Introduction
Thyrotoxic periodic paralysis (TPP) is considered a neurologic and endocrine emergency. It is a rare, potentially fatal complication of thyrotoxicosis, and is characterized by a variable degree of hypokalemia and muscle paralysis due to a massive shift of potassium into the intracellular space. TPP patients usually seek medical care in the emergency department (ED), but the diagnosis is often missed. The disease has mainly been reported in the male, East Asian population living within East Asian countries. However, published cases from other countries and ethnic groups have increased in the last decade, probably as a result of expanded migration and improved awareness of the condition. A New Zealand study found that Maori Polynesians and Pacific Islanders were also at higher risk for TPP compared to Europeans and European descendants living in New Zealand. Although thyrotoxicosis is more often seen in females than in males, the incidence of TPP is 22 to 76 times more frequent in males compared with females. This chapter will focus on the different features and treatments of TPP, in addition to the novel findings on the molecular mechanisms which underly the disorder.
Clinical Presentation
TPP has been described in the literature since the late 1900s. In 1957, Okinaka et al. published that the incidence of TPP was 1.9% in the thyrotoxic Japanese patient population, and a decade later it was described that 1.8% of Chinese patients with thyrotoxicosis had a history suggestive of TPP. The disorder has also been identified in Asian populations of Thais, Vietnamese, Filipinos, Koreans, Taiwanese, and Malaysians. Cases have been reported in many different populations, including Europeans, Afro-Americans, , Native Americans, , Afro-Caribbeans, Polynesians, , Hispanics, , Brazilians, Nepalese, Lebanese, Turks, , Indians, , and Saudis. The incidence of TPP among thyrotoxic patients in the United States has been estimated to be 0.1% to 0.2%. ,
The typical patient is a 20- to 40-year-old East Asian male. The few reported female cases have had a similar age distribution. , , , , Although it is rare in children and adolescence, some cases of TPP in adolescence have been reported from China, Korea, and in other ethnic groups. Until recently, reported cases of TPP have been attributed to Graves’ disease, although autoimmune thyroiditis has been seen to be a very rare cause of TPP in children and adolescences. The hallmark of TPP is recurrent muscle weakness. The attacks tend to be more commonly seen during the hot summer months, likely due to outdoor activities, loss of potassium through perspiration, and the drinking of cold and sweet drinks. , , It has been reported , , occasionally even on cold winter days. Manoukian et al. reported that the paralysis started between 0100 and 0600 hours in 84% of his patients. The attacks frequently occurred at night or early in the morning upon waking. Others have reported that the attacks tended to occur during weekends, and were often preceded by a heavy meal, high alcohol intake, or strenuous exercise. Other triggering factors that have been described included infections (mainly upper respiratory tract), emotional stress, menstruation, trauma, high-salt diet, beta 2 adrenergic bronchodilators, and glucocorticoid administration. , , Excessive alcohol intake can result in increased catecholamine secretion and hyperinsulinemia and that, along with hyperthyroidism and the hyperadrenergic state, may lead to a low serum potassium. Affram et al. highlighted a case of TPP after an epidural steroid injection for traumatic low back pain in a patient with severe hypokalemia, hyperthyroidism, and Graves’ disease. Corticosteroids have been well-known precipitating factors. The side effect profile of corticosteroids, ranging from the common to the rare, should be kept in mind.
Initial symptoms typically seen on presentation include muscle aches, cramps, and stiffness. Minor muscle aches and weakness accompanied by sore joints can precede the inability to move the legs altogether by approximately 2 weeks. As a rule, the proximal muscles of the lower extremities are initially affected, but in approximately 80%, all four extremities are eventually involved. Symptoms can range in intensity from mild weakness to total paralysis. Asymmetrical weakness has also been noted. Attacks involving all four extremities are frequently linked to urinary retention, oliguria (occasionally polyuria if there is prolonged hypokalemia), and constipation. However, the acute onset of lower motor neuron quadriparesis, without bladder or bowel involvement, has also been described occurring with concomitant hypokalemia and compensated respiratory alkalosis. Improvement in quadriparesis has been achieved with intravenous (IV) potassium, magnesium sulphate, and cautious monitoring.
It is hypothesized that the size of the quadriceps femoris muscle may contribute to TPP. A study by Tang et al. found that the muscle thickness of TPP patients was larger than in nonparalytic hyperthyroid patients. For a hyperthyroid patient, the increase in the quadriceps thickness may indicate a close correlation with TPP, independent of age and body mass index (BMI). Thus, the study concluded that the size of the quadriceps femoris may be, in some way, causally related to the TPP.
Achilles tendon reflexes are absent in about two-thirds of patients, but mental status and sensory functions have been seen to be intact. Ocular, bulbar, and respiratory involvement, although rare, has been documented in a few very severe and occasionally fatal attacks. , Affected muscle groups recover in the reverse order from the paralysis, usually within 36 hours and all within 72 hours. The time from the start of symptoms until the IV potassium treatment is around 3.5 hours in hospitals that have seen many cases of TPP.
In 76% of patients, the thyrotoxicosis had not been diagnosed before the attack, and 79% had no family history of hyperthyroidism. Signs and symptoms of hyperthyroidism were often elusive and were totally absent in 55% of patients. TPP may remain undiagnosed if thyroid function tests are not taken and reviewed in the ED. Occasionally, patients have been subjected to extensive and expensive neurologic investigations and prolonged hospitalization prior to the discovery of TPP. , A key feature to note is that the attacks may recur, but there is always a complete recovery between the episodes of muscle weakness. About half of the patients had suffered at least one attack before the correct diagnosis was made in one hospital where TPP was seen. The delay in diagnosis is, on average, 14 months. Concurrence of potentially treatable TPP and encephalopathy associated with autoimmune thyroid disease (EAATD) as an initial neurologic manifestation of Graves’ disease has been reported. EAATD is a heterogeneous and extremely rare clinical entity that may occur in patients with clinical or subclinical autoimmune thyroid disease. A high level of antithyroid antibodies, increased cerebrospinal fluid protein concentration, nonspecific neurologic symptoms, and psychiatric symptoms with nonspecific electroencephalogram abnormalities are the hallmark of EAATD. High doses of corticosteroids often lead to dramatic improvement in the patients.
Diagnosis
TPP usually occurs when the patient is in a hyperthyroid state, and thyroid function tests, when performed, will lead the clinician to the correct diagnosis. However, attacks after the resolution of hyperthyroidism have been reported. The severity of the attack does not always correspond with the degree of hyperthyroidism, and the thyroid hormone levels are usually lower than in unaffected thyrotoxic patients. This observation suggests that TPP typically occurs in the early stages of thyrotoxicosis and accounts for why the patients display so few thyrotoxic symptoms.
Different etiologies of hyperthyroidism can result in TPP. The majority of patients have Graves’ disease, but a small number of cases have been associated with toxic nodular goiter, , , thyroiditis, , , , solitary toxic thyroid adenoma, , , thyrotropin-secreting pituitary adenoma, iodine-induced thyrotoxicosis, excessive thyroxine replacement, misuse of thyroxine/triiodothyronine , , or triiodothyroacetic acid (tiratricol), and amiodarone-induced thyrotoxicosis. , Soonthornpun et al. reported that 90% of TPP individuals were overweight and 40% had an increased waist circumference that was significantly larger than in unaffected thyrotoxic controls. This increase of BMI in TPP has also been confirmed by others. ,
A correlation between the degree of hypokalemia and severity of the paralysis was found in one study but was absent in another. Serum potassium levels ranged from 1.1 to 3.4 mmol/L and was most often less than 3.0 mmol/L. , , Sporadic cases of lethal and severe ventricular arrhythmias together with hypokalemia have been described. , , In extremely rare cases of TPP, patients with both normokalemia and hyperkalemia have been reported. , , In our experience, however, delayed blood sampling can explain why serum potassium levels in many cases were normal, as the patients had already recovered in the interim.
Due to the intracellular shift of potassium, the urinary potassium excretion rate is low in TPP. A study by Abbas et al. describes a case where all TPP patients demonstrated urinary potassium less than 20 mmol/L. However, long-standing hypokalemia can impair the renal ability to concentrate urine, resulting in polyuria, and make a spot urine collection of potassium unreliable. In these cases, the urinary potassium/creatinine ratio may be used. In TPP the ratio is typically less than 2.0 potassium/mmol creatinine. One case report described an atypical presentation of TPP. The findings included hypokalemia with hyperthyroidism and renal potassium wasting in a 36-year-old Chinese man with no previous history of renal disease. TPP was not initially recognized due to the renal potassium wasting, which does not occur normally in patients with TPP. However, his serum potassium level rebounded rapidly within several hours after potassium supplementation, indicating that the intracellular shifting of potassium ions was the main etiology of his hypokalemia.
Bone turnover is stimulated by thyroid hormones, which increase the glomerular filtration rate and decrease the tubular resorption of calcium, leading to a higher urinary calcium concentration in hyperthyroidism. Calcium is often elevated in Graves’ disease due to high thyroid hormone levels.
An intracellular shift is the probable reason for the transient hypophosphatemia and hypomagnesemia seen in TPP. In the study conducted by Manoukian et al., 80% of the attacks were associated with mild to moderate serum hypophosphatemia (0.36 to 0.77 mmol/L [1.1 to 3.0 mg/dL]; reference range 0.81–1.55 mmol/L) and all demonstrated low or low-normal serum magnesium levels (0.60 to 0.80 mmol/L [1.5 to 1.9 mg/dL]; reference range 0.7–1.2 mmol/L).
TPP patients usually present with a marked decline in urinary phosphate excretion, although there has been considerable overlap with that of normal. However, a spot urine calcium/phosphate ratio of greater than 1.4 mmol/mmol (1.7 mg/mg) indicated TPP with a sensitivity of 100% and a specificity of 96%. A spot urine calcium/phosphate ratio has therefore been suggested as a useful tool when diagnosing TPP in the ED.
Serum alkaline phosphatase levels were mildly elevated in 75% of patients (118 to 268 U/L; reference range 39–117 U/L). Serum creatine phosphate has been found to be elevated in almost 70% of attacks, especially in those precipitated by exercise. The elevation was predominantly mild but in a few cases it was severe.
The acid-base status is generally normal in TPP, although fear, anxiety, and stress can uncommonly give rise to a mild respiratory alkalosis as a result of hyperventilation. A study performed by Sonkar et al. describes a case of acute onset of lower motor neuron quadriparesis with concomitant hypokalemia and compensated respiratory alkalosis. Occasionally a mild respiratory acidosis is seen as an effect of respiratory muscle weakness attributable to severe hypokalemia.
Hypokalemia increases membrane excitability in the Perkinje fibers of the cardiac conducting system. Severe hypokalemia can induce ventricular arrhythmias and delays ventricular repolarization by inhibiting potassium channel activity. While awaiting thyroid function results, electrocardiographic (ECG) changes in patients with TPP can offer supportive clues to the diagnosis and strengthen the clinical suspicion of TPP in the ED.
Pathological ECG is found in 83% to 100% of patients with TPP. , The mechanism of TPP includes increases in Na + /K + ATPase activity stimulated by thyroid hormone and/or hyperadrenergic activity along with hyperinsulinemia. Elevated levels of thyroid hormones usually affect the electrophysiologic system in the heart in addition to the typical ECG changes of hypokalemia (U waves, T wave flattening, ST depression, QT prolongation, widened QRS complexes, and high QRS voltage). Sinus tachycardia is commonly seen. Sinus arrest and second-degree atrioventricular block (AVB) have been noted. Dangerous electrocardiographic abnormalities include AVB, atrial fibrillation, ventricular fibrillation, and asystole. The cause of these conduction disturbances was unknown. Atrial fibrillation and other supraventricular arrhythmias and, more rarely, ventricular arrhythmias have also been reported. , , , Almost 75% of TPP patients demonstrate increased QRS voltage, which is also common in ordinary hyperthyroidism. Other cardiogenic effects that have been reported on the electrocardiogram include sinus tachycardia and marked ST segment depression in leads II, III, aVF, and V4–V6, and QT interval prolongation. Acquired long QT interval often results from hypokalemia, hypomagnesemia, or from medications.
Myopathic changes with reduced amplitude of compound muscle action potentials are generally seen when an electromyogram (EMG) is done during an attack.
Nerve conduction studies have been normal. A prolonged exercise test can reproduce EMG changes seen during paralysis and were shown to be very sensitive and specific for TPP (90% and 70%, respectively). Jiaoting et al. described a long exercise test performed during the interphase time between attacks of paralysis in the absence of muscle weakness. The right hand was chosen for the long exercise test. The compound muscle action potential (CMAP) was recorded from the abductor digit minimi muscle. Results showed a significant difference in the CMAP amplitude decrease rate (CMAPADR) between healthy control subjects (non-TPP patients) and TPP patients, with TPP patients showing a decreased CMAPADR rate significantly greater than a healthy control subject. In the euthyroid state, these abnormalities may not completely improve, indicating a permanent latent abnormal excitability of the muscle membrane.
Differential Diagnosis
Periodic paralysis is classified into primary and secondary, depending on the etiology. Primary periodic paralysis is most often familial. Primary causes include hyperkalemic or hypokalemic periodic paralysis and Andersen syndrome, while TPP remains a secondary cause. TPP is seldom associated with a positive family history and has a late onset of paralytic attack, whereas in Andersen syndrome, primary hyperkalemia and hypokalemia are autosomal dominant and manifest within the first two decades of life. In more than half of cases the cause is a shift of potassium from the extracellular to the intracellular space due to TPP or familial hypokalemic periodic paralysis (FHPP). The two can often be distinguished by the case history and the patient’s hereditary background. The muscle weakness is similar in the two conditions, but thyroid function tests are normal in FHPP. FHPP is an autosomal dominant condition in which a family history of hypokalemic paralysis and the genetic mutations are often well characterized. White people are generally affected with equal sex distribution, and it usually presents before the age of 20 years. In FHPP, acetazolamide and thyroxin usually lessen the frequency of attacks, in contrast to the situation in TPP, where the attacks may become worse. As the treatment is quite different for the different hypokalemic paralyses, accurate diagnosis is essential. In addition to TPP, the differential diagnosis of lower limb paralysis includes: Guillain-Barre syndrome, polymyositis, myasthenia gravis, acute intermittent porphyria, sagittal sinus thrombosis, severe hypokalemia, familial periodic paralysis, and transverse myelitis.
Pathophysiology
Skeletal muscles contain the largest pool of potassium and have an important role in extracellular potassium homeostasis. Exercise will increase the extracellular potassium pool, whereas rest will restore it to normal. The cell membrane sodium/potassium (Na + /K + ) ATPase pump actively transports potassium from the extracellular to the intracellular compartment in muscles and the outward flow is controlled by inward rectifying K + (Kir) channels and delayed rectifying K + channels. Activation of Na + /K + -ATPase exists in many conditions, including thyrotoxicosis. Thyroid hormones increase the activity of the cell membrane Na + /K + -ATPase pump, and thyroid hormone excess can cause hyperstimulation. The Na + /K + -ATPase pump activity in TPP patients is increased by 80% compared to other thyrotoxic patients. However, this is not the cause of hypokalemia in the majority of hyperthyroid patients because the increased activity will in most cases be compensated by an increased outward potassium flow. Only if the outward flux is hampered as well will hypokalemia and subsequent paralysis occur. Gragg et al. described a case of a 26-year-old Black male with normokalemic TPP who developed the syndrome post strenuous exercise. On admission to the ED, he presented with limb paralysis but remained normokalemic on serial testing. This suggests that a single test or even multiple tests showing normokalemia should not rule out TPP.
The Na + /K + -ATPase pump activity in skeletal muscle is intensified by catecholamines and as the β-adrenergic responsiveness is increased in hyperthyroidism, the catecholamines will further enhance the activity. This could potentially be one explanation for the effectiveness of nonselective beta-blockers such as propranolol in TPP. The Na + /K + -ATPase pump is also stimulated by insulin, explaining why many attacks are preceded by meals overloaded with carbohydrates and sweet snacks. Similarly, drugs that impair the insulin sensitivity, i.e., glucocorticoids, can precipitate an attack. Insulin sensitivity has been indicated to be decreased in TPP compared with other hyperthyroid patients. , Glucose infusions should therefore be avoided in TPP, as it would increase insulin secretion, exacerbating the intracellular shift and aggravating the hypokalemia. Propranolol decreases insulin secretion, which could be another reason for its therapeutic effect in TPP. Insulin and catecholamines not only stimulate Na + /K + -ATPase activity but can also inhibit Kir channels.
In animal models, testosterone increases Na + /K + -ATPase pump activity, whereas estrogen and progesterone decrease Na + /K + pump activity. , This might partly explain why TPP almost exclusively affects males. Male TPP patients have higher testosterone levels than thyrotoxic male patients without paralysis. In two TPP males, unilateral adrenal adenomas and hyperandrogenemia were found. TPP has also been associated with testosterone supplementation in a patient with female to male gender reassignment. Studies in animal models of influenza virus–infected lungs have demonstrated that the release of several cytokines stimulates the hypothalamic-pituitary-adrenal axis, leading to increased cortisol and adrenalin production, which activates the Na + /K + -ATPase pump. ,
Genetic Aspects
To date, six mutations of the KCNJ18 gene encoding an inwardly rectifying potassium channel named Kir2.6 have been described in TPP patients. The loss of Kir2.6 ion channel function leads to a decreased outflow of potassium from the intracellular compartments, and the massive influx of potassium in the skeletal muscle cells due to the increased Na + /K + ATPase activity leads to extracellular hypokalemia and episodic weakness. , These changes occur during thyrotoxicosis but not during the euthyroid state. Zhao et al. reported a series of 537 patients with TPP in the Chinese Han population. The authors genotyped over 100 TPP-specific single nucleotide polymorphisms (SNPs) and found three loci that were unequivocally associated with TTP.
Jin et al. published a study that included 127 mainland Chinese patients with TPP, in which 3.1% (4/127) of TPP cases harbored the KCNJ18 gene mutation. No mutations were identified in the KCNJ2 gene. Mutations in the KCNJ18 gene may be an important cause of TPP in some patients; however, not all TPP patients have KCNJ18 gene mutations. KCNJ18 gene mutations were found in 33% patients from Brazil, USA, and France, and in 26% patients from Singapore, whereas it was seen only in 1.2% of patients from Hong Kong, and none from Thailand. In addition, whereas KCNJ18 gene mutations were detected in 1.6% of Taiwanese patients with TTP, it was detected in 3.3% of patients with sporadic periodic paralysis. Other gene mutations and channelopathies may also be responsible for the development of TPP. A genetic variant, 75 kb downstream of the KCNJ2 gene, was associated with TPP in Thai patients with hyperthyroidism ; however, no mutations in the actual the KCNJ2 gene were found. In the Korean population, the genotype and allelic frequency of a single nucleotide polymorphism (SNP;r312691) has shown a significant association with TPP.
Although TPP and FHPP have a similar clinical presentation, there is no common genetic background besides the fact that FHPP is an autosomal dominant disease. The main difference appears to be racial: FHPP usually affects White people, whereas TPP mainly affects East Asian males. FHPP is caused by mutations in the gene encoding the L-type α1 calcium channel subunit (Ca v 1.1; CACN1AS ) in skeletal muscle, leading to ionic channel defects. These mutations could not be routinely demonstrated in Brazilian or Chinese patients with TPP, , , with only two cases being reported. The mutation in the K v 3.4 gene ( KCNE3 ), which encodes for a voltage-gated potassium channel, was found in a Brazilian patient with TPP. Another mutation in the voltage-gated sodium channel, Na v 1.4 ( SCN4A ), was reported in a White family with TPP. Whether these cases represent a true genetic overlap or a random genetic variation is unclear, as in four studies of a total of 251 Asian people affected by TPP, similar mutations were not found. , , , It has been shown, however, that both in FHPP and in TPP, insulin is able to reduce Kir conductance, suggesting similarities in insulin regulation.
Thus our present knowledge indicates that TPP is caused by a mixture of genetic predispositions, the presence of thyrotoxicosis, dietary excesses of various types, and other, yet unknown stimulants. The diagnosis of TPP is still primarily based on the clinical presentation, the presence of hypokalemia, and the demonstration of hyperthyroidism with elevated serum T4, free T3, and suppression of TSH.
Acute Treatment
Guidelines for the management of thyrotoxic hypokalemic periodic paralysis have been updated by Correa et al., who encourage early beta-blockade, with potassium replacement “taking the backseat,” as beta-blockers treat the underlying disease process driving the periodic paralysis. Propranolol, a nonselective beta-blocker, is used for blocking the hyperadrenergic activity seen in the pathogenesis of TPP. The rationale for choosing a nonselective beta-blocker is that a beta-1 selective agent is less likely to inhibit the beta-2 receptor mediated hypokalemic effect of epinephrine. Propranolol should be started as a solitary treatment at a rate of 3.0 mg/kg orally. If the oral route is not tolerated, an intravenous infusion of 1.0 mg every 10 minutes up to a total of three doses can be given. The goal of the currently recommended therapy focuses on the importance of preventing rebound hyperkalemia. If potassium is given, it should be administered as a 5-mEq (1.95 g) oral dose every 2 hours, with a maximum of 90 mEq (3.52 g). The patient’s serum electrolytes should be obtained within 3 hours of potassium administration and frequently thereafter, to minimize the development of hyperkalemia. It has been demonstrated that propranolol IV (1.0 mg IV every 10 minutes for a total of 3 doses) was successful in treating attacks of TPP when potassium chloride (KCl) supplementation was shown to be ineffective. , Falhammar et al. have summarized the need for vigilant monitoring. Fatal arrhythmias have been reported when high doses of potassium have been given (hyperkalemia of greater than 10 mmol/L). With an IV KCl infusion at a rate of 10 mmol/hour, 59% to 70% of patients treated had rebound hyperkalemia of greater than 5.0 mmol/L. , Among those receiving in total 50 mmol or less of KCl IV, few developed hyperkalemia. Most likely this lower dose of KCl is sufficient in the majority of TPP patients, and severe hyperkalemia could thus be avoided. A fatal outcome has been reported with simultaneous administration of IV KCl and IV glucose. As a glucose infusion can further aggravate the hypokalemia, saline is the preferred solution.
Paradoxical hypokalemia, defined as a paradoxical drop in serum potassium level when potassium is given for severe hypokalemia, has been mentioned in the literature as it was reported initially in the TPP patient population. These patients had higher serum free thyroxin concentrations, higher heart rate, and higher systolic blood pressure compared to TPP patients without paradoxical hypokalemia but with similar lead time, age, BMI, and initial potassium concentration. It is postulated that it affects one-quarter of patients. It is quantified as a fall in serum potassium of 0.1 mmol/L or greater during IV KCl. These patients needed more than double the recovery time, and their potassium infusions resulted in more severe hyperkalemia. Potassium supplementation as prophylaxis against further attacks was ineffective, and it was recommended that it should be avoided. Nevertheless, rebound hyperkalemia with cardiac arrhythmias developed, although paralytic symptoms were rapidly reversed. ,
Oral propranolol (40 mg four times a day) also prevented recurrent attacks by inhibiting the Na + /K + -ATPase pump activity. Propranolol, however, must be used with precaution, especially if given IV and/or in cases of heart block, to prevent adverse effects such as severe bradycardia, complete heart block, and cardiac failure. Cardiovascular collapse has been associated with both oral and IV administration of propranolol in thyroid storm. A safer alternative in the emergency situation may be the use of ultra-short-acting beta-blockers, for example, IV esmolol. If thyroid storm is present, methimazole or propylthiouricil should also be administered along with steroids at least 1 hour prior to administration of iodine.
Although some authors advocate propranolol as the first-line treatment in the ED for TPP, , others consider that further studies are needed before this is the definitive treatment strategy. , , The use of propranolol, with its beneficial effects of nonselective beta-blockade, includes the beneficial effects of lysis of the physiologic response to catecholamines and beta-2 mediated insulin release. This places a brake on the Na/K pump and rapidly normalizes potassium levels. It also reduces conversion of T4 to T3 and mitigates associated symptoms. Whether propranolol should be combined with a low dose of KCl is also an unsettled issue.
Definitive Treatment
Curative therapy of the underlying hyperthyroidism is the main treatment option, as TPP, with very few exceptions, does not recur in the euthyroid state. In Graves’ disease, toxic nodular goiter, and solitary toxic thyroid adenoma, antithyroid drugs are usually used until definitive treatment with radioactive iodine or surgery can be done. If radioactive iodine is given, an ablative dose should be used to minimize the risk of relapse. Patients should avoid known precipitating factors of TPP, which include heavy carbohydrate intake, a high-salt diet, alcohol ingestion, and undue exertion, until the thyrotoxicosis condition is under control. It should also be suggested that they continue to use propranolol until a euthyroid status has been achieved. , Treating Graves’ disease with only antithyroid drugs for 12 to 18 months is not a preferred option considering the high risk of relapse of Graves’ after cessation of these drugs. In one study, 56% of patients diagnosed with TPP treated with only antithyroid drugs and beta-blockade had a new attack within 7 months, most probably as a result of an insufficient dose of the drug and/or non-compliance. Based on the American Thyroid Association guidelines, both fixed dose and thyroid weight-adjusted dose calculation can be used in treating hyperthyroidism with radioactive iodine, with the goal of reaching a hypothyroid status. , In China, only the thyroid weight-adjusted radioactive iodine dose is recommended to achieve euthyroid status. ,
Anesthesia Considerations
If possible, surgery should be postponed until the patient reaches a euthyroid state. In case of surgery in emergency situations, the thyrotoxic patient with TPP should be given propranolol pre- and intraoperatively, and if hypokalemic, KCl should be administered. At the present time it is understood that both regional and general anesthesia may be performed safely with no specific induction method. Glucose-containing IV solutions should be avoided, as these can precipitate or worsen an attack due to insulin release stimulating Na + /K + -ATPase activity.
Summary
A decline in the incidence of TPP has been reported from Japan. However, it has become increasingly common in the Western countries not only due to population migration but also because of improved awareness of the condition. Many patients are not correctly diagnosed in the ED, as thyroid function tests may not be readily available and there can be delays in controlling serum potassium levels. Rapid recognition and management of TPP is essential to reduce morbidity and prevent mortality. Emergency treatment includes KCl and/or propranolol; however, the definitive treatment is control and cure of the hyperthyroidism. At the present time we can say novel insights of the pathophysiology and molecular aspects of TPP have been achieved. The present concept is that TPP is a result of a combination of genetic predisposition, thyrotoxicosis, dietary, and other miscellaneous factors. In addition to the known factors, the underlying mechanisms still remain incompletely understood and further investigations are required.
References
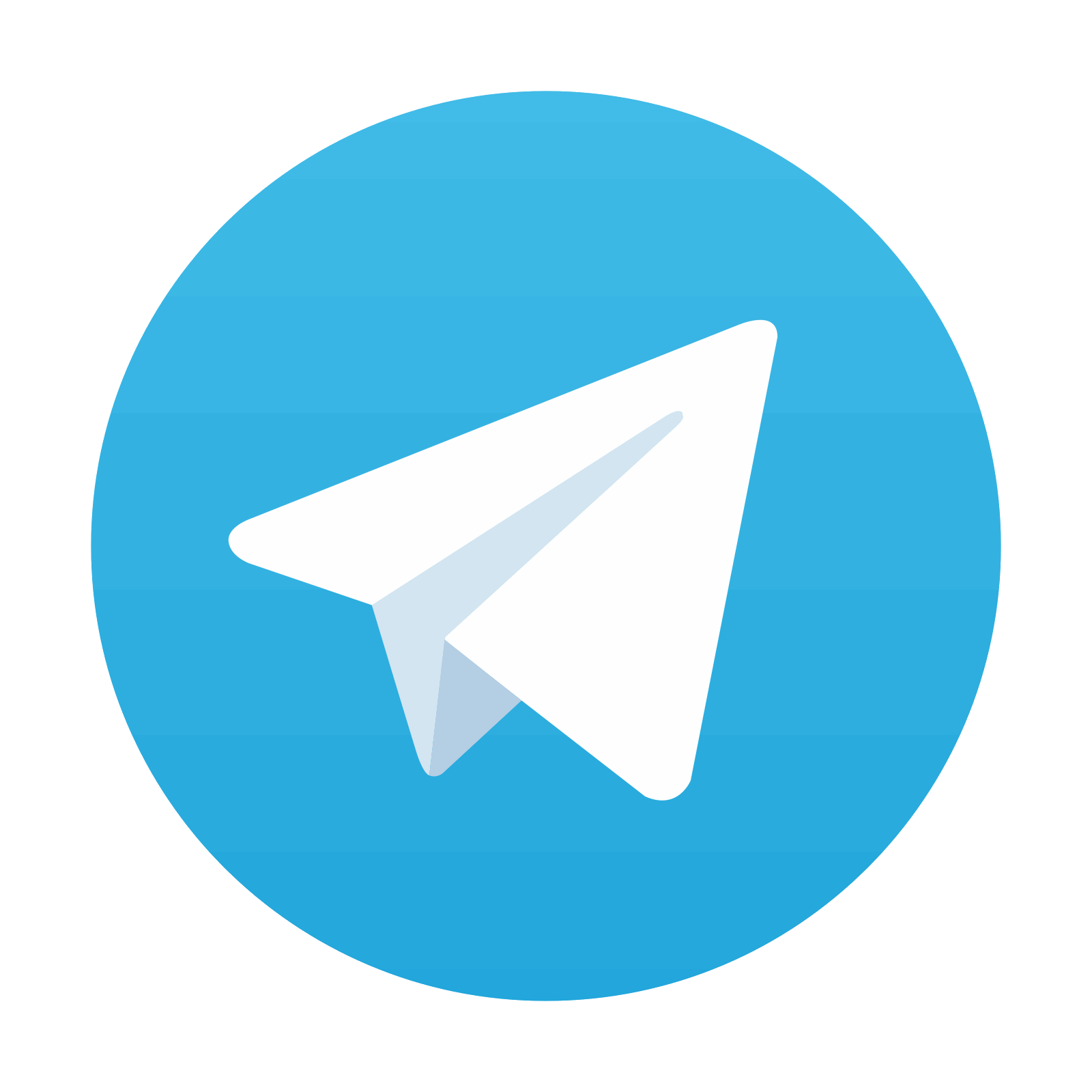
Stay updated, free articles. Join our Telegram channel

Full access? Get Clinical Tree
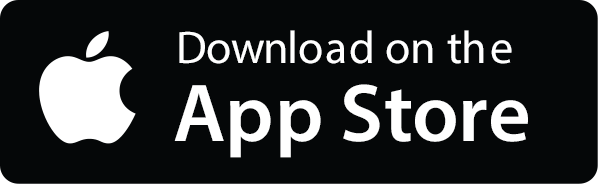
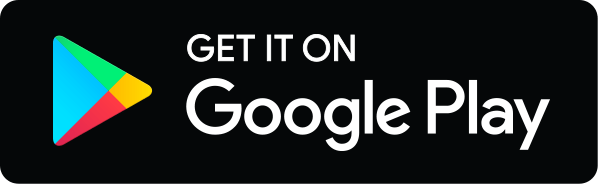
