Fig. 5.1
TAM in modulating cancer-related inflammation. TAM participate in many aspects of cancer growth and progression. More in details, they sustain tumor growth through the release of growth factors, promote the remodeling of the ECM through the production of proteases and soluble mediators, sustain angiogenesis and lymphangiogenesis through the release of MMP-9 or other soluble factors, and suppress the antitumoral immune response through the expression of immunosuppressive molecules, such as IL-10, IDO, and TGF-β
TAM contribute to the ECM remodeling and promote tumor cell invasiveness and metastasis due to the release of several proteolytic molecules, such as plasmin, uPA, cathepsin B and matrix metalloproteinases (MMP) (Gocheva et al. 2010; Nagakawa et al. 2002; Wang et al. 2011), and non-proteolytic molecules. As one of the first evidences, transfer of thioglycollate-elicited peritoneal macrophages in mice significantly increased the number of metastatic lung nodules induced by the intravenous injection of melanoma or Lewis lung carcinoma tumor cells (Gorelik et al. 1982). Conditioned media derived from mammary epithelial cells containing FGF receptor 1-induced soluble factors promoted the macrophage expression of CXCR2 ligands which, in turn, induced tumor cells migration and invasion (Bohrer and Schwertfeger 2012). In a mouse pancreatic cancer xenograft model, macrophages-associated scavenger receptor A was essential for cancer metastasis (Neyen et al. 2013). Moreover, macrophages promoted the expression of MMP-9 in glioma stemlike cells through TGF-β1, thus contributing to the invasive behavior of tumor cells (Ye et al. 2012). Finally, the macrophage expression of the antimicrobial peptide hCAP18/LL37 was enhanced by tumor-derived versican V1 and contributed to ovarian tumor cell proliferation and invasion (Li et al. 2013). In human hepatocellular carcinoma (HCC), TAM were found to correlate with cancer stem cells density and promoted cancer stem cell properties in hepatoma cell lines through TGF-β1, favoring tumor invasiveness and metastasis (Fan et al. 2014). In a mouse model of breast cancer, IL-4 induced macrophage upregulation of the protease cathepsin B, which promoted lung metastasis (Vasiljeva et al. 2006). Moreover, tumor cells-conditioned media induced in macrophages the expression of a truncated fibronectin isoform, namely, migration-stimulating factor (MSF), a potent chemotactic factor for tumor cells (Solinas et al. 2010).
Tumor angiogenesis and lymphangiogenesis may also be modulated by TAM, via the production of mediators involved in new vessel formation and sprouting (Schmidt and Carmeliet 2010). For instance, TAM-derived MMP-9 favors the release of heparin-bound growth factors, particularly VEGF-A, crucial for the angiogenic switch (Ebrahem et al. 2010). Prostate cancer-derived CCN3 skewed macrophages to the M2 phenotype, which contributed to angiogenesis in a VEGF-dependent manner (Chen et al. 2014). Moreover, in an elegant in vivo model of organ-specific VEGF-induced monocytes recruitment, Ly6Chi monocytes undergo phenotypic and functional changes, contributing to proliferation of endothelial cells and vessels remodeling (Avraham-Davidi et al. 2013). Tumor-associated hypoxia has been described as a master regulator of TAM distribution and differentiation. In the tumor microenvironment, low-oxygen tension increased the expression levels of HIF-1 and HIF-2, which triggered a proangiogenic program in macrophages leading to high expression levels of VEGF, bFGF, CXCL8/IL-8, and glycolytic enzymes (Murdoch et al. 2004). In addition, high levels of adenosine present in the tumor microenvironment during local hypoxia also contributed to the release of angiogenic and lymphangiogenic factors from macrophages (Granata et al. 2010). Hypoxia-induced cancer cell-derived Sema3A, through Nrp1 signaling, is responsible for TAM recruitment into hypoxic niches, where they escape antitumor immunity and promote neoangiogenesis (Casazza et al. 2013). Impeding TAM “migration” from normoxic to hypoxic tumor areas by affecting the Sema3A/Neuropilin-1 pathway could reestablish antitumor immunity and limit angiogenesis, overall inhibiting tumor growth and metastasis.
TAM may also take part in tumor-promoting immunosuppressive networks, through the release of molecules (such as TGF-β, iNOS, arginase-1, IDO, and IL-10) known for their immunosuppressive effects (Sica and Mantovani 2012; Zhao et al. 2012). In mouse models of breast cancer, T cell suppression depends, at least in part, on TAM-associated arginase-1 or iNOS expression (Bronte and Zanovello 2005; Doedens et al. 2010; Movahedi et al. 2010), whereas in humans it may also occur regardless of the l-arginine pathway (Kryczek et al. 2006; Chen et al. 2012, 2013). Tumor-infiltrating hematopoietic cells and epithelial cancer cells may also fuse, making tumor cells acquire the expression of hematopoietic markers, including CD45 and CXCR4, thus favoring tumor cells dissemination and escape from immune-mediated elimination (Ramakrishnan et al. 2013).
Because of their overall pro-tumor effects, TAM-targeting promises to be a useful tool for anticancer therapy. Accordingly, several therapeutic strategies have been proposed to interfere with their recruitment, modulate their survival, or reprogram them into a M1 inflammatory antitumor phenotype (Beatty et al. 2011; Germano et al. 2013; Edwards and Emens 2010).
5.4 Therapeutic Targeting
Targeting their recruitment and polarization is the milestone of the TAM-mediated anticancer therapy (Ries et al. 2014). Accordingly, preclinical models strongly support the anti CCL2 therapy in prostate and breast cancer (Qian et al. 2011; Zhang et al. 2010). Anti-CSF-1 antibodies and antisense oligonucleotides have been described to reduce macrophage infiltration and xenograft mammary tumor growth in mice (Aharinejad et al. 2004; Paulus et al. 2006). Quite recently, a new monoclonal antibody inhibiting CSF-1R activation has been developed that strongly reduced F4/80+ TAM infiltration in animal models; in a phase 1 clinical trial, this drug reduced CSF1R + CD163+ TAM with a systemic clinical positive impact in diffuse-type giant cell tumors (Ries et al. 2014). In line with this evidence, another anti-CSF1R antibody has been recently developed which inhibited macrophage polarization toward M2 phenotype, skewing their differentiation toward dendritic cells (Haegel et al. 2013). TAM-targeting may also modulate the response to chemotherapy. In a phase II clinical trial, a partial clinical effect was obtained in patients with advanced pancreatic cancer treated with the fully human CD40 agonist antibody in combination with gemcitabine chemotherapy, likely dependent on a modified macrophage phenotype showing upregulation of MHC class II and CD86 expression (Beatty et al. 2011). Accordingly, in a gallbladder cancer model, the Th1 cytokine IFNγ promoted the antitumor effector functions of CD40 ligand-activated macrophages (Luheshi et al. 2014), and, even though favored monocyte/macrophages recruitment, it inhibited TAM differentiation, reducing VEGF levels and tumor angiogenesis (Sun et al. 2014). In a genetic mouse model of breast cancer, paclitaxel chemotherapy increased CSF1 receptor-expressing TAM: blockade of macrophage recruitment with inhibitors of CSFR1 in combination with chemotherapy-enhanced therapeutic activity, inhibited metastases, and increased T cell infiltration in the tumors (Denardo et al. 2011). Trabectedin is an EMEA-approved natural product derived from the marine organism Ecteinascidia turbinata, displaying potent antitumor activity; it is specifically cytotoxic for human and murine macrophages and TAM (Germano et al. 2010), and targeting TAM has recently been shown to mediate its antitumor action (Germano et al. 2013).
An alternative TAM-targeting strategy could consist on targeting their effector functions. The bisphosphonate zoledronic acid is a classical MMP inhibitor. In cervical cancer, it suppressed TAM-related MMP-9 expression and activity, reducing angiogenesis and carcinogenesis (Giraudo et al. 2004). The bisphosphonate derivative clodronate efficiently depleted TAM in murine teratocarcinoma and human rhabdomyosarcoma mouse models, resulting in inhibition of tumor growth (Zeisberger et al. 2006). Enhanced 15-Lipoxigenase-2 (15-LOX2) activity in renal cell carcinoma (RCC) has been implicated in monocytes recruitment through the CCL2-CCR2 axis. In turn, TAM exerted immunosuppressive actions by inducing FOXP3 and CTLA4 in LOX-independent fashion. Therapeutic approaches aimed at manipulating the 15-LOX2-mediated arachidonic metabolism could be useful to subvert the immunosuppression in patients with RCC (Daurkin et al. 2011).
In addition to blocking their recruitment, differentiation, and effector functions, a line of evidence also suggests that some immunological mediators, such as IFN-γ or IFN-α, could also “reprogram” macrophages from immunosuppressive M2 into immunostimulatory M1 cells, thus adding a new feature to macrophage plasticity and new possibilities to TAM targeted anticancer approaches (Duluc et al. 2009; De Palma et al. 2008).
Advance in understanding the biology of TAM offers new possibilities in developing anticancer strategies aimed at targeting these cells or modulating their function, although further investigation of their features in the context of different tumors may be required to optimize their therapeutic manipulation.
Acknowledgments
Alberto Mantovani is supported by the Italian Ministry of Health and by ERC. Maria Rosaria Galdiero is supported by a fellowship from P.O.R. Campania FSE 2007–2013, Project “CREMe”.
References
Ajami B, Bennett JL, Krieger C, Tetzlaff W, Rossi FM (2007) Local self-renewal can sustain CNS microglia maintenance and function throughout adult life. Nat Neurosci 10(12):1538–1543. doi:10.1038/nn2014 PubMedCrossRef
Algars A, Irjala H, Vaittinen S, Huhtinen H, Sundstrom J, Salmi M, Ristamaki R, Jalkanen S (2012) Type and location of tumor-infiltrating macrophages and lymphatic vessels predict survival of colorectal cancer patients. Int J Cancer 131(4):864–873. doi:10.1002/ijc.26457 PubMedCrossRef
Allavena P, Mantovani A (2012) Immunology in the clinic review series; focus on cancer: tumour-associated macrophages: undisputed stars of the inflammatory tumour microenvironment. Clin Exp Immunol 167(2):195–205. doi:10.1111/j.1365-2249.2011.04515.x PubMedCentralPubMedCrossRef
Avraham-Davidi I, Yona S, Grunewald M, Landsman L, Cochain C, Silvestre JS, Mizrahi H, Faroja M, Strauss-Ayali D, Mack M, Jung S, Keshet E (2013) On-site education of VEGF-recruited monocytes improves their performance as angiogenic and arteriogenic accessory cells. J Exp Med 210(12):2611–2625. doi:10.1084/jem.20120690 PubMedCentralPubMedCrossRef
Beatty GL, Chiorean EG, Fishman MP, Saboury B, Teitelbaum UR, Sun W, Huhn RD, Song W, Li D, Sharp LL, Torigian DA, O’Dwyer PJ, Vonderheide RH (2011) CD40 agonists alter tumor stroma and show efficacy against pancreatic carcinoma in mice and humans. Science 331(6024):1612–1616PubMedCentralPubMedCrossRef
Bierie B, Moses HL (2010) Transforming growth factor beta (TGF-beta) and inflammation in cancer. Cytokine Growth Factor Rev 21(1):49–59. doi:10.1016/j.cytogfr.2009.11.008 PubMedCentralPubMedCrossRef
Bingle L, Brown NJ, Lewis CE (2002) The role of tumour-associated macrophages in tumour progression: implications for new anticancer therapies. J Pathol 196(3):254–265. doi:10.1002/path.1027 PubMedCrossRef
Biswas SK, Mantovani A (2010) Macrophage plasticity and interaction with lymphocyte subsets: cancer as a paradigm. Nat Immunol 11(10):889–896. doi:10.1038/ni.1937 PubMedCrossRef
Biswas SK, Mantovani A (2012) Orchestration of metabolism by macrophages. Cell metabolism 15(4):432–437. doi:10.1016/j.cmet.2011.11.013
Biswas SK, Gangi L, Paul S, Schioppa T, Saccani A, Sironi M, Bottazzi B, Doni A, Vincenzo B, Pasqualini F, Vago L, Nebuloni M, Mantovani A, Sica A (2006) A distinct and unique transcriptional program expressed by tumor-associated macrophages (defective NF-kappaB and enhanced IRF-3/STAT1 activation). Blood 107(5):2112–2122PubMedCrossRef
Bohrer LR, Schwertfeger KL (2012) Macrophages promote fibroblast growth factor receptor-driven tumor cell migration and invasion in a CXCR2-dependent manner. Mol Cancer Res 10(10):1294–1305. doi:10.1158/1541-7786.MCR-12-0275 PubMedCentralPubMedCrossRef
Bronte V, Zanovello P (2005) Regulation of immune responses by l-arginine metabolism. Nat Rev Immunol 5(8):641–654. doi:10.1038/nri1668 PubMedCrossRef
Casazza A, Laoui D, Wenes M, Rizzolio S, Bassani N, Mambretti M, Deschoemaeker S, Van Ginderachter JA, Tamagnone L, Mazzone M (2013) Impeding macrophage entry into hypoxic tumor areas by Sema3A/Nrp1 signaling blockade inhibits angiogenesis and restores antitumor immunity. Cancer Cell 24(6):695–709. doi:10.1016/j.ccr.2013.11.007 PubMedCrossRef
Chaturvedi P, Gilkes DM, Takano N, Semenza GL (2014) Hypoxia-inducible factor-dependent signaling between triple-negative breast cancer cells and mesenchymal stem cells promotes macrophage recruitment. Proc Natl Acad Sci U S A 111(20):E2120–E2129. doi:10.1073/pnas.1406655111 PubMedCentralPubMedCrossRef
Chen C, Qu QX, Shen Y, Mu CY, Zhu YB, Zhang XG, Huang JA (2012) Induced expression of B7-H4 on the surface of lung cancer cell by the tumor-associated macrophages: a potential mechanism of immune escape. Cancer Lett 317(1):99–105. doi:10.1016/j.canlet.2011.11.017 PubMedCrossRef
Chen C, Shen Y, Qu QX, Chen XQ, Zhang XG, Huang JA (2013) Induced expression of B7-H3 on the lung cancer cells and macrophages suppresses T-cell mediating anti-tumor immune response. Exp Cell Res 319(1):96–102. doi:10.1016/j.yexcr.2012.09.006 PubMedCrossRef
Chen PC, Cheng HC, Wang J, Wang SW, Tai HC, Lin CW, Tang CH (2014) Prostate cancer-derived CCN3 induces M2 macrophage infiltration and contributes to angiogenesis in prostate cancer microenvironment. Oncotarget 5(6):1595–1608PubMedCentralPubMed
Condeelis J, Pollard JW (2006) Macrophages: obligate partners for tumor cell migration, invasion, and metastasis. Cell 124(2):263–266. doi:10.1016/j.cell.2006.01.007 PubMedCrossRef
Cortez-Retamozo V, Etzrodt M, Newton A, Rauch PJ, Chudnovskiy A, Berger C, Ryan RJ, Iwamoto Y, Marinelli B, Gorbatov R, Forghani R, Novobrantseva TI, Koteliansky V, Figueiredo JL, Chen JW, Anderson DG, Nahrendorf M, Swirski FK, Weissleder R, Pittet MJ (2012) Origins of tumor-associated macrophages and neutrophils. Proc Natl Acad Sci U S A 109(7):2491–2496. doi:10.1073/pnas.1113744109 PubMedCentralPubMedCrossRef
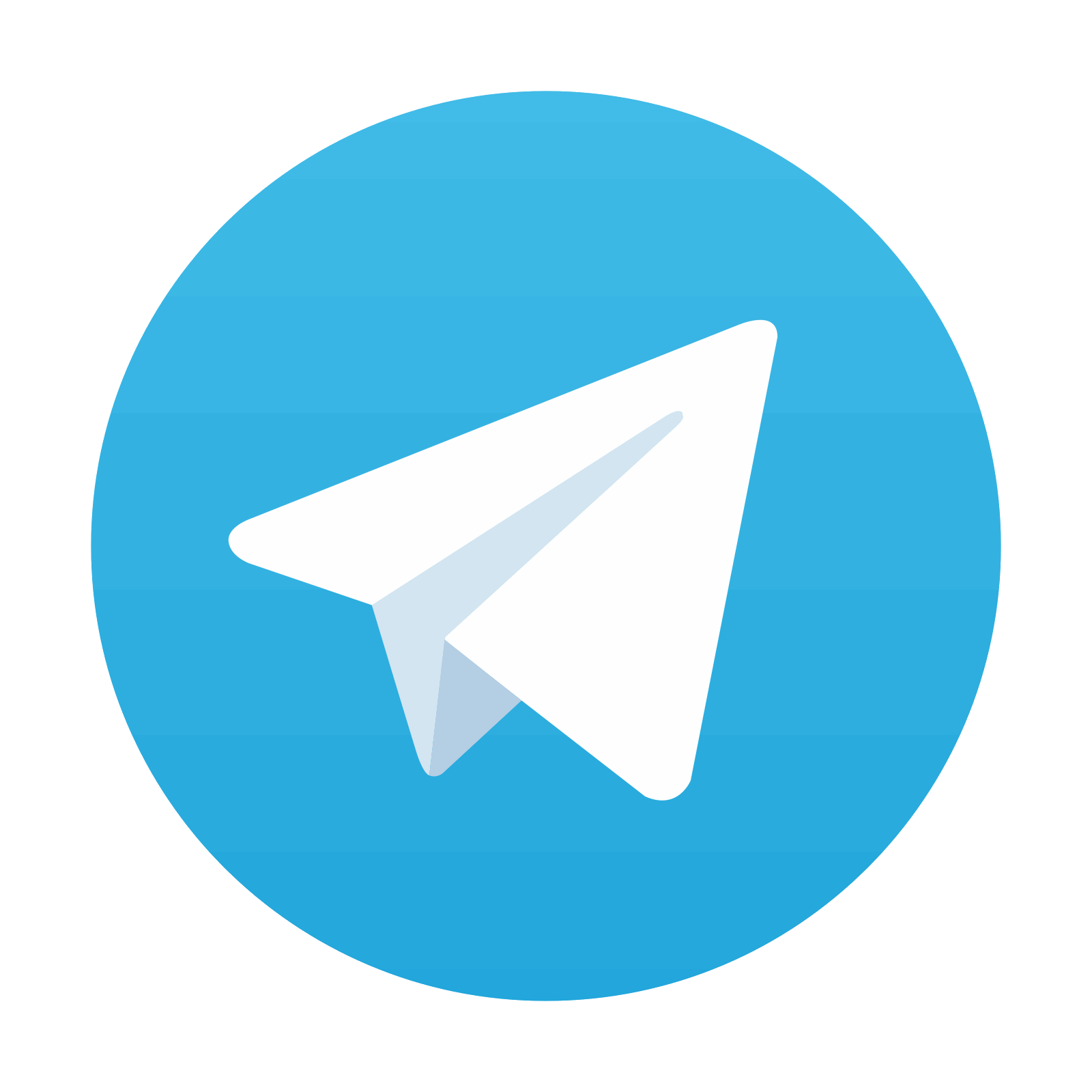
Stay updated, free articles. Join our Telegram channel

Full access? Get Clinical Tree
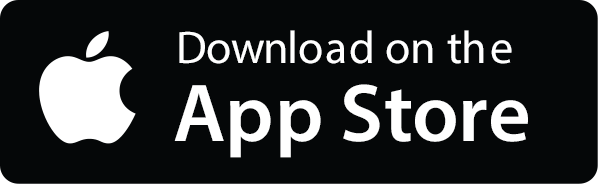
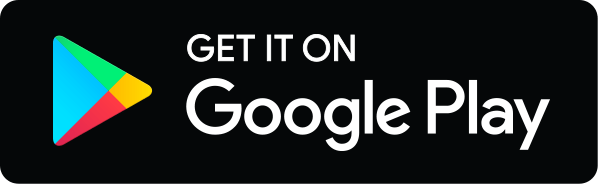