Fig. 1.1
Early stage of mammalian development. Panel a: Schematic cross-section of a mammalian embryo after gastrulation. The position of three germ layers, ectoderm (brown), mesoderm (orange), and endoderm (green) is indicated. The endoderm is the internal-most layer of the embryo. Panel b: The endoderm lineage. The endoderm generates a primitive gut tube which runs along the anterior-posterior axis of the embryo. The tube is subdivided into the foregut (the most anterior region of the tube), midgut and hindgut. Panel c: Schematic representation of an E10 mouse embryo. The embryonic structures derived from ectoderm, mesoderm and endoderm are indicated in brown, orange and green respectively. At this stage the primordia of organs derived from the foregut are evident as buds (outpockets) from the primitive endodermal tube. thb thyroid bud, tb trachea bud, lb lung bud, hb liver bud, vpb ventral pancreatic bud, dvb dorsal pancreatic bud. A ← → P: anterior-posterior axis of the embryo (Courtesy of Dott. MT De Angelis)
Shortly after formation, the gut tube is formed of a single layer of largely unspecified epithelial-like cells. Subsequently, due to complex phenomena, only partially clarified, the endodermal cells acquire a positional identity along the anterior-posterior axis, and distinct groups of cells can be identified by the expression of specific signaling molecules and transcription factors. Endodermal cells are recruited into specific cell lineages (this initial, still reversible, step of the differentiation program is called specification); as a consequence, the tube, previously monotonous, is patterned in defined domains (endoderm regionalization). In many cases, the first visible event is the appearance of a multilayered structure from which, eventually, organ primordia emerge as outpockets (buds) from the tube (Fig. 1.1b).
As in humans, also in mouse embryos (19.5 day-long gestation), the earliest morphological proof of thyroid specification is the appearance, at E 9–9.5, of an endodermal thickening in the ventral wall of the primitive pharynx (Fig. 1.2). However, a day before this thickening is evident, a group of cells of the pharynx endoderm (thyroid anlage) is distinguishable from any other endodermal cells for the simultaneous expression of the transcription factors Nkx2-1, Pax8, Foxe1, and Hhex [11]. These cells can be defined as precursors of thyroid follicular cells (pTFC), i.e., developing thyroid cells that do not yet synthesize thyroid hormones. It is worth noting that, even if the ultimobranchial bodies participate in the organogenesis of the gland, thyroid hormone-producing cells derive only from the thyroid anlage. This assumption has been proven formally using lineage studies in zebrafish [12] but not yet in mammals where thyroid primordium and UBBs merge in the definitive gland.


Fig. 1.2
Thyroid anlage in mouse embryo. Panel a: Sagittal section of mouse embryo at E9.5. Precursors of Thyroid follicular cells are specifically stained with anti Pax8 antibody. Thyroid anlage (arrow head) appears as a restricted area of the endodermal epithelium of the pharynx floor (ph). Panel b: Enlargement of the same section (From: Missero et al. [44])
The prime mover responsible for the specification of the thyroid anlage in mice is still rather obscure. However, data obtained in other organisms such as zebrafish and chicks have been offering valuable information to extend our knowledge of early steps of thyroid development in mammals.
The development of some endoderm-derived organs (liver, pancreas, lungs) requires inductive signals from the surrounding mesoderm. In the case of the thyroid, we know that in the mouse by E8.5, the thyroid anlage is very close to the aortic sac, from which embryonic heart outflow and pharyngeal arteries originate [13]. The developing thyroid could be targeted by signals coming from the cardiac mesoderm or endothelial lining of the aortic sac. This hypothesis has received strong support by studies in zebrafish [14]. It has been demonstrated that early thyroid development absolutely requires both the expression of the transcription factor Hand2 in the cardiac mesoderm surrounding the thyroid anlage and fibroblast growth factor (FGF)-signals. In humans, a cross-talk between the developing thyroid and heart is supported by epidemiological studies. In fact, a number of patients affected by thyroid dysgenesis also present cardiac malformations at birth [15]; furthermore, DiGeorge syndrome includes both congenital heart defects and an increased risk of congenital hypothyroidism [16].
1.3.2 Translocation and Lobe Formation
In mouse embryos, by E9.5 the thyroid anlage expands and then forms a bud that moves caudally in the surrounding mesenchyme. In the early phase of morphogenesis, the proliferation rate of pTFC is lower than that of the other endodermal cells [13]. Hence, enlargement of the developing thyroid probably depends on the recruitment of other endodermal cells of the primitive pharynx into the thyroid anlage.
The migration of pTFC is a notable feature of thyroid organogenesis. Morphogenesis of the neck encompasses a number of events that can contribute to the translocation of pTFC in the direction of the trachea. However, translocation mostly involves active migration of the precursor cells, which probably move through a process of “collective cell migration” by which cells move in concert without completely disrupting their cell-cell contacts [17]. The genetic basis of the thyroid bud migration has been, at least in part, elucidated; the presence of transcription factor Foxe1 in pTFC is required for thyroid bud migration [11, 18].
At E11.5, after the thyroid primordium is no longer connected to the pharynx, the process of lobulation begins, attested by a lateral enlargement of the developing thyroid; one day later, thyroid appears as an elongated structure close to third pharyngeal arch arteries that will participate in the formation of the definitive carotid vessels. These reports suggest that inductive signals originating from adjacent vessels could be relevant in the lobulation of the gland. This hypothesis was validated using genetically modified mice deprived of sonic hedgehog [19] (a morphogen relevant in vertebrate organogenesis) or Tbx1 [16] (a transcription factor regulated by sonic hedgehog itself). In these models, the development of vessels is impaired, and the thyroid bud does not stay in contact with them. At the same time, the lobulation process is disturbed, and the gland does not separate into two distinct lobes but appears as a single mass. However, in addition to extrinsic signals, pTFC specific mechanisms are required for correct lobulation. In fact, in mice partially deficient for both the transcription factors Nkx2-1 and Pax8, thyroid hemiagenesis is frequently observed [20].
1.3.3 Functional Differentiation and Enlargement
By E14, precursors of TFCs proliferate and begin to undergo a differentiation program completed at E16.5, when the thyroid is composed by bona fide adult TCFs, able to synthesize thyroid hormones. TCFs express, according to a precise temporal pattern, a number of specific proteins such as thyroglobulin (Tg), TSH receptor (Tshr), thyroid peroxidase (TPO), sodium/iodide symporter (NIS), thyroid NADPH oxidases (Duox’s), and pendrin (PDS). At the same time, the gland accomplishes the proper follicular organization required to guarantee a correct hormone supply to the organism.
In the postnatal thyroid, mechanisms triggered by TSH upon its interaction with Tshr are extremely relevant in the regulation of both proliferation and function of TFCs. The finding that in the mouse Tshr is detectable in thyroid cells by E14 and TSH by E15 could suggest a role for TSH/Tshr signaling in the control of functional differentiation and proliferation of TFC in the developing thyroid. However, in mouse embryos lacking a functional Tshr, the size of the fetal thyroid is quite similar to that in wild-type mice [21]. In addition, the number of proliferating cells in the thyroid is comparable in mutants and wild-type embryos. On the contrary, adult mice lacking TSH signaling have a clearly hypoplastic thyroid. These data indicate that in the mouse, TSH/Tshr signaling does not have a relevant effect on the proliferation of thyroid cells during embryonic life [22]. This scenario could be different in humans. In fact, in the mouse, thyroid organogenesis is complete just at the end of gestation, and the functions of the hypothalamic-pituitary-thyroid axis are accomplished only after birth. In humans,, the thyroid is realized by 12–13 weeks; during the rest of gestation, the thyroid continues to grow and the hypothalamic-pituitary-thyroid axis is functioning. While the role of Tshr/TSH signals in controlling the growth of fetal thyroid remain rather obscure, molecular analysis reveals that both TPO and NIS are almost absent in mouse embryos lacking a functional Tshr. Thus, TSH induced signals are required to express key molecules for hormone biosynthesis and to complete the differentiation program of the thyroid follicular cells [21].
Finally, TSH signaling is not required for folliculogenesis because normal thyroid follicles are present in mouse embryos lacking a functional Tshr. Signals from endothelial cells are relevant in forming follicles. In fact, in animal models characterized by a thyroid-specific severe reduction of vascular density, the gland appears as a multilayer mass of nonpolarized cells [23].
1.3.4 Merging of Ultimobranchial Bodies
In placental mammals, the ultimobranchial bodies are embryonic transient structures derived from the fourth pharyngeal pouch, which will merge with the developing thyroid.
In mice by E10, the fourth pharyngeal pouches are detectable as lateral extroflexions of the primitive foregut expressing the transcription factor Islet1 [24]. One day later, the caudal portion of the pouches grows forming UBB primordia identified for the expression of the factors Hes-1 [25], Isl1, and Nkx2-1 [26]. UBBs begin to migrate and reach the primordium of the thyroid at E13. By E14.5, UBB cells begin to disperse within the thyroid parenchyma, and only remnants of UBBs can be distinguished in the thyroid gland.
The expression of Nkx2-1 is required for the survival of UBBs [26], while Hes-1 plays a role in the merging of these structures into the thyroid [25]. UBB defects have been also described in animal models with an impaired expression of Hox3 genes, encoding factors involved in the morphogenesis of several structures. In these models, UBBs do not merge into the thyroid but remain as bilateral vesicles composed exclusively of calcitonin-producing cells (persistent ultimobranchial bodies) [27].
1.4 Thyroid Toolkit Genes
The generation of an increasing number of mouse models carrying mutations on demand of specific loci has been offering biomedical research an invaluable tool for the identification of the functions of proteins in vivo and the dissection of the pathways underlying developmental processes. Also in the case of the thyroid, a large part of our knowledge of molecular mechanisms of its morphogenesis is due to studies of phenotype mice, in which genes encoding proteins expressed during thyroid development have been inactivated by gene targeting (knockout mice).
Molecular genetics of thyroid development was inaugurated by the discovery of a number of transcription factors expressed in the thyroid anlage, Nkx2-1 (formerly called TTF-1), Foxe1 (formerly called TTF-2), Pax8, and Hhex. These genes, which can be considered thyroid “toolkit genes,” remain expressed in both precursor and differentiated thyroid follicular cells. Although these factors are also expressed in other tissues, the coexpression of all four is a peculiar hallmark of thyroid cells [11].
In the next paragraphs, the focus will be on the role of these genes as deduced by their distribution and by the phenotype of knockout animals; the consequences of mutations in these genes in the pathogenesis of thyroid diseases will be exhaustively treated in later chapters.
1.4.1 Nkx2-1
During embryonic life, Nkx2-1 is expressed in the developing brain, lungs, and thyroid. In the brain, the factor is present in some areas of the developing diencephalon, such as the hypothalamic areas and the infundibulum, and in the neurohypophysis [28]. As for the lungs, Nkx2-1 is detectable in the epithelial cells of the developing trachea and lungs. In the thyroid, it is expressed in both precursor and differentiated TFC, in the C cells [26], and in the epithelial cells of the fourth pharyngeal pouches forming the ultimobranchial body. In human embryos, the expression pattern of Nkx2-1 is not different from that of mice [29]. The phenotype of Nkx2–1 knockout mice reflects the expression domain of this gene: severe defects in lung and forebrain morphogenesis, lack of pituitary and thyroid [30]. Notably, in absence of Nkx2-1, the thyroid anlage forms and buds, but already by E10 the thyroid primordium appears smaller than in wild-type embryos and soon undergoes degeneration. The ultimobranchial bodies undergo the same process and disappear by E12 [26]. Thus, Nkx2-1 is required not for the initial specification of both follicular and parafollicular thyroid cells but for the survival of these cells. In addition, Nkx2-1 is also involved in the folliculogenesis. In fact, in mice in which this gene is made inactive by the middle of organogenesis, the postnatal thyroid shows a reduced number of dilated follicles [31].
1.4.2 Pax8
During embryonic life, Pax8 is expressed since E8.5 in the kidneys, brain, and thyroid primordium [32]. In the developing kidney, at an early stage, Pax8 is expressed in the nephrogenic cord and in mesonephric tubules; then, it is present in the cortex of the metanephros. In the brain, Pax8 is transiently expressed in the myelecephalon, through the entire length of the neural tube, otic vesicle, and at the midbrain-hindbrain boundary. In the thyroid, Pax8 is expressed in the thyroid follicular cells and in their precursors. In Pax8 knockout embryos, the thyroid primordium forms, buds from the gut, and begins its migration [33]. However, the thyroid primordium is almost undetectable by E12. In newborn mice, the thyroid lacks thyroid follicular cells and is composed almost completely of calcitonin-producing C cells. The animals are affected by a severe hypothyroidism and die within 2–3 weeks after birth. In mice in which the Pax8 gene is inactivated in the late fetal life, the thyroid shows a severe hypoplasia and reduced expression of genes required for hormone biosynthesis. Thus, Pax8 is required for the survival and the differentiation of thyroid follicular cells.
1.4.3 Foxe1
At variance with Nkx2-1 and Pax8, whose presence in the gut is restricted to the thyroid anlage, during development Foxe1 is expressed in tissues which are developed from the pharynx and pharyngeal arches: thyroid, tongue, epiglottis, palate, choanae, and esophagus [34]. In addition, Foxe1 is also detected in the whiskers and hair follicles, which derive from the ectoderm [35].
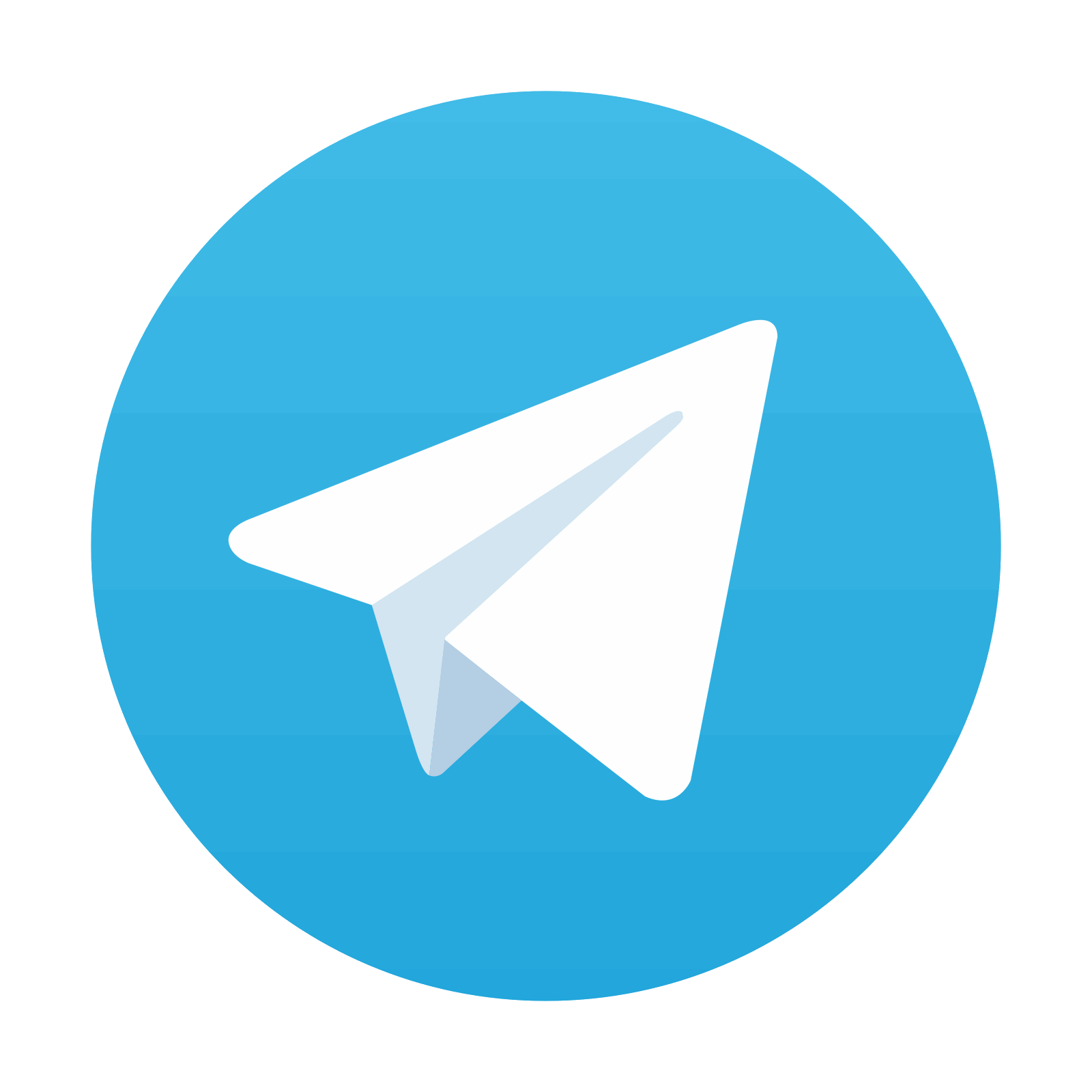
Stay updated, free articles. Join our Telegram channel

Full access? Get Clinical Tree
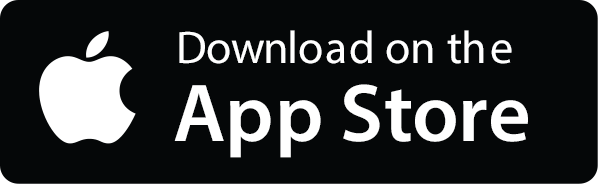
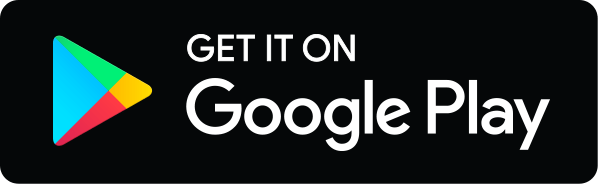