collections of biological samples from patients have been established by individual scientists to support their research work. However, there are several differences between the personal collection of an individual scientist and modern biobanks. These differences include the implementation of a governance structure for the biobank, defining responsibilities, access conditions and ethical and legal compliance. Furthermore, quality management is essential to guarantee proper sample quality. In general, individual scientists are not in a position to provide such a professional environment for their collections, and this led to the establishment of institutional biobanks or biobanks as common research infrastructures for whole universities or companies (Asslaber and Zatloukal 2007; Harris et al. 2012). Personalised medicine with its smaller and smaller disease subentities created a demand for increased collaboration among biobanks nationally and across borders, because single biobanks could not provide sufficient numbers of samples for a given disease subentity to achieve statistical significance in studies of single biobanks. Furthermore, increasing interest in understanding the impact of the genetic make-up of populations with different ethnic origins, as well as the increasing importance of environmental and lifestyle factors, made international collaboration mandatory. This development has gone hand in hand with the requirement for international quality standards and harmonised procedures to improve the interoperability of biobanks (Box 9.2). In Europe, in the roadmap of the European Strategy Forum for Research Infrastructures (ESFRI) member states have prioritised a pan-European research infrastructure for Biobanking and Biomolecular Resources (BBMRI; Yuille et al. 2008). BBMRI was established by the European member states and the European Commission under the recently created legal entity the European Research Infrastructure Consortium (ERIC) in 2013. BBMRI-ERIC is the only ESFRI research infrastructure for biobanking for the whole of Europe that will provide access to quality-defined human biological samples and associated information following internationally harmonised standards and procedures. BBMRI-ERIC offers a common framework and a single access point to foster high-level scientific collaboration that requires access to various types of human biological samples, ranging from blood or deoxyribonucleic acid (DNA) samples from large cohort studies, tissues from cancer biobanks, serum, plasma or urine collections to samples containing pathogens or microbiomes. Furthermore, the scope of BBMRI-ERIC comprises biological resources, such as cell lines, gene clone collections, protein libraries or antibodies. All samples are linked to comprehensive information on the health or disease condition of the sample donor and related to sample quality. A minimal data set that has to be linked to each sample has been defined (Brochhausen et al. 2013). The data management infrastructure of BBMRI-ERIC is designed to handle not only data related to sample origin, but also the massive data generated by sample analyses using modern -omics technologies. A specific challenge in this context is also to meet ethical and legal requirements, particularly to guarantee the privacy of sample donors. It is noteworthy that BBMRI-ERIC is a research infrastructure owned by the European member states and should be in operation for several decades, thereby providing sustainable access to a key resource for the advancement of human health, medicine and related industries. Biobanks provide a broad spectrum of solutions that are also relevant for nutrition research. For example, several EU or nationally funded research programmes have put major effort into developing proper data-management concepts, including solutions for data protection and privacy. Furthermore, useful expertise has been developed in the effective consideration of different ethical and legal systems in multinational study designs (reports are available at www.bbmri-eric.eu). Several biobanks have linked their sample-associated data with health, disease and death registries, which has resulted in a marked enrichment of biobanks. This is of particular importance in establishing the relationship between molecular alterations identified in samples and health or disease outcomes. Expertise in sample management is mandatory for each biobank. Proper collection, processing, aliquotting, stabilisation and storage of samples are prerequisites to ensure that analytical data are reliable and reflect the status of biological processes within the body (Figure 9.2). The rapid advancement of analytical technologies requires a continuous co-development of quality standards for biological samples in order to deliver the full analytical capacities, because even the most advanced technologies cannot produce better results than the quality of the samples analysed. Figure 9.2 Biobanking of cryopreserved tissues. (a) Snap freezing of a tissue sample in metylbutane pre-cooled by liquid nitrogen. (b) Different types of storage containers and labelling (handwritten, barcode, radiofrequency identification). (c) Liquid nitrogen storage system with cooled retrieval unit to prevent temperature shifts of samples (BioBank Graz). Furthermore, biobanking has extended its scope from blood and tissue to a variety of other sample types, such as urine and saliva as well as the intestinal microbiome, allowing a comprehensive investigation of individuals’ biological response to environmental and lifestyle factors. Each of these sample types requires specifically optimised standard operating procedures for handling and specific quality controls, resulting in a comprehensive body of knowledge that has to be established in the context of the operation of biobanks. This knowledge has often been generated in parallel in various biobanks, resulting in unnecessary duplication of effort or, even worse, in different procedures that are not compatible between biobanks and thus impede collaboration. Therefore, a major emphasis is currently being placed on overcoming the fragmentation of the biobanking landscape by improving interoperability and coordinating developments (Harris et al. 2012). A substantial amount of evidence on diet–health relationships is based on population cohort studies assessing dietary intake and subsequent health outcomes. However, these observational studies are hampered by unavoidable errors in dietary assessment methods. Randomised controlled trials circumvent this problem, but their duration is usually limited and risk factors or intermediate endpoints are used instead of ‘hard’ clinical endpoints. As indicators of dietary intake, biomarkers can fulfil a role in the validation of dietary assessment or as a proxy for dietary intake. As effect markers they are relevant as intermediate or proxy endpoints for the disease outcome. Thus, biomarkers can be seen as signals of subsequent biological events between the consumption of foods, the impact on metabolism and health, and the eventual occurrence of disease (Box 9.3). In this way, they are crucial in substantiating the biological and pathophysiological models of nutrition-related diseases. In the context of environmental protection, Hulka et al. (1990) provide a framework for biomarkers that distinguishes biomarkers of internal dose, of the biologically effective dose, early response, and altered structure and function, with all the relationships between these being modified by inherited or acquired susceptibility. In nutrition research the framework translates to familiar concepts, for instance for vitamin B12 we distinguish biochemical indicators of short-term intake (B12 concentration in plasma) or long-term intake (B12 concentrations in erythrocytes), biomarkers of nutrient status (liver stores quantified by B12 concentration), early indicators of altered metabolic responses or deficiency (methylmalonic acid, MMA) and clinical alterations (megaloblastic anaemia) causing tiredness and weakness. Susceptibility is increased when there is insufficient hydrochloric acid to separate B12 from food proteins, or because of intrinsic factor insufficiency prohibiting the absorption of the intrinsic factor–B12 complex (pernicious anaemia), or impaired absorption resulting from coeliac disease or Crohn’s disease. Biomarkers can be used for numerous objectives and in various research settings. For the validation of dietary assessment, there is a distinction between recovery markers and concentration markers. Examples of recovery markers are urinary nitrogen excretion for validation of protein intake assessment, and urinary sodium and potassium; the doubly labelled water technique can be used for validation of energy expenditure. Recovery markers are based on the principle of nutrient balance and are expressed in amount per day. They are measured in urine; in the ideal situation, 24-hour urine should be collected. If this is not available spot urine can be used, but should be corrected for creatinine excretion. If the objective is to validate assessment methods for nutrition surveillance, the estimated intake is compared to excretion and the conclusion is on the average amount (or percentage) of over- or underreporting. If the objective is to estimate the strength of association between dietary intake and health outcomes in, for example, a cohort study, the degree of attenuation of the observed association can be estimated by regression of the recovery marker on the assessed intake (calibration factor).
Use of Biobanks in Nutrition Research
9.1 Introduction
9.2 Biomarkers in nutrition research
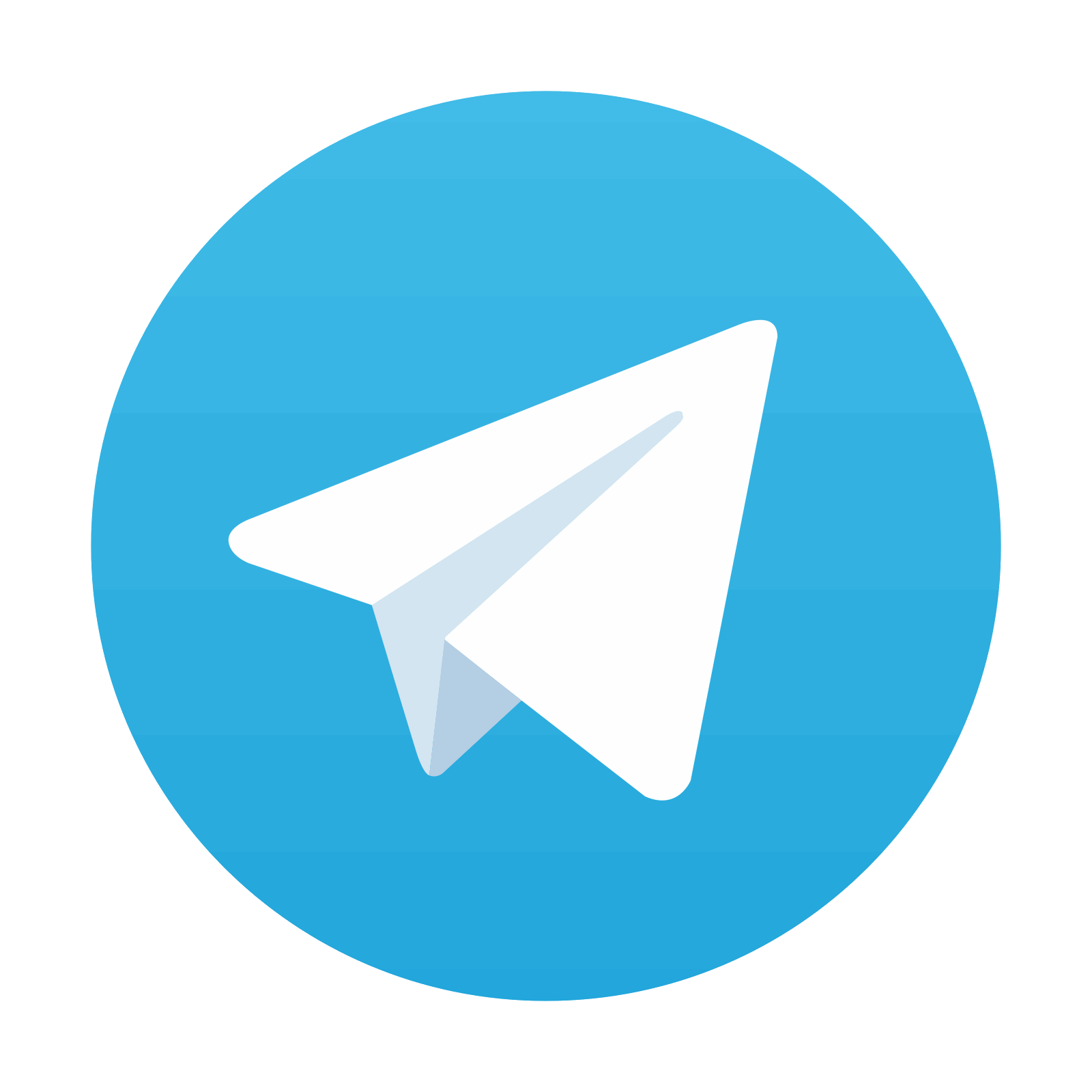
Stay updated, free articles. Join our Telegram channel

Full access? Get Clinical Tree
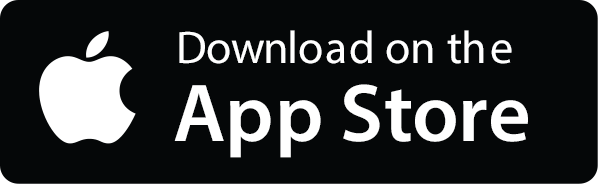
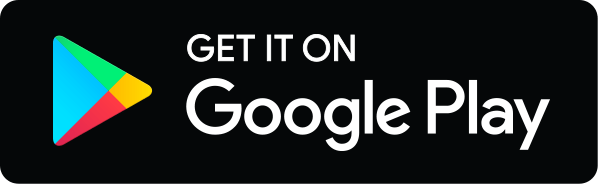