Developmental stage
Gestational weeks
Thyroid gland organogenesis
Pre-colloid
7–13
Colloid
13–14
Follicular
>14
TRH in hypothalamus
10–12
TSH in anterior pituitary gland and circulation
10–12
TSH receptor in thyroid gland
10–12
Iodide uptake in thyroid gland
10–12
Thyroglobulin synthesis
10–12
Iodinated amino acids
14
Synthesis and secretion of thyroid hormones
16–18
Rise in plasma T3
30
Gene and protein expression of thyroid hormone transporters
7–9
Thyroid hormone receptor binding
10–16
During pregnancy, the pattern of fetal blood levels of T4,T3, and TSH is quite different because T4 and TSH levels progressively rise while T3 remains low until the 30th week of gestation when it begins to increase [19].
At birth, TSH peaks, decreasing thereafter in the first few days of postnatal life while T3 and T4 increase in response to various stimuli, such as increase of cortisol (the most important hormonal trigger in the complex phenomenon of adaptation of life). The role of TH at birth seems, however, to be more supportive than pivotal as demonstrated by the fact that the adaptation of postnatal life of the hypothyroid fetus is normal [20].
2.4 TH Metabolism and Biological Activity
TH levels, however, are not only due to the FHPT axis with negative feedback at pituitary and hypothalamus levels but depend also on the metabolism of TH after their synthesis and secretion. At peripheral level, two enzymatic systems regulate the metabolism of TH in more biologically active (T3) or relatively inactive (rT3) form. The metabolism is regulated firstly by the deiodinase enzyme activity (D1,D2, and D3 deiodinases). It is interesting to note that these three deiodinases which catalyze the deiodination of T4 to T3 could not only act with different kinetics but are also differently expressed in several tissues. For example, the deiodination in liver regulated by D1 seems to be the most important source of T3 in blood [21] while D2 products of catalyzation are mainly responsible for tissue concentrations. The role of D3, as previously discussed, is prominent in the placenta.
T4 is metabolized to less active or inactive forms not only by deiodinase activity but also by sulfation. It has been demonstrated that more or less 80 % of T4 secreted by the thyroid gland could be processed to sulfate inactive metabolites [22]. Sulfation is a reversible chemical reaction by the activity of specific sulfatase enzymes present in several fetal tissues. This reversibility could be very significant in hypothyroidism because sulfate forms of T3 could be transformed into more active T3 reducing potential damage of the brain.
In experimental animals, it has been demonstrated that in hypothyroidism peripheral fetal tissues could modulate the metabolism of TH. Polk et al. [23] showed, for example, that in hypothyroid sheep fetus deiodination ot T4 in liver is reduced due to a less active D1 while in brain D2 activity, which acts on transformation of T4 to T3, is enhanced.
In other words, we should consider concentrations of TH both in blood and in fetal tissues as a result of a complex balance between the synthesis of these hormones, under the control of the FHPT axis, and their metabolism in different tissues. This complex homeostatic system is flexible because, as discussed before, metabolism of TH could be modulated in different fetal tissues on the basis of the specific metabolic condition (like hypothyroidism).
Moreover, the system might change during development. A clear example of this could be the increase of T3 levels during the second trimester and the peak of this hormone seen near the term of pregnancy, due to a change of tissue deiodinase activity.
In order to reach full biological activity determined by the entrance of T3 in the cell nucleus and its binding to nuclear coactivetors, many other metabolic steps are involved [24]. In particular, a normal action of thyroid hormone transporters could be necessary, but our knowledge of the development of these transporters in the fetus is very limited [18]. Also, the expression of the specific TH receptors (THr) and of their different isoforms at various developmental stages need further research. Several evidences show, however, that in a species-specific manner TRr binding capacity varies in different tissues during development [18].
2.5 TH Metabolism and Biological Activity in Brain
It is well known that absence or insufficiency of TH during fetal life causes cerebral damage which could clinically manifest itself postnatally by different grades of cognitive impairment ranging from mild forms to overt cretinism. Therefore, several studies have been addressed to better understand TH action in the brain. Herewith, we summarize some aspects.
TH enter the brain mainly crossing the blood-brain barrier of the choroid plexus transported by specific transporters or indirectly via the blood–CSF barrier. MCT8 seems to be the most important transporter as demonstrated by the fact that the mutation of its gene coding could cause serious developmental impairment in the affected fetus [25].
The transport system seems to involve mainly T4 and contributes to maintain a TH concentration in the brain at about 20 % in comparison to blood levels. TH metabolism is regulated by deiodinase: deiodinase 2 is active in astrocytes where T4 is converted to T3 while deiodinase 3 is expressed in neurons where TH are converted into less biologically active rT3 and T2. The balance between the activities of the deiodinase is a key factor to protect the brain from the detrimental effects of hyper-or hypothyroidism [26].
Next step of TH metabolism is the binding of TH to specific receptors (TR). It has been demonstrated that the two major isoforms of TR, i.e., TRα1 and TRβ1, are expressed at different times during fetal life. TRα1 is detected by 8 weeks while TRβ1 is expressed later; moreover, TRα1 shows a stronger response to T4 than TRβ1. On the basis of several experimental data, it has been recently hypothesized that T4 could act in the brain not only as prohormone, as always suggested, but also as a direct-acting hormone [26].
2.6 TH and Fetal Growth
As underlined at the beginning of this chapter, intrauterine growth is determined by multiple hormonal and nutritional drivers [1]. Hormones stimulating growth might exert an anabolic effect directly on the tissues and modulate the fetal growth according to the nutrients [27].
Among the hormones involved in fetal growth, insulin and insulin-like growth factors (IGFs: IGF-1 and IGF-2) seem to play a pivotal role. In fact, both hypoinsulinemia, due to mutation of insulin promoting factor 1 gene [28], and mutation of IGF-1 and IGF-2 gene are responsible for intrauterine growth retardation [29, 30]. Changes in the IGF system were seen up to the 35th week of gestation in cord serum suggesting that by that time the system was mature in the fetus [31].
Moreover, in our experience [32], IL-6 gene expression and protein content in the placenta were significantly increased in IUGR newborns and were positively related with IGFBP-1 and IGFBP-2 gene expressions, suggesting cytokine and IGF system relationships in the placenta. However, relationships are difficult to demonstrate in observational studies, in particular, when linear statistical methods are being applied to virtually nonlinear relationships; thus, “the system of intra-uterine growth” was further analyzed using biology approaches that identified these as important hubs of the system [33].
A role of TH in regulating fetal growth has been suggested by clinical observations showing that low birth weight could be associated with several thyroid dysfunctions during pregnancy [34, 35]. Moreover, a positive association between FT4 in cord blood at birth and birth weight, birth length, head circumference, and sum of skinfold thicknesses has been recently reported [36].
As demonstrated by several experimental studies, TH act directly on growth regulating the oxidative metabolism of tissues; their blood concentration is positively correlated with the total oxygen fetal consumption, and its action is tissue specific being more evident on fat and skeletal muscle [18]. On the other hand, TH levels could influence fetal growth indirectly by modulation of several hormones and growth factors including expression of IGFs and GH [18].
In experimental animals, it has been recently demonstrated that bone formation but not reabsorption is altered in induced hypothyroidism underlying the important role of TH on skeletal growth [37].
Further information on TH action during fetal life could be derived by studies of intrauterine growth retardation (IUGR). IUGR is primarily due to an abnormal placental development and could be associated both to an increased fetal mortality and to a postnatal impairment of cognitive functions, intelligence quotient, and neurodevelopmental delay [38–40].
Several lines of experimental evidence seem to indicate that abnormalities in thyroid homeostasis could contribute to the etiology of these derangements. A reduction of TH blood levels has been reported in IUGR fetus through cordocentesis [15], and more recently Chan et al. [41] published, for the first time, data on human tissues demonstrating that the transporter MCT8 is less expressed in the central neuro system of IUGR supposing that this abnormality could contribute to the neurodevelopment impairment.
2.7 TH and Fetal Maturation
It is well known that near term, several biological mechanisms are activated for the transition from intra- to extrauterine life. A rise in fetal cortisol concentrations allows the activation of a series of physiological processes involving pulmonary gas exchange, cardiac function, hepatic gluconeogenesis, thermogenesis and is considered the most significant phenomenon.
Cortisol acts on the deiodinase system stimulating T4 deiodinazation to T3 which otherwise play an important role in mediating cortisol maturational effects through different mechanisms which are not yet completely understood and which merit further investigation. The most important actions have been recently reviewed by Forhead and Fowden [18] and can be summarized as follows:
2.7.1 Lung
Cortisol and T3 synergistically induce liquid absorbance in the lung [42] and improve surfactant synthesis and release by type 2 pneumocytes [43].
T3 contributes to upregulation of pulmonary angiotensin-converting enzyme stimulating the fetal renin angiotensin system and therefore acting on the maturation of cardiovascular and renal function.
2.7.2 Heart
On the basis of experimental data in animals both under physiological conditions and after manipulation of thyroid function (thyroidectomy or TH infusion), it has been shown than TH might not only contribute to cardiomycyte growth and differentiation and to the expression of contractile proteins but may also interfere with changes of several receptors for angiotensin II and catecholamines which are of primary importance in cardiovascular adaptation in postpartum life.
2.7.3 Liver
A synergistic activity of cortisol and T3 seems to be necessary to activate hepatic glucogenesis at birth.
2.7.4 Adipose Tissue
At birth, thermogenesis is activated by brown adipose tissue through an uncoupling oxidative metabolism from ATP synthesis in the mitochondria, with the release of heat [44].
This uncoupling is mediated by uncoupling protein 1 (UCP1) level increase in the brown adipose tissue during late gestation in response to a local conversion of T4 to T3 and to induction of UCP1 synthesis in response to the increasing cortisol levels in the fetal plasma as term approaches [20].
2.8 Maternal Thyroid Function and Its Influence on the Fetus
It is well known that overt maternal hyper-and hypothyroidism are associated with increased morbidity both in the mother [45] and in the fetus [46].
It has been estimated that overt hyperthyroidism, mainly due to Grave’s disease, occurs in 0.1–0.4 % of pregnancies [47]. A treatment with antithyroid drugs is recommended [48], and propylthiouracil (PTU) should be the first choice because methimazole (MMI) seems to cause a higher than normal incidence of fetal congenital malformations [49]. On the other hand, liver damage may be associated with PTU treatment [48].
The incidence of overt hypothyroidism is quite similar to that of the hyperthyroidism [50], and the treatment with levothyroxine is recommended [48].
It is interesting, however, to note that overt hypothyroidism is usually defined by elevated TSH and low FT4 serum levels, but according to Canaris et al. [51] it could be difficult to be clinically diagnosed because only about one third of pregnant women diagnosed as overtly hypothyroid on the basis of biochemical analysis were symptomatic.
While overt hyper-and hypothyroidism are relatively rare, a subclinical thyroid derangement seems to be more frequent and may be detected only by some forms of screening (universal or case-finding). How to screen is a matter of discussion mainly because prospective interventional studies are lacking; therefore, the guidelines published by professional associations or scientific societies are different [52, 53].
We point out some examples to better explain why it is not clear which policy should be followed during pregnancy to eliminate or at least minimize the possible damage of the mother and/or fetus.
(1)
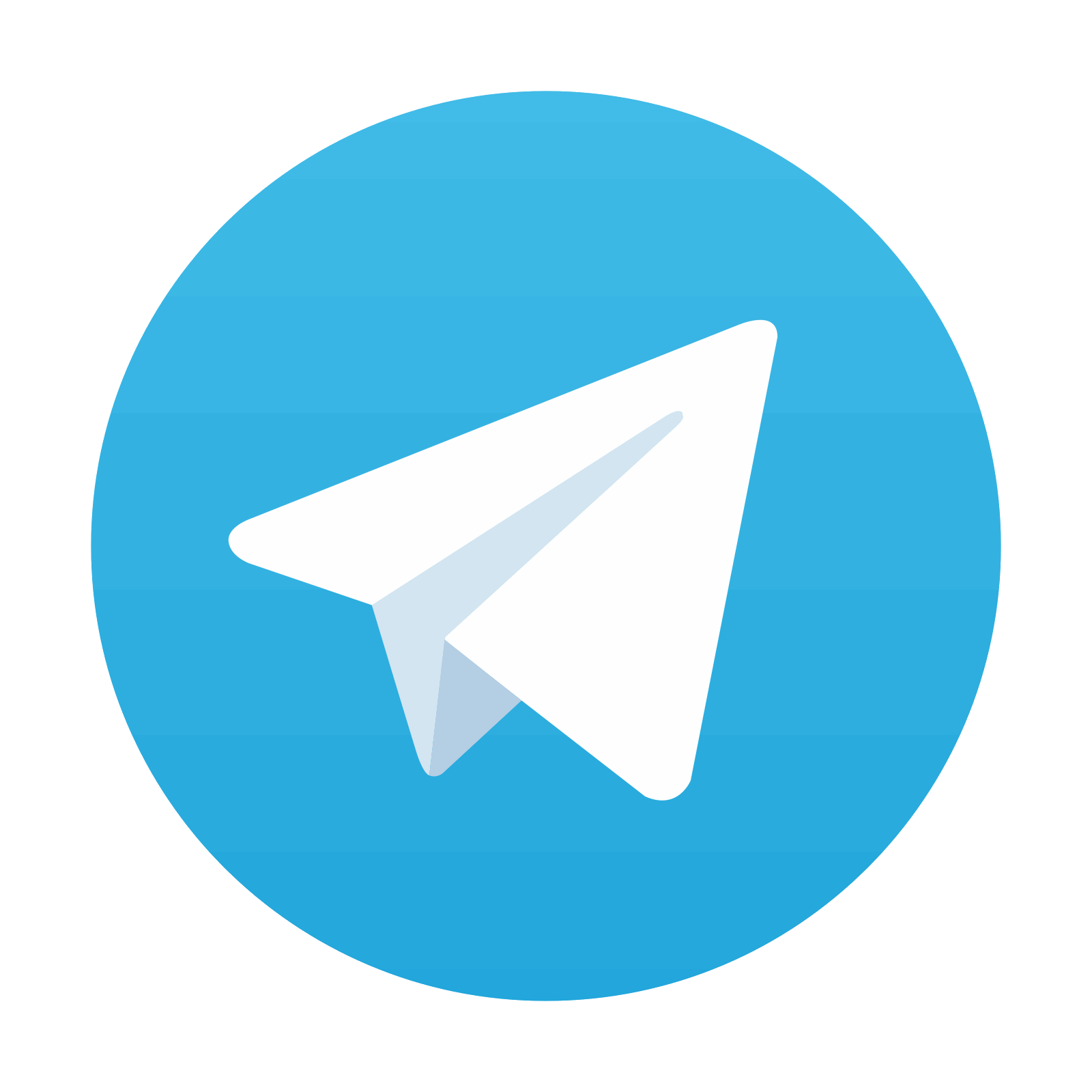
Subclinical hyperthyroidism, defined as low TSH with normal TH levels, has been considered not harmful by some AA [54], while recently, some other AA [55] have found an association between relatively high T4 levels in the early pregnancy and increased incidence of low weight at birth. This discrepancy could be due to several factors, but one of the most meaningful is the difficulty to find a general agreement on the definition of what is low or normal for TH levels.
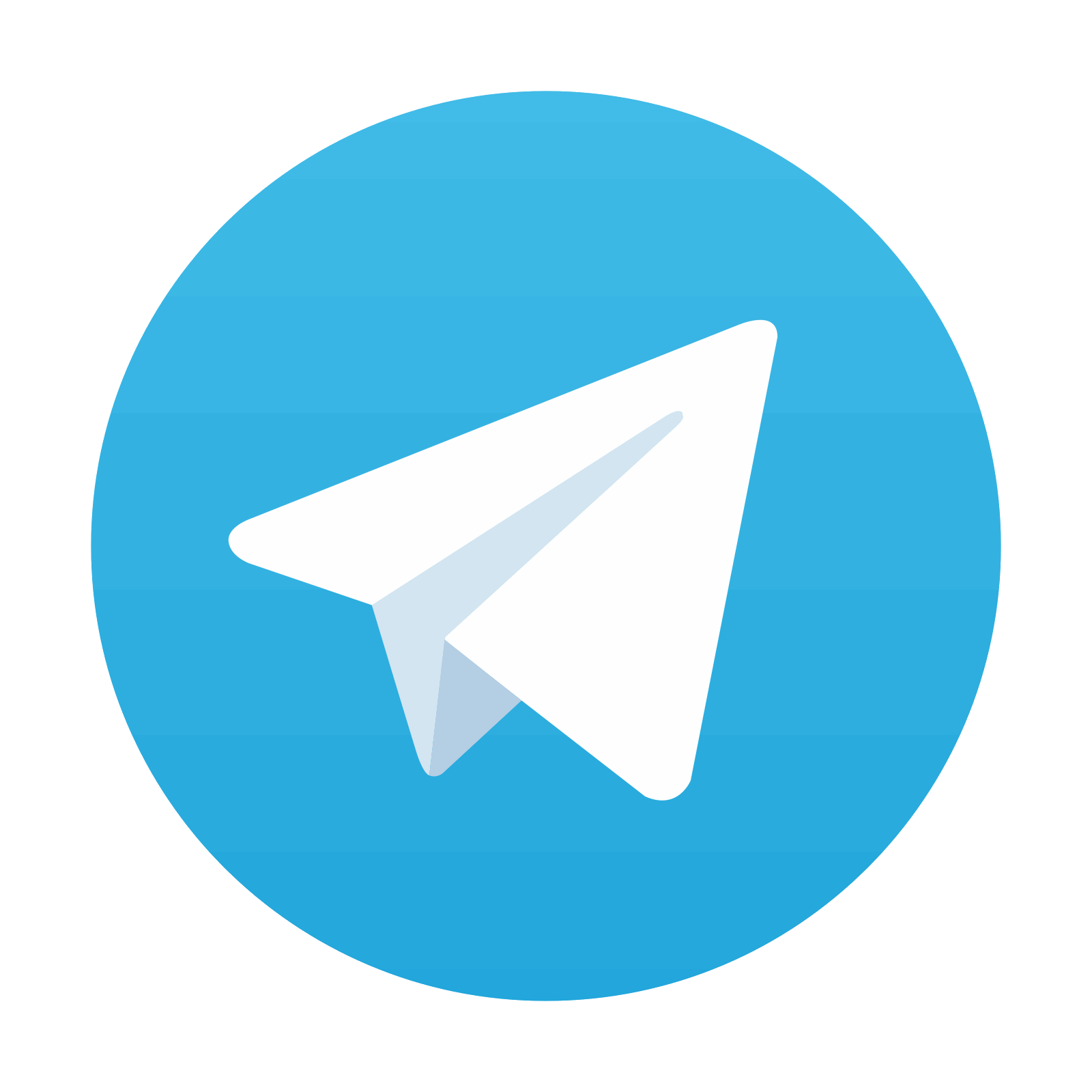
Stay updated, free articles. Join our Telegram channel

Full access? Get Clinical Tree
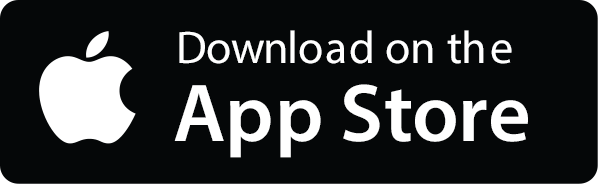
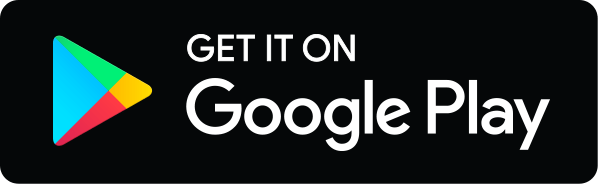
