Chronic lymphocytic leukemia (CLL) is the most common leukemia in adults. Although significant advances have been made in the treatment of CLL in the last decade, it remains incurable. Treatments may be too toxic for some elderly patients, who constitute most of the individuals with this disease, and there remain subgroups of patients for which this therapy has minimal activity. This article summarizes the current understanding of the immune defects in CLL. It also examines the potential clinical implications of these findings.
Key points
- •
Chronic lymphocytic leukemia (CLL) is the commonest type of leukemia in adults.
- •
CLL is associated with a profound immunodeficiency highlighted by the presence of recurrent infections and failure of antitumor immune responses.
- •
This immunodeficiency is caused by a combination of impaired T-cell and natural killer cell function, and increased numbers of regulatory T cells and nurselike cells.
- •
Many of the standard treatments for CLL have significant effects on the immune system.
- •
Immunomodulatory approaches such as lenalidomide, tumor vaccines, and chimeric antigen receptor–modified T cells are showing great promise for the future of CLL treatment.
Introduction
Chronic lymphocytic leukemia (CLL) is the most common leukemia in adults, with a lifetime risk of 0.49%. It is characterized by the clonal expansion of CD5+IgD+/IgM+ B lymphocytes that accumulate in the peripheral blood (PB), lymph nodes (LNs), bone marrow (BM), liver, and spleen. Although significant advances have been made in the treatment of CLL in the last decade, it remains incurable. Combination chemoimmunotherapy with fludarabine, cyclophosphamide, and rituximab (FCR) is now established as the standard of care, with overall response rates of 95%, and complete remission rates of 44%. However, this treatment is too toxic for many elderly patients, who constitute most of the individuals with this disease, and there remain subgroups of patients for whom this therapy has minimal activity. A critical feature of the inability of patients to tolerate FCR is that it exacerbates the immunodeficiency already present in CLL, which can result in severe infective complications. This article summarizes the current understanding of the immune defects in CLL, with particular emphasis on the role of T cells, natural killer (NK) cells, and nurselike cells (NLCs) in this disease. It also examines the potential clinical implications of these findings, focusing on the immunomodulatory aspects of current and novel pharmaceutical agents in addition to biologic therapies such as CD40 ligand transduction, vaccination strategies, and chimeric antigen receptor (CAR)–modified T cells.
Introduction
Chronic lymphocytic leukemia (CLL) is the most common leukemia in adults, with a lifetime risk of 0.49%. It is characterized by the clonal expansion of CD5+IgD+/IgM+ B lymphocytes that accumulate in the peripheral blood (PB), lymph nodes (LNs), bone marrow (BM), liver, and spleen. Although significant advances have been made in the treatment of CLL in the last decade, it remains incurable. Combination chemoimmunotherapy with fludarabine, cyclophosphamide, and rituximab (FCR) is now established as the standard of care, with overall response rates of 95%, and complete remission rates of 44%. However, this treatment is too toxic for many elderly patients, who constitute most of the individuals with this disease, and there remain subgroups of patients for whom this therapy has minimal activity. A critical feature of the inability of patients to tolerate FCR is that it exacerbates the immunodeficiency already present in CLL, which can result in severe infective complications. This article summarizes the current understanding of the immune defects in CLL, with particular emphasis on the role of T cells, natural killer (NK) cells, and nurselike cells (NLCs) in this disease. It also examines the potential clinical implications of these findings, focusing on the immunomodulatory aspects of current and novel pharmaceutical agents in addition to biologic therapies such as CD40 ligand transduction, vaccination strategies, and chimeric antigen receptor (CAR)–modified T cells.
T-cell defects in CLL
Abnormalities in T-Cell Numbers
Immune dysfunction is a key feature of CLL, highlighted by the hypogammaglobulinemia, increased susceptibility to infections, and increased incidence of autoimmune anemias that are commonly seen in this disease. As a consequence, attempts to understand the role of T cells in CLL have been active areas of investigation. It has been known for some time that the T-cell compartment is highly abnormal in CLL, with one of the earliest studies reporting an increase in absolute numbers of T cells in the PB. This expansion is primarily accounted for by an increase in CD8+ T cells, resulting in a decrease in the CD4/CD8 ratio, in contrast with the situation in the LNs and BM, where increased numbers of CD4+ T cells have been reported. The mechanism behind these changes remains unclear, although it has been suggested that the relative reduction in numbers of PB CD4+ T cells is caused by their increased susceptibility to FasL-mediated apoptosis. Several studies have linked changes in the numbers of circulating T-cell numbers to prognosis. Early work showed that there was greater expansion of CD8+ T cells, reflected in decreasing CD4/CD8 ratios, with advancing Rai stage. A more recent study has shown that inverted CD4/CD8 ratios predict shorter time to first treatment and reduced progression-free survival. Furthermore, other investigators have shown that patients with premalignant monoclonal B-cell lymphocytosis (MBL) seem to lack any significant expansion in T-cell numbers, in contrast with patients with CLL. However, there may be difficulties trying to prognosticate based on just the PB microenvironment, because another study found that increased numbers of circulating CD8+ T cells correlate with longer median time of survival.
Abnormalities in Cytokine Secretion Profiles
Despite their increased numbers, these T cells show profound defects in function and proliferative capacity, and abnormal cytokine secretion profiles. Early studies suggested that CLL was a Th2/Tc2-mediated disease, with increased numbers of CD4+ and CD8+ T cells producing interleukin (IL)-4. Further work showed that these IL-4–producing T cells also upregulate CD30 by an IL-4 and OX40L-dependent mechanism. Interaction of CD30 with CD30L expressed on the CLL cells results in increased tumor necrosis factor-α (TNF-α) production and CLL cell proliferation. In contrast, ligation of CD30L on the surface of the nonmalignant B cells impairs isotype class switching, and increases their sensitivity to FasL-mediated cell death, potentially contributing to the hypogammaglobulinemia observed in CLL. IL-4 also seems to be able to protect the CLL cells from undergoing apoptosis, by increasing/maintaining BCL-2 protein levels. There is evidence to suggest that IL-6 production by CLL cells drives this skew toward IL-4–producing T cells. Healthy T cells stimulated in the presence of tumor supernatant containing high levels of IL-6 have been shown to increase their production of IL-4, findings that could be replicated using recombinant IL-6, or reversed by removal or blockade of this cytokine. Furthermore, IL-6 has been shown to be increased in the serum of patients with CLL, with higher levels predicting poorer survival.
This shift toward a type 2 T-cell response was an attractive hypothesis given that these T cells could be expected to both enhance humoral immunity and help the malignant B cells, while suppressing Th1/Tc1-mediated cellular antitumor immune responses. However, several other reports have described increased production of interferon-γ (IFN-γ) and TNF-α by T cells from patients with CLL, showing correlation with disease stage. Furthermore, IFN-γ and TNF-α have been shown to protect CLL cells from apoptosis and induce their proliferation in vitro. It is probable that the situation in vivo is more complicated than the traditional Th1/Th2 paradigm, with interplay between a variety of cell types producing a mix of cytokines, chemokines, and cell surface molecules that protect the CLL cells from apoptosis and regulate their proliferation ( Table 1 ).
Cytokine | Action | Reference |
---|---|---|
IFN-γ | Protects CLL cells from apoptosis | |
TNF-α | Increases CLL-cell proliferation | |
TGF-β | Inhibits CLL-cell proliferation | |
IL-2 | Increases CLL-cell proliferation | |
IL-4 | Protects CLL cells from apoptosis | |
IL-5 | Induces CLL-cell apoptosis | |
IL-6 | Protects CLL cells from apoptosis; inhibits CLL-cell proliferation | |
IL-7 | Increases CLL-cell proliferation; protects CLL cells from apoptosis | |
IL-10 | Inhibits CLL-cell proliferation; induces CLL-cell apoptosis | |
IL-21 | Induces CLL-cell apoptosis |
Evidence for Chronic Activation: the Role of Cytomegalovirus?
Another feature of CLL T cells is that they show evidence of chronic activation, with upregulation of activation markers such as CD69, HLA-DR, and CD57, and downregulation of CD28 and CD62L. Several reports document expansions of clonal and oligoclonal T cells in CLL, in both CD4+ and CD8+ populations. There is also evidence that these oligoclonal expansions are primarily restricted to populations with an activated CD57+ phenotype, suggesting a role for chronic antigen stimulation in their development. However, it remains unclear whether this is directly related to CLL, or whether other factors such as cytomegalovirus (CMV) are involved. CMV is known to have a profound influence on distribution of lymphocyte subsets in healthy individuals, with CMV seropositivity driving the T-cell repertoire toward greater clonality in the elderly. Subsequent reports have shown an expansion of CMV-specific CD4+ and CD8+ T cells in patients with CLL, with an effector phenotype. It is therefore possible that the earlier reports of clonality and increased expression of activation markers merely reflect an immune response to CMV.
Alterations in Gene Expression: Cytoskeletal Dysfunction and Inhibitory Ligands
An important finding in the immunobiology of CLL was our work showing profound changes in the global gene expression profiles of T cells from patients with CLL compared with healthy age-matched controls. Despite not being part of the malignant clone, alterations were found in the expression of genes involved in cell differentiation and cytoskeletal formation in patients’ CD4+ T cells, and in cytoskeletal formation, vesicle trafficking, and cytotoxicity pathways in patients’ CD8+ T cells. Similar defects in gene expression could be induced in healthy T cells by coculturing them with CLL cells. These changes in the expression of cytoskeletal genes translated into a functional defect in filamentous actin (F-actin) polymerization, with T cells from patients with CLL having defective immunologic synapse formation with antigen-presenting cells (APCs). Again, this functional defect could be induced in healthy T cells by coculturing them with CLL cells. In both cases, the induction of the defects relied on direct contact between the CLL cells and the T cells, because the defects were not seen after coculture experiments using the Transwell system.
Similar defects could be observed in T cells taken from the Eμ-TCL1 mouse model of CLL. In this model, the TCL1 gene is placed under the control of a B cell–specific IgV H promoter and Ig H Eμ enhancer, resulting in mice that develop normally into adulthood, but then subsequently develop lymphadenopathy and hepatosplenomegaly, with increased numbers of circulating CD19+CD5+IgM+ lymphocytes. Despite this model being dependent on a single transgene, T cells from animals that had developed the disease showed alterations in the expression of cytoskeletal genes, and had dysfunctional immunologic synapse formation. Furthermore, infusion of CLL cells into young Eμ-TCL1 mice caused defects comparable with those seen in mice that had clinically apparent leukemia, showing causality of the CLL cells to induce the T-cell defects Another study showed that this model also accurately mimics the shift toward the antigen-experienced phenotype observed in the human disease. This work showed both a skewing of T-cell subsets toward antigen-experienced memory T cells, and development of clonal T-cell populations, consistent with a CLL-dependent antigen-driven skewing of the T cells in these mice.
We have examined the mechanism by which CLL cells induce these defects in the T-cell populations. Using functional screening assays based on actin polymerization responses to APCs, the molecules CD200, CD270 (herpes virus entry mediator [HVEM]), CD274 (programmed death ligand-1 [PD-L1]), and CD276 (B7-H3) induced impaired immunologic synapse formation in both allogeneic and autologous T cells. Higher expression of these molecules was observed on CLL cells compared with healthy B cells, with increased expression of CD200 and CD274 correlating with poor prognosis. Signaling axes involving these inhibitory ligands were also able to induce impaired F-actin polymerization in other cancers, suggesting that both hematologic and solid cancer cells use common immunosuppressive mechanisms to suppress T-cell–mediated antitumor immune responses. There is evidence that some of the receptors for these inhibitory ligands are increased on CLL T cells. Programmed death-1 (PD1; CD279) is an inhibitory T-cell receptor that has been postulated to be important in the maintenance of peripheral tolerance. Engagement of PD-1 by PD-L1 leads to the inhibition of T-cell receptor–mediated lymphocyte proliferation and cytokine secretion. We recently reported that PD-1 expression is increased on CD3+ T cells from patients with CLL and is an indicator of poor prognosis. Another group has reported similar findings, showing that PD-1 expression is increased on CD8+ T cells, with its expression predicting a more aggressive clinical course. However, another report has suggested that PD-1 expression is decreased on effector T cells, which are expanded in CLL. In addition to PD-1, we also have unpublished data showing that expression of a second inhibitory receptor, CD160, is increased on CLL T cells. CD160 interacts with HVEM to inhibit lymphocyte activation, along with B lymphocyte attenuator (BTLA). CD160 is normally expressed on NK cells, and a subset of CD8+ cytotoxic lymphocytes found in the intestinal epithelium. Circulating cytotoxic T cells from patients with CLL upregulate a range of other inhibitory receptors that are normally found on NK cells, including the killer inhibitory receptors CD158a, CD158b, and CD158e, and the C-type lectin receptor, CD94. The significance of this requires further investigation, but it is possible that pathways normally used in the regulation of NK-cell activity could be coopted to suppress CD8+ cytotoxic lymphocyte function in CLL.
Regulatory T Cells in CLL
In addition to increased expression of inhibitory receptors in CLL, there is also an increase in CD4+CD25 hi regulatory T cells (T regs ) in CLL. T regs have been shown to inhibit proliferation and cytokine release by conventional CD4+CD25− T cells, and increased numbers of T regs are associated with inhibition of antitumor immunity in solid cancers. The expansion of T regs in CLL has been postulated to be caused by 2 factors. First, there is evidence of increased formation, facilitated by CD27-CD70 interactions in LN proliferation centers. CD200 expression on the CLL cells may also play a role, because anti-CD200R antibodies promote development of T regs , whereas CD200 blockade significantly decreases T reg numbers. Second, T regs from patients with CLL have increased resistance to drug-induced apoptosis, compared with T regs from healthy donors, as a consequence of higher expression of BCL-2. The degree of increase correlates with disease stage, with the greatest increases in patients with the most clinically advanced disease. T reg numbers were also slightly increased in patients with MBL, although lower than in patients with CLL. The degree of increase in T regs has also been found to be of prognostic significance, with higher numbers of T regs predicting significantly shorter time to first treatment in 2 studies. T regs also express CD39, with increased expression of this molecule showing correlation with disease severity and prognosis in CLL. Higher frequencies of T regs have also been shown to correlate with decreased T-cell responses against viral and tumor antigens. A further feature of T regs is they express another inhibitory receptor, cytotoxic T lymphocyte–associated antigen-4 (CTLA-4; CD152). CTLA-4 is a homolog of CD28 and acts both directly, by decreasing phosphorylation of signaling proteins proximal to the T-cell receptor, and indirectly, by competing with CD28 for CD80/CD86 binding. T cells from patients with CLL have been shown to have significantly increased expression of both surface and cytoplasmic CTLA-4 compared with healthy controls, with expansion of a CD4+CD25+CTLA-4+ subset. Furthermore, although CTLA-4 is normally upregulated on T-cell activation, CLL T cells also showed a greater degree of upregulation of CTLA-4, and prolonged expression. Therefore, it is likely that CTLA-4 signaling is another inhibitory pathway mediating T-cell dysfunction in CLL, in addition to the PD-1:PD-L1, CD160:HVEM, and CD200:CD200R axes ( Fig. 1 ).
T Cells as Part of the Protumor Niche
In addition to the role for the CD4+CD25+ subset in the pathogenesis of CLL, evidence is emerging that implicates CD4+ T cells in the development and maintenance of this disease. As mentioned earlier, in contrast with the PB where CD8+ T cells are increased, CD4+ T cells are primarily expanded in the LNs and BM of patients with CLL. It has been suggested that this is caused by production of the chemokine CCL22 by CLL cells, which has been shown to be released into culture supernatants on CLL cell activation. These supernatants were able to induce the migration of activated CD4+CD40L+ T cells expressing the CCL22 receptor, CCR4: migration that could be blocked by antiCCL22 antibodies. A recent article highlighted the potential importance of CD4+ T cells in providing a protective niche for engraftment of CLL cells. The ability of primary human CLL cells to engraft and proliferate in a nonobese diabetes/severe combined immunodeficiency/γc null mouse model was assessed. Autologous CD4+ T cells from the patients with CLL were key mediators of CLL-cell growth in these mice, despite representing only a minor component of the transferred lymphocyte population. There was direct correlation between the degree of proliferation of T cells and that of the CLL clone, with selective depletion of CD4+ T cells leading to failure of CLL-cell growth. Further investigation is required to corroborate these findings in patients with CLL, but, taken together, these observations suggest that the expanded CD4+ T cells seen in BM and LNs are a critical part of the protumor microenvironment.
The role of other immune cells in CLL
NLCs and Chemokines
NLCs are another key constituent of the immune microenvironment implicated in providing a protective niche for CLL cells. NLCs are a subset of peripheral blood mononuclear cells (PBMCs) that differentiate into large, round, or fibroblastlike adherent cells in the presence of CLL cells. Although they are thought to originate from CD14+ monocytes, their expression of CD68 is significantly higher than normal monocyte-macrophages, making them more comparable with the CD68+ lymphoma-associated macrophages observed in follicular lymphoma. Further work identified these large CD14+CD68 HI cells in the spleens of patients with CLL, suggesting that they might also function to promote leukemia cell survival in vivo. An important feature of the biology of CLL cells is that they undergo spontaneous apoptosis when cultured in vitro, in marked contrast with their apparent longevity in vivo. However, when NLCs are present in vitro, the leukemic cells attach to them and are protected from undergoing spontaneous apoptosis. NLCs are thought to promote CLL-cell survival because of a combination of secretion of the chemokine CXCL12, and increased expression of B cell–activating factor of the TNF family (BAFF), and a proliferation-inducing ligand (APRIL). In addition, differentiation of monocytes into NLCs is associated with dysregulation of genes involved in antigen presentation and immunity, impairing their antitumor function. The receptor for CXCL12, CXCR4, is expressed at high levels on the surface of CLL B cells, with its expression correlating with Rai stage. Therefore, secretion of CXCL12 by both NLCs and stromal cells induces leukemia cell trafficking and homing to protective niches populated by these cells. Furthermore, coculture of CLL cells with NLCs induces the leukemic cells to release 2 T cell–attracting chemokines, CCL3 and CCL4. The production of these chemokines may explain the observation of increased numbers of T cells in CLL pseudofollicles, where the T cells seem to have been coopted to help B-cell activation and proliferation. Furthermore, increased CCL3 levels correlate with other adverse prognostic markers such as advanced clinical stage and poor-risk cytogenetics, and are predictive of shorter time to first treatment. In addition, CXCL12 acts as a costimulatory factor for CLL CD4+ T cells, resulting in their increased proliferation, cytokine production, and increased expression of activation markers. These activated T cells are then able to enhance the activation and proliferation of the leukemic cells. It is therefore possible that a mix of chemokines including CXCL12, CCL3, and CCL4 results in colocalization of stromal cells, NLCs, and activated CD4+ T cells, which provide sanctuary sites for the tumor cells, protecting them from chemoimmunotherapy and resulting ( Fig. 2 ) in drug resistance and disease persistence.
NK Cells
Abnormalities have also been found in other immune cells in CLL. Initial studies revealed that NK cells also have a functional defect in CLL, showing reduced ability to lyse leukemia cell lines associated with a lack of cytoplasmic granules. This activity could be restored by the use of IL-2, which also results in increased granularity of the large granular lymphocyte subset. The mechanism by which CLL cells downregulate NK-cell function is not known, although there is some evidence that it may involve soluble factors. CLL cells may also inhibit NK cells by direct contact, by their expression of the tolerogenic nonclassic major histocompatibility complex (MHC) class I molecule, HLA-G, or by expression of 4-1BB ligand. NK cells from patients with CLL show defective actin polymerization and impaired immunologic synapse formation, comparable with the cytoskeletal dysfunction seen in CLL T cells. The NK-cell defect seems to be of clinical significance, because higher NK-cell numbers were observed in patients with early-stage disease and in those with mutated IGHV genes. Furthermore, for patients with a given Rai stage, a higher NK/CLL cell ratio predicted a longer time to treatment, implying a protective effect of NK cells. In support of this, NK cells from patients with MBL have a higher cytolytic capacity than NK cells from patients with CLL. Other studies have shown that numbers of CD3+CD16+CD56+ NK T cells are also important, because a reduction in numbers is associated with disease progression and a higher risk of death in patients with CLL. An understanding of the NK-cell defect may prove to be clinically useful because preclinical studies have shown that NK cells from patients with CLL can be expanded and show cytotoxicity against K562 cells, highlighting their potential as a source of cellular immunotherapy.
There is also evidence suggesting that monocytes and neutrophils are defective in CLL, with the latter having been shown to be deficient in lysozyme and myeloperoxidase, and to modulate CLL B-cell survival through altered secretion of TNF-superfamily proteins.
Potential clinical implications: immunotherapy for CLL
Immunomodulatory Aspects of Cytotoxic Chemotherapy
Virtually all treatment of CLL is immunomodulatory. Treatments that are cytotoxic to the malignant B cells are invariably toxic to other leucocytes, both granulocytes and lymphocytes. One of the most important clinical aspects of cytotoxic chemotherapy is the degree to which it exacerbates the existing immunodeficiency. The ability of a patient to tolerate the treatment is often an important predictor of overall prognosis. In addition to the effects of cytotoxic agents on the tumor cells, there is also the potential for differential toxicity to immune components of the protumoral microenvironment. The purine analogue fludarabine is widely used for the treatment of CLL. Because T-cell depletion is a commonly encountered side effect of this agent, it can be hypothesized that fludarabine treatment has a significant impact on the T-cell microenvironment. Analysis of the composition and function of the T-cell pool in patients with CLL after treatment with fludarabine and cyclophosphamide revealed a rapid and sustained reduction of circulating CD4+ and CD8+ T cells, as expected. T cells surviving this combination had a more mature phenotype, and fludarabine-treated T cells were significantly more responsive to mitogenic stimulation than their untreated counterparts, and showed a shift toward Th1 cytokine secretion. Furthermore, fludarabine therapy seems to have a particularly marked effect on T regs , with both a reduction in the inhibitory function and a decrease in the frequency of this subset after treatment. Both these studies were performed on PB samples, so it would be particularly interesting to examine the impact of fludarabine on CD4+ T cells in LNs and BM.
Improved NK-cell Functionality with Rituximab
Rituximab is a humanized anti-CD20 monoclonal antibody (mAb) widely used in the treatment of B-cell malignancies and autoimmune diseases. The mechanism of action of this agent is not clear, although it seems to be a combination of several factors including antibody-dependent cellular cytotoxicity (ADCC), complement-dependent cytotoxicity (CDC), induction of apoptosis of CD20+ B cells, inhibition of B-cell receptor (BCR) signaling, and regulation of the cell cycle. The efficacy of this agent in CLL is perhaps even more paradoxic, given the low expression of its target antigen on CLL cells compared with normal B cells and other malignant B cells. An important component of the mechanism of action of rituximab in CLL seems to be the enhancement of NK-cell functionality. Opsonization of CLL cells by anti-CD20 antibodies such as rituximab allows binding of the NK-cell Fc receptor, FcγRIII (CD16), to the Fc region of the antibodies. Antibody binding to CD16 results in NK-cell activation, release of cytolytic granules, and consequent target cell apoptosis. Rituximab has been shown to increase NK-cell degranulation as measured by the CD107a assay. There is evidence that FcγR polymorphisms predict response to rituximab in patients with lymphoma. Third-generation monoclonal anti-CD20 antibodies such as obinutuzumab (GA-101) and LFB-R603 that have higher binding affinities for this receptor have been shown to be even more efficient at inducing NK-cell degranulation. In vitro experiments have shown that NK-cell functionality can be further enhanced by coculturing the NK cells with cytokines such as IL-21 and IL-15. Opsonization of the tumor cells by rituximab may also be important for the activity of other immune cells, because there is evidence that rituximab enhances macrophage killing of CLL cells by increasing phagocytosis-mediated ADCC.
Eviction from the Niche: the Mobilizing Effect of BCR Signaling Inhibitors
There has been a great deal of recent interest in inhibitors of BCR signaling for the treatment of CLL. BCR signaling is known to be crucial for B-cell proliferation and survival, and several major prognostic markers that are clinically useful in CLL are associated with aberrations in BCR signaling. Absence of mutations in immunoglobulin variable (IgV) region genes and expression of zeta-chain–associated protein kinase 70 (ZAP-70) have been shown to correlate with an inferior outcome, and are both associated with increased signaling through the BCR. This aberrant BCR signaling was previously thought to be antigen dependent, but a recent publication showed that BCRs from patients with CLL induce antigen-independent cell-autonomous signaling, dependent on the heavy-chain complementarity-determining region (HCDR3) and an internal epitope of the BCR. Several molecules that inhibit kinases downstream of the BCR have been developed and are showing great promise in early-phase clinical trials. An unexpected feature of some of these agents is that there is a transient increase in the circulating lymphocytosis after initiation of treatment, associated with rapid reductions in LN size, consistent with mobilization of the tumor cells from the LNs into the blood. The phosphatidylinositol 3-kinase (PI3K) inhibitor GS1101, the Bruton tyrosine kinase (Btk) inhibitor ibrutinib (PCI-32765), and the mammalian target of rapamycin (mTOR) inhibitor, everolimus, have all been found to induce this lymphocytosis during the first weeks of treatment. As discussed earlier, the CXCL12-CXCR4 axis is important for the homing of CLL cells to potential sanctuary sites in the BM and LNs. A small-molecule inhibitor of CXCR4, plerixafor, has also shown clinical activity in patients with CLL, again associated with mobilization of tumor cells into the circulation. It is therefore likely that some of the clinical activity of these novel therapies may be caused by their ability to evict CLL cells from protective microenvironmental niches populated by NLCs, stromal cells, and T cells.
CD40L Based Therapies
A key feature of CLL cells is that they have impaired antigen presenting function, caused by reduced expression of costimulatory molecules including CD80 and CD86. CLL-cell activation by ligation of CD40 can upregulate these proteins, along with adhesion molecules such as intercellular adhesion molecule-1 (ICAM-1/CD54). Under normal circumstances, this activation signal is provided by T cells, which transiently express CD40L (CD154) after antigen engagement of their T-cell receptors (TCRs). However, this critical axis is impaired in CLL, because the tumor cells seem to be able to directly downmodulate T-cell expression of CD40L. In light of this, several strategies have been developed to reexpress CD40L in the tumor microenvironment, thereby improving CLL-cell antigen-presenting function and overcoming T-cell anergy.
One such strategy has been to use adenoviral vectors to transduce CLL cells to express CD40L. In addition to inducing expression of costimulatory and adhesion molecules on the transduced cells, increased expression of CD40L in the CLL microenvironment can activate other bystander CLL cells, even if they were not transfected. Further preclinical work showed that the transfected CLL cells become effective stimulators of mixed lymphocyte reactions, and induce generation of cytotoxic T cells specific for autologous unmodified CLL cells. Similar findings have been reported by culturing CLL cells on CD40L-expressing feeder cells in place of transfection. Use of fibroblasts overexpressing CD40L and OX40L to transfer both these ligands to CLL cells also resulted in the generation of tumor-reactive cytotoxic T lymphocytes. There were some initial concerns that CD40-stimulated CLL cells may become more resistant to apoptosis induced by fludarabine, because of upregulation of the NF-κB/Rel transcription factors. Gene expression profiling performed on CD40-stimulated CLL cells also identified upregulation of several genes involved in the suppression of apoptosis mediated by the TNF family of receptors and NF-κB. However, further investigation showed that, although CD40 stimulation enhanced the constitutive antiapoptotic profile of the CLL cells, and these cells were not sensitive to CD95L, they remained good targets for cytotoxic T cells.
An early clinical trial examined the effects of infusions of autologous tumor cells that had been transduced ex vivo with murine CD40L, which was more efficiently expressed than human CD40L. This treatment was well tolerated and the patients showed some PB and LN responses. However, some of the patients developed antibodies against the murine CD40L. In light of this, a recombinant humanized CD40 binding protein, ISF35, was developed, and was tested in a more recent phase I study. The infusions of transduced autologous tumor cells were again well tolerated, and were consistently followed by reductions in circulating lymphocyte counts and lymphadenopathy. The increased levels of this humanized CD40L were associated with induction of a proapoptotic state in the circulating CLL cells, with increased expression of proapoptotic molecules CD95, DR5, p73, and BCL-2 interacting domain (BID), and reduced levels of the antiapoptotic molecule, MCL-1. These findings were also observed in patients with deletion of chromosome 17p. There is a rationale for combining this approach with rituximab, because stimulation of CLL B cells by CD40L sensitizes them to rituximab-induced cell death. However, evidence has emerged that there is significant heterogeneity in the responses of CLL B cells to CD40L stimulation. Patients with CLL B cells that were unresponsive to CD40L showed a poor clinical outcome and shorter time to progression, which was thought to reflect less dependency on the microenvironment and higher autonomous proliferative and survival potential.
Other work has investigated whether there is a role for anti-CD40 mAbs in the treatment of CLL. Two therapeutic antibodies that target the CD40 antigen are in development, dacetuzumab (SGN-40), which acts as a partial agonist, and lucatumumab (HCD122), which blocks CD40-mediated signaling. However, phase I trials using these agents have shown minimal single-agent activity, but they warrant further investigation as part of combination therapies. Preclinical experiments have suggested that dacetuzumab treatment in combination with the immunomodulatory agent lenalidomide enhanced direct apoptosis and ADCC against primary CLL cells. However, it is possible that this activity represents tumor cell opsonization and the induction of NK-cell responses, as much as a direct biologic effect of CD40 binding.
Vaccine Therapy for CLL
The observation that CLL cells maintain some residual B-cell function, which can be enhanced by appropriate stimulation, has led to attempts to induce an effective autologous immune response by vaccine therapy. A vital component of this approach is identifying a suitable tumor-associated antigen (TAA) with which to induce an immune response. An ideal TAA should be only expressed on the tumor cells to avoid induction of autoimmunity, should be expressed on all of the tumor cells, and should be essential for tumor cell survival to prevent the emergence of antigen-negative variants and immune escape. The major challenge to cancer vaccines is that the tumor cells are derived from self, with the consequence that few TAAs have these characteristics. In light of this, the search for possible TAAs has focused on proteins that are either commonly mutated in the malignant cells or are aberrantly expressed. Serologic identification by recombinant expression cloning (SEREX) has been used to detect such mutated proteins, and cytotoxic T-cell responses could be generated against these antigens. Several other TAAs have been identified in CLL, including survivin, fibromodulin, RHAMM (CD168), FMNL1, MDM2, and CD23. Of particular interest is the oncofetal surface antigen receptor tyrosine kinase–like orphan receptor 1 (ROR1), which has the advantage that it is selectively expressed by malignant B cells, although it is also expressed by undifferentiated embryonic stem cells, and at low levels in adipose tissue. When 6 patients with CLL were infused with autologous CLL cells that had undergone adenoviral transfection with CD154, 3 of them (50%) subsequently generated antibodies against ROR1. Furthermore, upregulation of CD154 by lenalidomide has also recently been shown to induce production of ROR1 tumor-specific antibodies, notably in a patient who had not received prior therapy with rituximab.
A particular feature of B-cell malignancies is that there is a further type of TAA in the form of the immunoglobulin idiotype. The surface immunoglobulin expressed by the malignant B cells is unique to each clone, and thus represents an attractive target for vaccine strategies. Using a bioinformatics approach, several human immunoglobulin–derived peptides were identified that were capable of inducing cytotoxic T cells. Furthermore, autologous cytotoxic T cells specific for these peptides were able to kill primary malignant CLL cells. One of the problems with this method is that the uniqueness of the idiotype means that any potential vaccine would have to be individualized to each patient. However, peptides generated from the framework regions of the immunoglobulin molecule, regions that show less variability between patients, were immunogenic, allowing a single such peptide to be used in several patients. A second problem with idiotype vaccination strategies is that the immunoglobulin idiotype is only weakly immunogenic, and often only generating humoral responses. A solution to this is the use of heteroclitic peptides, in which MHC-binding amino acid residues are modified to bind MHC more tightly, while leaving the T-cell recognition residues intact, inducing more potent immune responses.
In the normal immune system, the most potent APCs are dendritic cells (DCs). This property can be exploited by manipulating DCs to take up antigen in vitro, before presenting it to T cells, causing their activation and proliferation. There have been several clinical studies that have investigated the use of DCs in CLL. In one such study, DCs were generated from unrelated donors, before being pulsed ex vivo with patient CLL-cell lysate or apoptotic bodies. They were subsequently administered to patients with early-stage CLL, a subset of whom showed clinical responses. These investigators then studied autologous DCs pulsed with CLL-cell lysates, and a subset of patients showed responses associated with an increase in T cells specific for RHAMM/CD168 and fibromodulin. A further study used oxidizing radiation to augment the immunogenicity of the CLL cells by improving their antigen-presenting function, resulting in enhanced T-cell antitumor activity and clinical responses.
Lenalidomide
Lenalidomide has recently been shown to have significant clinical activity in CLL. Its mechanism of action is not well understood, but it is thought to act primarily by enhancing antitumor immunity and reducing production of protumoral factors in the CLL microenvironment. Lenalidomide and its analogues thalidomide and pomalidomide, were originally identified for their ability to inhibit production of TNF-α from lipopolysaccharide-stimulated PBMCs in vitro. Lenalidomide has also been shown to have costimulatory effects, causing T-cell activation with increased production of IL-2 and IFN-γ, and triggering tyrosine phosphorylation of CD28 with downstream activation of NF-κB. A key part of these agents’ mechanism of action seems to be their ability to activate cytoskeletal pathways, which are critical regulators of T-cell activation. In particular, it has been shown that pomalidomide can activate the cytoskeletal regulators Rac1 and RhoA in CD4+ T cells. We have shown that the functional defect of T cells in CLL is associated with alterations in the expression of cytoskeletal genes and defective immunologic synapse formation. We subsequently showed that treatment of both autologous T cells and CLL cells with lenalidomide resulted in repair of this defect, suggesting that this may be a key component of this agent’s activity in CLL.
In addition, there is also evidence to suggest that lenalidomide has effects on CLL cells directly, by inducing CD40L expression through a PI3K kinase–dependent pathway. The CD40L-positive CLL cells also upregulate BID, DR5, and p73, sensitizing them to TNF-related apoptosis-inducing ligand (TRAIL)–mediated apoptosis. Lenalidomide-induced upregulation of CD40L is of particular interest, because the CLL cells also upregulate expression of CD40, CD80, and CD86, in a similar manner to CD40L-transfected CLL cells. The increased expression of these costimulatory ligands has been found to correlate with a unique aspect of the clinical activity of lenalidomide in CLL, the tumor flare reaction. This reaction is manifested by acute swelling of involved LNs, hepatosplenomegaly, rash, and fever, and is thought to be caused by lenalidomide-induced CLL-cell and T-cell activation. There has been some suggestion that the presence of a tumor flare reaction correlates with clinical outcome, highlighting that the mechanisms underlying this phenomenon may also account for lenalidomide’s antitumor effect. The existence of this reaction, as well as the unexpected finding of increased hematologic toxicity, has meant that patients with CLL are generally unable to tolerate the same doses of lenalidomide that have been used in other hematological malignancies such as myeloma. Lenalidomide also has effects on other nonmalignant lymphocyte subsets. It has been shown to significantly inhibit the suppressor function and generation of T regs in vitro, and was found to reduce numbers of T regs in PB of patients with CLL. Lenalidomide has also been shown to downregulate the expression of inhibitory receptors on NK cells, and to enhance antigen-specific expansion of NK T cells. It seems to be particularly effective when used in combination with rituximab, because of its ability to augment NK cell–mediated cytotoxicity, potentially as a result of improved immunologic synapse formation.
A recent article identified cereblon (CRBN) as a primary target of thalidomide teratogenicity. Thalidomide binds to CRBN, inhibiting the activity of a E3 ubiquitin ligase complex composed of CRBN, DNA damage–binding protein-1 (DDB1), cullin 4A, and ring-box protein-1 (RBX1, also known as Roc1). This binding was shown to lead to the downregulation of fibroblast growth factor genes and teratogenic effects in zebra fish and chick models. CRBN is also implicated in other pathways, because it binds to a calcium-activated potassium channel in the brain, a chloride channel in the retina, and to adenosine monophosphate kinase, a master sensor of energy balance in eukaryotic cells. In addition, a nonsense R419X mutation of CRBN is associated with autosomal recessive nonsyndromic mental retardation. Further work has shown that CRBN binding is also a critical component of the activity of lenalidomide and pomalidomide, because its expression was required for the antimyeloma effect of these agents. Furthermore, CRBN depletion is associated with the resistance of myeloma cell lines to both of these drugs, and to lack of clinical responses in patients on lenalidomide treatment. It is not clear what the implications of these findings are for CLL because, in contrast with myeloma, lenalidomide is not directly cytotoxic to CLL cells. However, evidence is emerging that CRBN is also critical for the effect of lenalidomide and pomalidomide on T-cell activation, because siRNA knockdown of T-cell CRBN blocked the increase in production of IL-2 and TNF-α induced by these agents.
Lenalidomide has been shown to have significant clinical activity in CLL, with an overall response rate as a single agent in treatment-naive patients of 56%. This finding compares well with responses seen with commonly used therapies such as fludarabine, alemtuzumab, bendamustine, and chlorambucil. Furthermore, lenalidomide has been shown to have efficacy in patients with poor-risk cytogenetics with overall response rates of 38% in patients with del11q or del17p. A recent phase II trial has shown similar activity in elderly (≥65 years) patients, with lenalidomide being well tolerated. Trials are underway to examine the efficacy of combining lenalidomide with other agents, but the presence of the tumor flare reaction and other toxicities has meant that the concurrent administration of fludarabine, rituximab, and lenalidomide was not well tolerated. Given that T-cell activation seems to be a crucial component of this drug’s effect in this disease, it can be postulated that combinations with T-cell–depleting agents such as fludarabine or alemtuzumab may be counterproductive. However, the situation may be more complex, because there is some evidence that lenalidomide can still be effective after T-cell depletion by alemtuzumab and high-dose glucocorticoids. In contrast, the addition of rituximab to lenalidomide seems particularly attractive, with rituximab improving responses relative to single-agent lenalidomide, despite the patients having received this monoclonal antibody as part of previous therapy. Trials to evaluate the efficacy of this combination as first-line treatment, and also to investigate the combination of lenalidomide and ofatumumab, are ongoing. Furthermore, there is evidence that lenalidomide consolidation may prolong progression-free survival after induction treatment with pentostatin, cyclophosphamide, and rituximab.
Adoptive T-Cell Therapies
Allogeneic hemopoietic stem cell transplantation remains the only curative option for CLL. Critical to its activity is the graft-versus-leukemia (GVL) effect, in which the transplanted hemopoietic stem cells differentiate into effector cells that mount an antitumor immune response. This effect is known to be primarily T-cell mediated, and is probably caused by a combination of improved T-cell function and the presence of allogeneic MHC molecules. The major drawback of myeloablative allogeneic transplantation is that it is associated with unacceptably high rates of nonrelapse mortality, which can approach 50% even in younger patients because of a combination of toxicity from the conditioning regimens, the prolonged period of immunosuppression during engraftment, and graft-versus-host disease (GVHD). One way of attempting to reduce nonrelapse mortality is to use reduced-intensity conditioning (RIC) to exploit the GVL effect while avoiding the significant morbidity and mortality associated with myeloablative conditioning. RIC regimens are associated with decreased mortality, but GVHD remains a significant problem. Therefore, for most patients who are more than 65 years of age, allogeneic hemopoietic stem cell transplantation is not a reasonable option.
One approach to circumventing the problem of GVHD is the adoptive transfer of T cells with specificity for tumor antigens. By choosing T cells with specificity only for tumor cells, it should be possible to produce immune-mediated antitumor responses without the corresponding GVHD. There are 2 main strategies for generating tumor-specific T cells. The first involves the gene transfer of TCRs with known specificity into autologous or allogeneic T cells that are then expanded in vitro and infused into patients. This approach has had some successes, most notably in melanoma and the use of Epstein-Barr virus–specific T cells to treat posttransplant lymphoproliferative disorders. However, a potential risk of this approach is that the alpha or beta subunit of the transgenic TCR could misassociate with the alpha or beta subunit of the endogenous TCR, resulting in an autoreactive T cell. Furthermore, a particular drawback to widespread clinical use is that the recognition of the tumor antigens is MHC restricted, and therefore the use of these T cells needs to be individualized on a patient-by-patient basis according to their MHC type. A second strategy involves the use of the single-chain variable fragment from an antibody molecule fused with an internal signaling domain such as CD3ζ, to form a CAR. There are 2 important advantages to this approach. First, it eliminates MHC restriction, enabling the same CAR to be used for several different patients. Second, the use of an antibody receptor means that potential targets can be increased to include a wide range of surface proteins, sugars, and lipids.
Several phase I/II clinical trials are underway using anti-CD19 CAR T cells for the treatment of B-cell malignancies. One group has treated 8 patients with CLL in 2 cohorts. The first cohort of 3 patients did not receive any conditioning, and did not show any objective responses. They went on to give the next patient lymphodepleting conditioning with cyclophosphamide as part of the trial design. However, this patient rapidly developed hypotension, respiratory distress, and renal failure, and died within 48 hours of infusion of the T cells, highlighting the risks associated with this therapy. They have gone on to treat a further 4 patients with cyclophosphamide conditioning and a reduced dose of T cells, with 3 out of 4 of the patients showing disease stabilization or LN responses. A second group has investigated the use of anti-CD19 CAR T cells in 8 patients with progressive B-cell malignancies, including 4 patients with CLL. These patients received conditioning with cyclophosphamide and fludarabine, followed by infusion of anti-CD19 CAR T cells. They also received a postinfusion course of IL-2 to enhance in vivo T-cell expansion. Six of the 8 patients obtained objective remissions: of the 4 patients with CLL, 1 had a complete response, 2 had partial responses, and 1 had stable disease. This trial also investigated some of the potential adverse effects associated with the use of CAR T cells. Four out of the 8 patients had increased serum levels of IFN-γ and TNF, which correlated with the severity of acute toxicities. It is likely that the CAR T cells were a source of these inflammatory cytokines because T cells isolated from the patients after treatment produced IFN-γ and TNF-α ex vivo in a CD19-specific manner. These trials underscored the importance of the conditioning regimen in promoting T-cell engraftment and activation. As discussed earlier, it may be vital to use conditioning regimens that deplete the microenvironment of protumoral cell types such as regulatory T cells or immature dendritic cells. One important aspect of forthcoming clinical trials in this area will be the determination of which agents to use in the conditioning regimen, analogous to the impact of conditioning in allogeneic transplantation.
A key finding in preclinical studies of CAR T cells was that their efficacy could be significantly improved by the addition of a costimulatory domain such as CD28. It is becoming apparent that the choice of costimulation can have significant impact on the clinical success of CAR T cell–based therapies. Many of the CAR T cells currently under investigation in clinical trials have incorporated the use of the CD28 costimulatory domain, including the 2 trials discussed earlier. However, in a murine model of primary human pre–B-cell acute lymphoblastic leukemia, human T cells expressing anti-CD19 CARs containing the costimulatory domain CD137 (4-1BB) were significantly more effective and showed longer survival than cells expressing CARs containing the CD28 domain. Furthermore, preclinical data suggest that CD137 is less likely to trigger IL-2 and TNF-α secretion, and hence induction of a cytokine storm and differentiation of regulatory T cells. In light of this, other investigators have trialed the use of anti-CD19 CAR T cells incorporating a CD137 costimulatory domain. A recent report documented a case of a heavily pretreated patient with refractory CLL who entered a complete remission after the adoptive transfer of anti-CD19 CAR T cells. A significant feature of this case was that these cells were still detectable at 6 months after infusion, and had started to express molecules associated with a central memory phenotype, which is known to be important in maintaining robust and persistent antitumor immune responses. A further 2 cases have been reported, 1 of which also achieved complete remission after being heavily pretreated. Although this is encouraging, there remain many unresolved questions. One-hundred and sixty-nine days after infusion, the CAR T cells had high expression of CD45RA, PD-1, and CD57, which may reflect the extensive replication history of these cells leading to T-cell exhaustion and incipient loss of function. This situation could lead to treatment failure in the long term and necessitate further infusions of these T cells to maintain clinical responses. Furthermore, the risk associated with profound long-term B-cell lymphopenia and hypogammaglobulinemia is still unknown, and could result in increased susceptibility to infections analogous to Bruton X-linked agammaglobulinemia. As discussed earlier, other issues remain, such as the best time to use these cells, the type of conditioning and costimulatory domain, and which antigen is best to target. The malignant cells may develop the ability to downregulate CD19, necessitating the use of CAR T cells targeting alternative tumor antigens such as CD23 and ROR1, or the use of combinations of CAR T cells specific for a range of antigens. However, the potential of CAR T cells, and the possibility of engineering other cell types with CARs such as NK and NK T cells, means that this remains an exciting area of research.
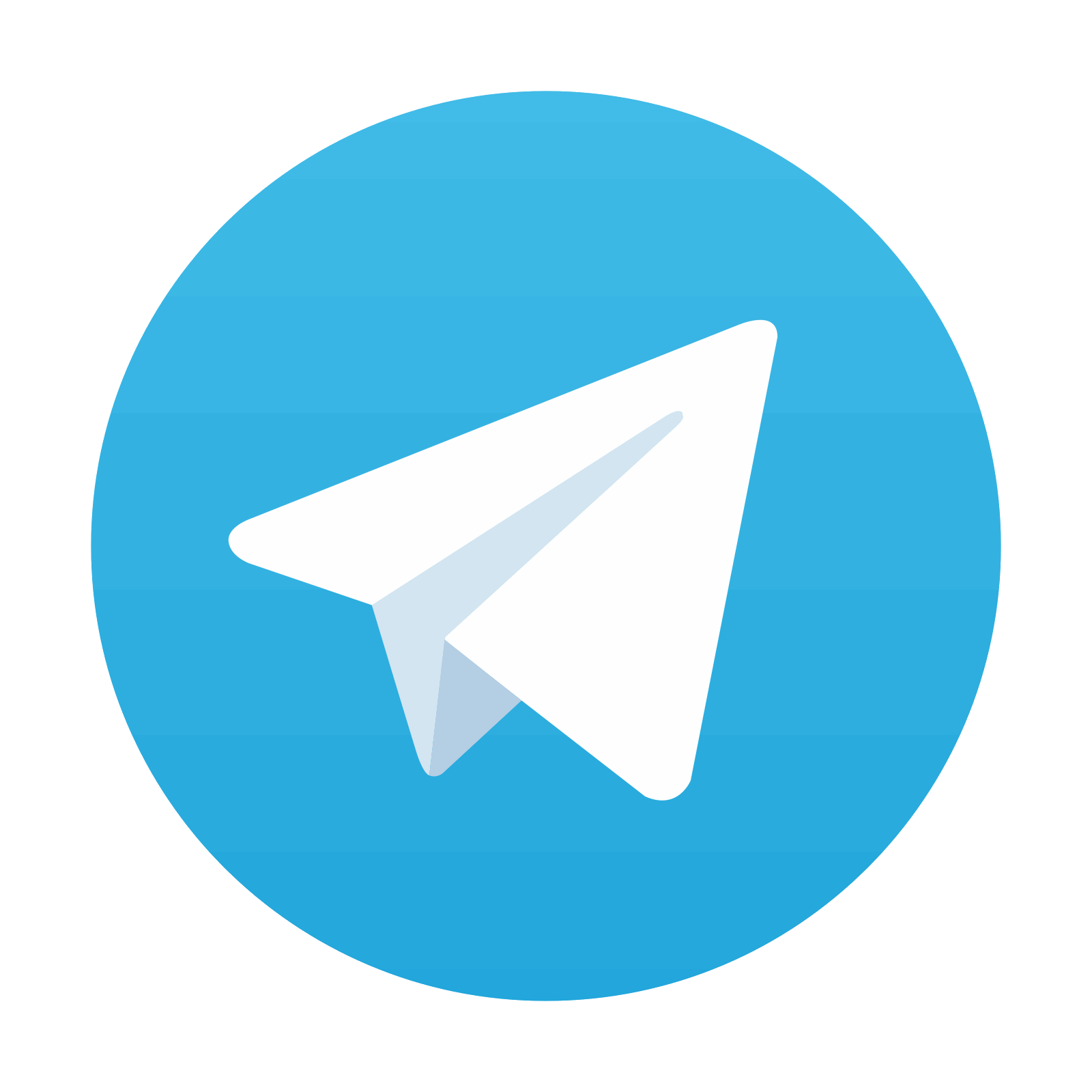
Stay updated, free articles. Join our Telegram channel

Full access? Get Clinical Tree
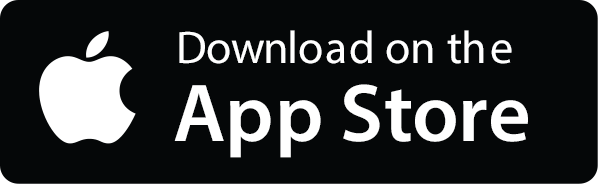
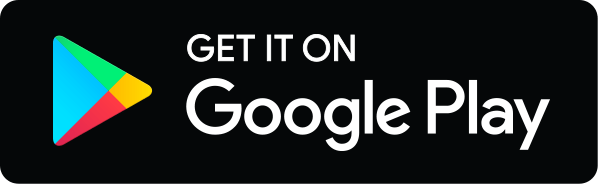