Fig. 10.1
Schematic model showing the overall domain structure and posttranslational modifications of PTEN. PTEN is a 403 amino acid protein that contains five domains. On the N-terminal side is a PtdIns (4,5) P2-binding domain that can be monoubiquitinated. This is followed by the catalytic domain that contains the signature CX5RT PTP phosphatase motif that is the active site. Following the catalytic domain is a topology II C2 domain that interacts with negatively charged phospholipids. On the C-terminus is a Pro-, Glu-, Ser-, Thr-rich domain (PEST) and a PDZ-binding motif
The active site of PTEN contains a reactive cysteine that is required for catalysis (HC 124 KAGKGRT) [50]. The pKa of Cys124 is 4.7–5.4 and is deprotonated at physiological pH making it a strong nucleophile. This is due to the presence of Lys residues (Lys125, 128) within the active site pocket. This makes Cys124 susceptible to oxidation by hydrogen peroxide via the formation of a disulfide with Cys71 resulting in inhibition of enzymatic activity [46]. These cysteine disulfides may be subsequently reduced via the thioredoxin system and the peroxiredoxin system [46, 51, 52]. In addition Cys124 is thought to be also susceptible to glutathionylation and carbonylation [42, 49, 53].
Another source of electrophiles in cells is reactive aldehydes that are formed from lipid peroxidation [54, 55]. In alcoholism, a focus of research has been the identification of the effects of lipid peroxidation in chronic alcoholics as well as in murine models [56]. The lipid aldehyde, 4-hydroxynonenal (4-HNE) irreversibly modifies PTEN both in its active site as well as within its C2 domain [42]. Studies have shown that inhibition of PTEN activity by direct 4-HNE modification occurs in numerous cell types (HepG2, MCF7, HEK-293) as well as in a murine chronic ethanol model [42, 53, 57, 58]. One deficit of these studies was that the site of lipid aldehyde modification of PTEN was not directly identified in vivo. Current technology was not sufficient to reliably identify the site of PTEN carbonylation from livers isolated from ethanol fed mice.
PTEN is reversibly modified by the electrophile S-nitrosoglutathione in pulmonary epithelial cells. This modification decreased PTEN activity, increased AKT activity, and increased HIF1α protein accumulation [59]. In the liver, using a NASH model, a high-fat diet resulted in increased glutathionylation of PTEN and decreased activity [44]. We have demonstrated increased glutathionylation occurs following chronic ethanol consumption. Utilizing immunohistochemistry, pericentral increases in protein-SSG were identified in mice chronically fed with ethanol for 6-weeks [60]. Based on these data, it is not unreasonable to predict increased glutathionylation of PTEN in alcoholism.
PTEN negatively regulated by acetylation. Acetylation of Lys125, Lys128 by the histone acetyltransferase PCAF results in decreased PTEN activity and increased Akt activation following growth factor stimulation [61]. Acetylation of Lys402 in the PTEN C-terminal PDZ-binding domain by CREB-binding protein results in enhanced binding to PDZ containing proteins [62]. Acetylation is significantly altered by ethanol via regulation of the deacetylases Sirtuin 1 and Sirtuin 3 [63–66]. Following chronic ethanol consumption, acetylation of cytosolic proteins as well as mitochondrial proteins is increased and Sirtuin 1/3 activities are decreased [38, 63, 67]. Based on these data, acetylation of PTEN may be altered during chronic ethanol consumption.
PTEN has been demonstrated to localize to the nucleus. Nuclear localization is increased following both monoubiquitination of Lys13 and Lys289 as well as under conditions of increased oxidative stress. Once in the nucleus, PTEN reduces p53-dependent tumor progression [68]. In a murine high-fat diet model of NASH, PTEN monoubiquitination was not increased [44]. In rat ethanol models, PTEN has been demonstrated to be downstream of p53 and increased p53 nuclear localization correlated with increased PTEN expression [36]. The authors concluded that these factors contributed to insulin resistance. Monoubiquitination of PTEN was not examined in this system.
Phosphorylation plays an integral role in regulating PTEN activity. Although PTEN has been demonstrated to be phosphorylated on numerous residues within its c-terminal tail as well as its C2 domain, of particular importance to this review is phosphorylation on Ser380, Thr382, and Thr383. Substituting these residues for alanine results in increased membrane association as well as PtdIns 3-phosphatase activity demonstrating an inhibitory role for these residues [69].
10.5 Conclusions
In summary as presented in Fig. 10.2, from the presented data it is clear that the role that PTEN plays in ALD as well as ALD-induced HCC has not been fully elucidated. What is known is that there appears to be concentration-dependent ETOH effects on PTEN inhibition/Akt activation. Given the preponderance of data is in rat models, additional experiments are necessary in alternative rodent models to validate findings in the rat. It is also not known if current data translates into humans. In other models, PTEN has been demonstrated to localize to not only the cytosol but also to the mitochondria and the nucleus. What are the effects of chronic ethanol consumption on hepatic PTEN subcellular localization? Does monoubiquitination play a role? Given the fact that ethanol induces a phase III response in the liver, are there hepatozonal-specific effects on PTEN signaling as well? Given changes in PtdIns 3 kinase signaling over time during ethanol consumption, more studies need to be performed using a spaciotemporal model to fully elucidate the contribution of the PTEN/Akt pathway to ethanol-induced steatosis and hepatocellular damage.
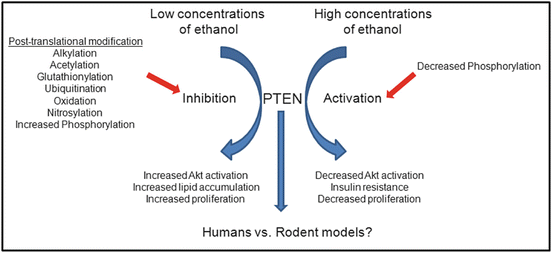
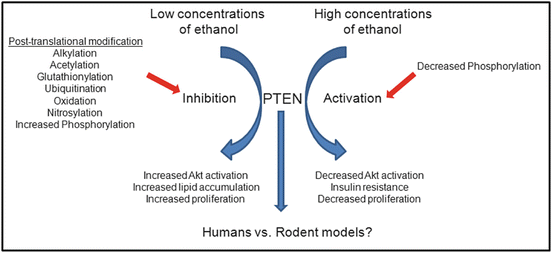
Fig. 10.2
Schematic summary of the effects of ethanol on PTEN and future directions. PTEN is clearly an important regulator of hepatic steatosis as well as insulin resistance. In chronic alcoholism, the effects of ethanol have not been fully elucidated. It is apparent that different concentrations of ethanol induce differential responses in PTEN activation. At low ETOH concentrations, PTEN is inhibited resulting in increased Akt signaling and increased lipid accumulation. At high ETOH concentrations, PTEN is activated contributing to decreased Akt signaling and insulin resistance. Future research will need to be focused on elucidating the ability of ethanol to affect PTEN spatiotemporally in the liver and to determine ethanol-dependent changes in posttranslational modification of PTEN
As mentioned, we have demonstrated an increase in overall protein glutathionylation in our chronic ethanol models [60]. An unexplored question is whether PTEN is glutathionylated following chronic ethanol consumption and what are the ramifications? In addition, does the acetylation status of PTEN change following ethanol consumption? Posttranslational modifications of PTEN have not been examined in patients with HCC. Furthermore, if PTEN is downregulated following ethanol consumption at low concentrations of ethanol, mild or moderate consumption may promote HCC. In alcoholics who consume high amounts of alcohol, via its ability to downregulate Akt, PTEN activation may actually have a “beneficial” inhibitory effect towards development of HCC.
Grants and Funding
This research was funded by the following grants from the National Institutes of Health; 5F32AA018613-03 CTS.
References
1.
2.
3.
Beier JI, McClain CJ (2010) Mechanisms and cell signaling in alcoholic liver disease. Biol Chem 391(11):1249–1264PubMedCentralPubMedCrossRef
4.
Kochanek KD, Murphy SL, Anderson RN, Scott C (2004) Deaths: final data for 2002. Natl Vital Stat Rep 53(5):1–115PubMed
5.
6.
7.
Koike K (2013) The oncogenic role of hepatitis C virus. Recent Results Cancer Res 193:97–111CrossRef
8.
Mathurin P (2012) EASL clinical practical guidelines: management of alcoholic liver disease. J Hepatol 57(2):399–420CrossRef
9.
Liew CT (1990) The clinicopathological spectrum of alcoholic liver disease—an autopsy survey of 441 cases. Changgeng Yi Xue Za Zhi 13(2):72–85PubMed
10.
Davis GL, Dempster J, Meler JD, Orr DW, Walberg MW, Brown B, Berger BD, O’Connor JK, Goldstein RM (2008) Hepatocellular carcinoma: management of an increasingly common problem. Proc (Bayl Univ Med Cent) 21(3):266–280
11.
12.
13.
Sze KM, Wong KL, Chu GK, Lee JM, Yau TO, Ng IO (2011) Loss of phosphatase and tensin homolog enhances cell invasion and migration through AKT/Sp-1 transcription factor/matrix metalloproteinase 2 activation in hepatocellular carcinoma and has clinicopathologic significance. Hepatology 53(5):1558–1569PubMedCrossRef
14.
15.
16.
Wu SK, Wang BJ, Yang Y, Feng XH, Zhao XP, Yang DL (2007) Expression of PTEN, PPM1A and P-Smad2 in hepatocellular carcinomas and adjacent liver tissues. World J Gastroenterol 13(34):4554–4559PubMed
17.
Boyault S, Rickman DS, de Reynies A, Balabaud C, Rebouissou S, Jeannot E, Herault A, Saric J, Belghiti J, Franco D, Bioulac-Sage P, Laurent-Puig P, Zucman-Rossi J (2007) Transcriptome classification of HCC is related to gene alterations and to new therapeutic targets. Hepatology 45(1):42–52PubMedCrossRef
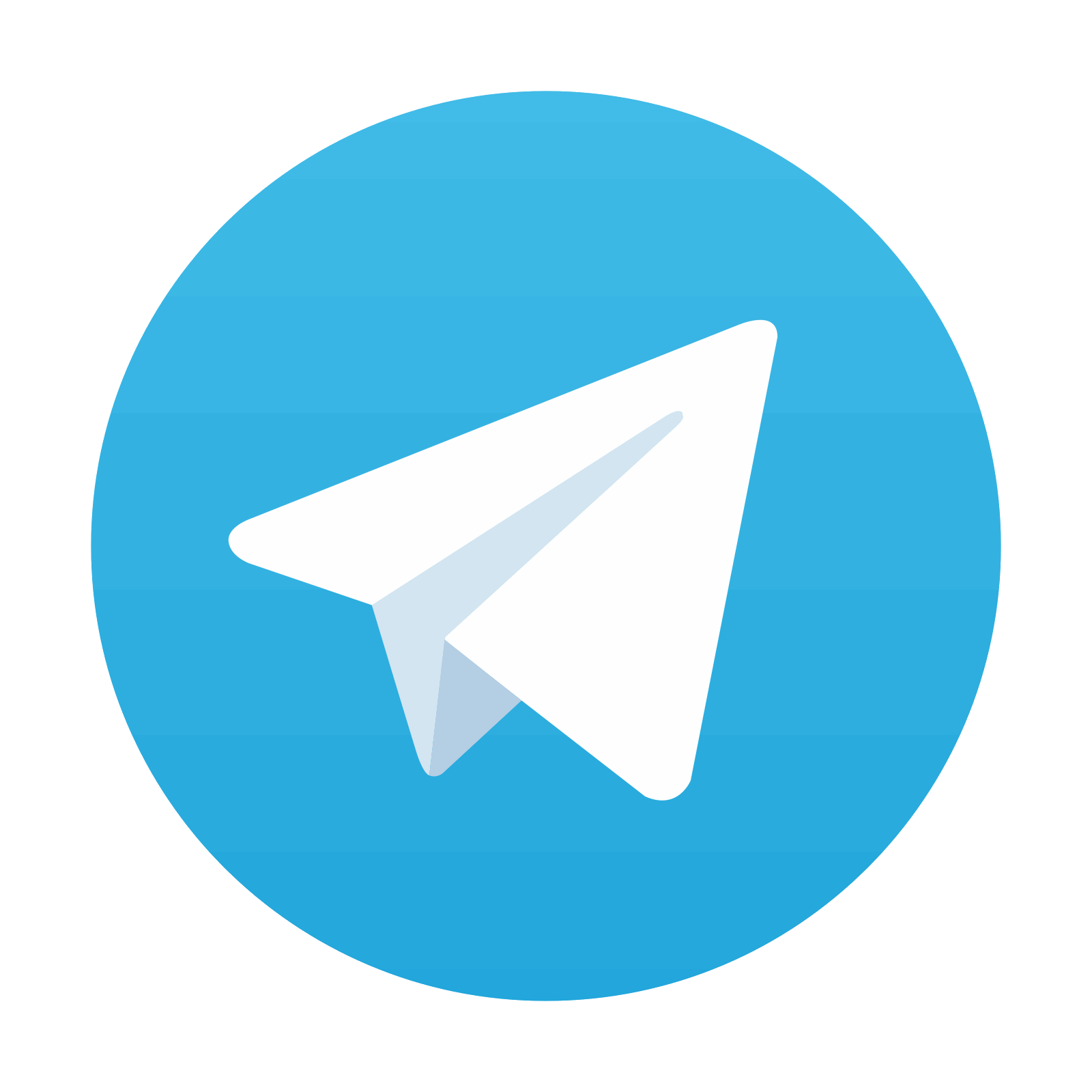
Stay updated, free articles. Join our Telegram channel

Full access? Get Clinical Tree
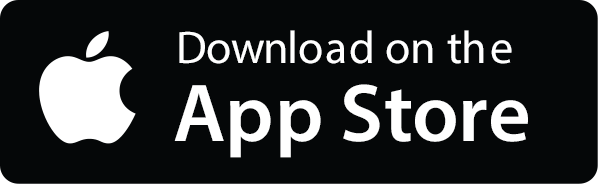
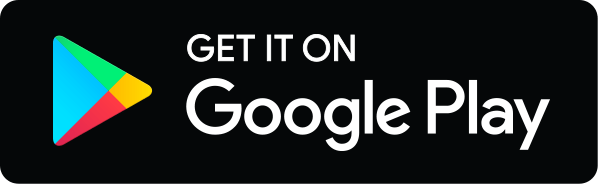