12. Thermal injury
Lynne D. Hubbard
LEARNING OBJECTIVES
By the end of this chapter the reader will be able to:
• Understand the mechanism of injury that leads to the hypermetabolic response and the role of nutritional support in patient recovery
• Assess the nutritional requirements for energy, protein, vitamins, minerals and trace elements and decide on the best way to deliver these nutrients to the patient
• Monitor the effectiveness of nutritional intervention
• Recognise the dietitians role in the multidisciplinary team
• Help support and motivate the patient throughout their recovery
Introduction
The UK national burn care review in 2001 found that every year 250,000 people sustain a burn injury, 175,000 of them visit an accident and emergency department, 16,100 of those are admitted to hospital, and of these 10,200 require care in a specialist burns unit. 1 The review stated that 90% of burn injuries are preventable and a prevention strategy remains at the heart of planning in the UK. Survival has improved in the past 25 years but there are still 300 deaths per year. The majority of deaths are in people over the age of 60 years, in which there has been little improvement in survival, probably due to pre-existing medical conditions. A team approach is vital at every stage of treatment from emergency care to rehabilitation and nutritional support has played a crucial role in improving mortality and morbidity by:
• Trying to ameliorate catabolism and loss of skeletal and visceral muscle;
• Trying to understand and ameliorate hypermetabolism;
• Supporting the immune system;
• Providing the correct balance of nutrients for wound healing; and
• Providing an appropriate balance of nutrients during the anabolic phase of recovery.
The burns team sets its goals high and the national burn care review states the goal of treatment is to ‘recover the individual to the pre-injury state and for them to return to their place in society with unaltered potential’. To try and achieve this goal the dietitian not only plans the dietary prescription but as part of the team plays a crucial role in motivating, supporting and encouraging the patient to help them in their road to recovery.
The structure and function of skin
Skin is the largest organ in the body comprising 15% of body weight. It has a number of key functions: 2
• As a sensory organ for touch, pain, temperature and pressure;
• Regulation of body temperature through vasodilation and vasoconstriction of the microcirculation in the dermis and via the sweat glands; and
• As a barrier to micro-organisms from the external environment entering the body.
Histologically it is made up of two layers: 3
• The epidermis—this outer layer of dead cells is avascular and relatively impermeable. It is produced by cells dividing at the basement membrane and migrating to the outer layer.
• The dermis—this inner layer is vascular and contains nerve fibres, hair follicles and sebaceous glands in connective tissue, composed of collagen, elastin and ground substance. Beneath this is a layer of fat.
The pathophysiology of thermal injury
Burn injury is described in relation to which layer of skin is damaged:
• A superficial burn, e.g. a sunburn involves some damage to the epidermis;
• A partial thickness burn, e.g. a scald involves destruction of the epidermis and part of the dermis;
• Deep dermal; e.g. a scald of longer duration in which more of the epidermis and dermis is destroyed including hair follicles and sweat glands; and
• Full thickness, e.g. flame burn includes the destruction of the epidermis, dermis and basement membrane. Because blood vessels and nerve endings are destroyed there is no pain.
During the first 3–6 hours and lasting for up to 36 hours the microcirculation becomes hyper-permeable. 4 The increased permeability means larger molecules such as albumin and other plasma proteins leak out into the extravascular space, producing tissue oedema and inflammation. The aim of the inflammatory response is to dilute, destroy or wall off the cause of injury, e.g. damaged cells and invading bacteria, and stimulate a series of events resulting in healing the damaged area. A variety of endogenous vasoactive mediators are thought to be involved in the development of wound oedema, including histamine, serotonin, bradykinin, catecholamines and products of arachidonic acid metabolism. The injury of cells stimulates tissue macrophages, monocytes, keratinocytes and fibroblasts to produce peptides called cytokines, particularly interleukin 1 and 6. 5 These are important in:
• Activation and increased production of neutrophils;
• Acting on the hypothalamus to produce fever; and
• Acting on the liver to reset it to synthesise acute phase proteins, e.g. C-reactive protein.
However, the activation of the macrophage-monocyte system which ensures phagocytosis produces unstable oxygen free radicals which produce lipid peroxidation. This leads to secondary damage of the cell membrane and where injury is great, this can result in the systemic inflammatory response. 4 The role of antioxidants in the body, in decreasing the damage done by oxygen free radicals, may be of vital importance (see Trace Elements below).
Metabolic response to injury
The resting energy expenditure falls during the period of fluid resuscitation, due to hypothermia, decreased cardiac output and a decrease in delivery of oxygen to the tissues, a period referred to as the ebb phase. As fluid resuscitation restores circulating volume and delivery of oxygen to tissues, the body enters the flow phase, a prolonged period of hypermetabolism. This is driven by an increase in the catabolic hormones, adrenalin and noradrenalin (catecholamines), cortisol (glucocorticoid) and glucagon. 6 The burn injury necessitates the mobilisation of large amounts of substrate from fat stores, glycogen and muscle for energy and repair. The catabolic hormones stimulate mobilisation of fat reserves, protein breakdown, glycogenolysis and gluconeogenesis. Glycogen reserves are utilised in less than 24 hours; therefore, skeletal muscle is converted to glucose via gluconeogenesis.
The effect of modern treatment on hypermetabolism and recovery
By understanding the pathogenesis of burn injury it has been possible to reduce energy expenditure:
• Occlusive dressings—Fluid is lost through the burn-injured area by evaporation. Combined with plasma exudates this can reach 300 mL/m²/hour or 7 L per day at an energy cost of 580 kcal/L. The use of occlusive dressings can reduce evaporative losses by 50% and with it the energy expenditure of evaporation is reduced. 7
• Temperature regulation—Because cytokines act on the hypothalamus to raise the internal body temperature, by controlling the environmental temperature to 28–32°C. Arturson found patients decreased their rate of heat production and dry heat loss, thus conserving energy. 8
• Pain control—Pain may be caused directly from the burn injury or during dressing changes and physiotherapy. Adequate pain medication reduces anxiety and energy expenditure. 9
• Early excision and grafting—While this does not decrease hypermetabolism, it does attenuate muscle catabolism when compared to delay in surgery and result in reduced sepsis and wound colonisation. 10
• The use of beta-blockers—Herndon used propanolol to block beta-adrenergic stimulation in children with burns of greater than 40%, resulting in a reduction in tachycardia, supraphysiologic thermogenesis, cardiac work and resting energy expenditure. 11 It was also found to increase protein synthesis with a reduction in protein catabolism. Potential risks include hypoperfusion from decreased cardiac output, decrease in blood pressure and bronchospasm.
In addition:
Global assessment and monitoring
In order to decide on an individual patient’s requirements a thorough assessment of nutritional status, medical history and injury history must be made on admission (see Table 12.1). Each unit needs to establish a protocol for biochemical and haematological monitoring. Table 12.2 gives a guide to monitoring, which may be increased in a critically ill patient or decreased in the later stages of recovery. Monitoring of progress should include:
Medical history | Diabetes, renal impairment, alcoholism, gastric ulcers, epilepsy, previous diarrhoea or vomiting |
Social history | Living alone, low income, elderly, physical disability, mental health problems, ethnicity, religious beliefs |
Diet and weight history | Height, dry weight, BMI, physical signs of weight loss, food preferences, vegetarian, dentition, allergies, history of normal intake |
History of injury | Where, when, mechanism (flame, chemical, scald, electrical), non-accidental, suicide |
Total body surface area burn | Size of burn, depth and exact area plotted on a Lund-Browder chart. Note if face, hands or feet are included |
Test | Frequency |
---|---|
Urea, electrolytes and creatinine | Daily for first week |
Liver function tests | Twice weekly |
Serum phosphate, magnesium and calcium | Weekly |
Serum glucose | Daily for first week |
Serum albumin | Twice weekly |
Haemoglobin | Twice weekly |
White cell count | Twice weekly |
Trace elements (copper, zinc, selenium) | On admission and weekly for first month in burns > 20% |
24-hour urinary collection | Twice weekly in burns > 20% |
• Weekly weights;
• Detailed description of the healing of the burn wound, the success of skin grafting and healing of donor sites;
• Information on infection and any other medical problems;
• Daily food and fluid charts;
• Bowel activity; and
• Discussion by the multidisciplinary team of the physical, social and psychological progress.
Calculating energy requirements
Whatever method of calculation is used, a useful guide is that energy expenditure does not exceed twice basic metabolic rate. 14,15 Using indirect calorimetry to measure energy expenditure is ideal but the cost of the equipment, the time to take the measurements, the skills required to take accurate measurements and the potential inaccuracies in some of the sickest patients means that currently this is not an available option in the UK in non-research settings. 16 This is problematic as it is recognised that in critically ill patients overfeeding can cause hyperglycaemia, fatty infiltration of the liver and increased production of carbon dioxide while underfeeding can affect immunocompetence, wound healing and mortality. 17,18
Numerous predictive equations exist for calculating energy expenditure in burns patients using either predicted BMR added to a predicted stress factor or stepwise multiple regression. However, the physiological response of people with burn injury varies according to age, sex, genetics and previous medical condition, meaning:
• The peak of the hypermetabolic response varies as does the length of time it continues, which can be up to 42 days. 13
• Body weight error can easily be introduced into a calculation, as fluid resuscitation may have begun before admission or it may be difficult to weigh the patient on ITU.
• There is no agreement on the use of body weight, body surface area, surface area of burn or basic metabolic rate in different calculations.
Currently the British Dietetic Association Burns Interest Group recommends the use of the Schofield formula based on BMR plus an added stress factor related to the size of the burn. 21 However, this introduces potential error from body weight and estimated stress factor. As much care as possible should be taken in establishing the patient’s dry weight at the time of injury. Taylor has suggested the use of the Toronto formula, developed from multiple regression analysis, for use in ventilated or spontaneously breathing adults. 13,22 Total energy expenditure is calculated as 120% of REE.

However, this is less user friendly than the Schofield equation and needs further evaluation to establish benefit. With the move towards larger and fewer burns centres in the UK, the purchase of equipment enabling the use of indirect calorimetry should be given a high priority if overfeeding or underfeeding is to be avoided. 1
Protein requirements
The increased production of cortisol stimulates muscle breakdown and protein oxidation, resulting in an increased level of circulating amino acids. It is thought these are required for:
• Wound repair;
• The production of acute phase proteins;
• Cellular immunity; and
• Glucose production.
Increased production of glucagon drives gluconeogenesis as protein is broken down into amino acids in the liver. 23
The burn injury therefore increases the requirement and changes the body’s priorities for the type of protein that needs to be produced. At the same time protein losses increase through the wound as plasma exudate and through the urine as urea.
Research continues over the amount and type of protein that can meet the patient’s requirements, reduce skeletal breakdown, maximise wound healing and the immune response. Currently the following is known:
• Although studies have shown no reduction in net protein catabolism above 0.23 g nitrogen per kilogram, 24 it seems likely that patients with large burn injuries still benefit from high protein intakes in relation to wound healing. Gore infused a radioisotope of phenyl alanine into 10 patients with burns over 40% and used it to measure the rate of protein synthesis in the wound, muscle and skin. 25 It was found that there was a net consumption of phenyl alanine by the wound but net release by the muscle. Compared with the skin the wound had a much greater rate of protein synthesis and reduced rate of protein breakdown.
• Alexander found that supplementation of protein (0.4–0.64 g nitrogen/kg, in an feed of 80 kcal to 1 g nitrogen) in severely burned children resulted in a statistically significant increased survival, opsonic index, serum total protein, C3, immunoglobulin G, nitrogen balance and decreased number of infectious episodes. 26
• The infusion of glucose or glucose and amino acids does not reverse gluconeogenesis and nitrogen loss. 27 However, Gore looked at the effects of infusing glucose and insulin on the peripheral amino acid metabolism. 28 Isotopic tracers of phenyl alanine and alanine were used to monitor metabolic changes. When glucose was infused without insulin the result was increased efflux of amino acids. When insulin was infused there was an increased uptake of phenyl alanine into the leg. The conclusions were that hyperglycaemia increased muscle catabolism while the supply of exogenous insulin producing euglycaemia impedes muscle breakdown.
• Catabolism is driven by catecholamines and peaks around day 5–8 but muscle breakdown continues long after this. This may be due to prolonged bed rest in the burn-injured patient, which continues to cause amino acid efflux from muscle. 29
• Early excision and grafting and aggressive enteral feeding, while not decreasing energy expenditure, decreased muscle protein catabolism and the number of infectious episodes compared with those whose excision and grafting and feeding had been delayed. 10
• A high protein diet may well be important during the period of rehabilitation, when patients have become anabolic. Demling studied patients who were healed and free of infection who had had burn injuries of 30–50%, all involved in physiotherapy programmes that included resistance exercise. 30 The group fed a high protein diet (0.32 g nitrogen/kg) gained more weight and showed greater muscle strength and ability to do endurance exercise than those on a lower protein intake (0.2–0.24 g nitrogen/kg). Regaining muscle is slow, with the rate of muscle protein synthesis reported as 10–25 g per day (5-10 times slower than the rate of loss during catabolism) in burn-injured patients.
Oxandrolone
Oxandrolone is an anabolic steroid, a testosterone analog with ten times the anabolic activity but one-tenth of the androgenic activity of testosterone. Research into its use in burn injury for preventing loss or restoring muscle mass has been going on since the mid-1990s. Demling found that patients in the rehabilitation phase on an exercise programme, oxandrolone and a high protein diet gained more body weight than those on an exercise programme and normal or high protein diet. 31 Oxandrolone was effective in older adults, over 60 years, as well as in younger adults. 32 This is important in light of the difficulties older adults face due to loss of lean body mass. In 2000 Demling used oxandrolone (20 mg/day) in the acute phase on burn injury, beginning when patients reached a minimum of 75% of their energy and protein requirements. 33 Twenty patients were studied in a double-blind randomised controlled trial. Patients on oxandrolone had an improved net nitrogen balance, decreased weight loss, faster healing of donor sites and a shorter length of stay. Oxandrolone did not decrease metabolic rate and there were no side effects. The use of oxandralone is being considered in burns in the UK.
Glutamine
Glutamine is an amino acid that becomes essential during critical illness. 34 Its role as the main fuel source for rapidly dividing cells in the mucosa of the GI tract and for lymphocytes, its involvement in gluconeogenesis and as a precursor for other amino acids all make it of crucial importance in burn injury. Increased uptake during this period by the intestinal mucosa, dividing leukocytes, liver and kidneys cannot be met by release from skeletal muscle and plasma levels fall. The European Society for Parenteral and Enteral Nutrition has supported the supplementation of burn-injured patients with glutamine. 35 Garrel and Zhou conducted trials that found a significant improvement in wound healing and a reduction in the length of stay. 36,37 Chen and Peng found a reduction in plasma endotoxin levels and an improvement in intestinal permeability. 38,39 Windle conducted a thorough review of all published data on the clinical impact of supplementation on patients with major burn injury and concluded that supplementation is of benefit. 40 Windle recommended that a minimum period of supplementation to be of benefit was 5 days, with trials varying from 1 to 4 weeks and most RCTs lasting at least 10–14 days. While a recommended dose has not been established the majority of trials on burns have used 0.35–0.57 g/kg. Plasma glutamine levels may be of benefit initially as an indication to begin therapy; however, reports vary on the effects of supplementation on plasma levels, therefore not clearly indicating an end point. However, Windle points out that this could be due to the different levels of glutamine supplementation in different trials. Even where plasma levels did not increase, clinical benefit was still found. A study by Wischmeyer identified raised plasma ammonia levels in patients with acute renal failure and liver dysfunction; 41 otherwise, the use of glutamine in burns has reported no other side effects. Some major burns units in the UK now supplement patients with burns who are critically ill.
Calculating protein requirements
In burns of 20% or greater monitoring of nitrogen balance using 24-hour urinary urea is beneficial and the calculation simple: 42,43


Any extra renal losses:
• pyrexia 0.6 g nitrogen/1°C with sweating
• burns exudates 0.2 g/% surface area burn
• extensive bed sores, GI fistulae or inflammatory bowel disease.
Currently the British Dietetic Association Burns Interest Group guidelines recommend the use of the Elia equation to initially calculate protein requirements (see Table 12.3). 44 While it is not possible to achieve nitrogen balance in patients with large burn injuries, high nitrogen intakes may well be beneficial in wound healing.
Nitrogen g/kg/day | |
---|---|
Normal | 0.17 (0.14–0.2) |
Hypermetabolic | |
5-25% | 0.2 (0.17–0.25) |
25-50% | 0.25 (0.20–0.30) |
> 50% | 0.30 (0.25–0.35) |
Depleted | 0.3 (0.2–0.40) |
Type of energy
Carbohydrate
During burn injury ketogenesis is partially inhibited. Glucose is the preferred source of energy as the substrate for the production of adenosine triphosphate through anaerobic glycolysis. Wolfe 45 studied glucose metabolism in burns using radio-isotopes and found that it was raised during burn injury compared with non-burn controls. 45 Wolfe went on to find that this was due to increased levels of glucagon, converting amino acids from protein catabolism into glucose. 46 Gluconeogenesis was not reversed by the infusion of glucose or glucose and amino acids. However, somatostatin, which was used to partially block the production of glucagon, did reduce the rate of protein breakdown. Provision of exogenous insulin also reduced muscle breakdown. 30 The burn wound has up to a tenfold increase in uptake of glucose compared with non-burned tissue. 47 Gottschlich recommended the provision of 60–65% of energy as carbohydrate as long as it does not exceed 5 mg/kg per minute, the maximum oxidation rate. 48 Hyperglycaemia during critical illness also needs to be controlled as it impairs immune function by altering cytokine production from macrophages, reducing lymphocyte production and the intracellular bactericidal activity of leukocytes. 49
Fat
Catecholamine production leads to the breakdown of adipose during burn injury, producing raised levels of free fatty acids and glycerol in the systemic circulation. Abbott found that ketone bodies are not significantly elevated, suggesting that ketogenenesis is inhibited and fatty acid oxidation is not increased. 50 It appears that the majority of free fatty acids are re-esterified by the liver to triglycerides or phospholipids. Excessive amounts of dietary fat associated with high lipid levels have been associated with impaired clotting and depression of the immune system. Therefore the provision of high levels of fat as an energy source is not recommended and 10–15% of total energy from fat has been proposed by Gottschlich. 51 However, dietary fat is also important as a carrier of fat soluble vitamins, for which a minimum of 15–25 g a day is required. Essential fatty acids, namely linolenic and linoleic acid, need to be provided. They cannot be synthesised by the body and form part of the cells’ phospho-lipid membrane. Evidence regarding the benefits of the provision of omega-3 fatty acids and their anti-inflammatory effects remains inconclusive and further studies are required.
Micronutrients
Trace elements
Since the 1980s it has been known that people sustaining large burn injuries could develop low serum levels of copper, zinc and selenium. 52 Trace element levels are, however, difficult to interpret during critical illness due to the redistribution around the body. Plasma zinc, iron and selenium fall while copper increases. 53 Trace elements are crucial as cofactors or as metalloenzymes in antioxidant systems (glutathione peroxidase, superoxide dismutase), wound healing (Lysyl oxidase, integrins) and protein synthesis (RNA polymerase). For more detail see Table 12.4
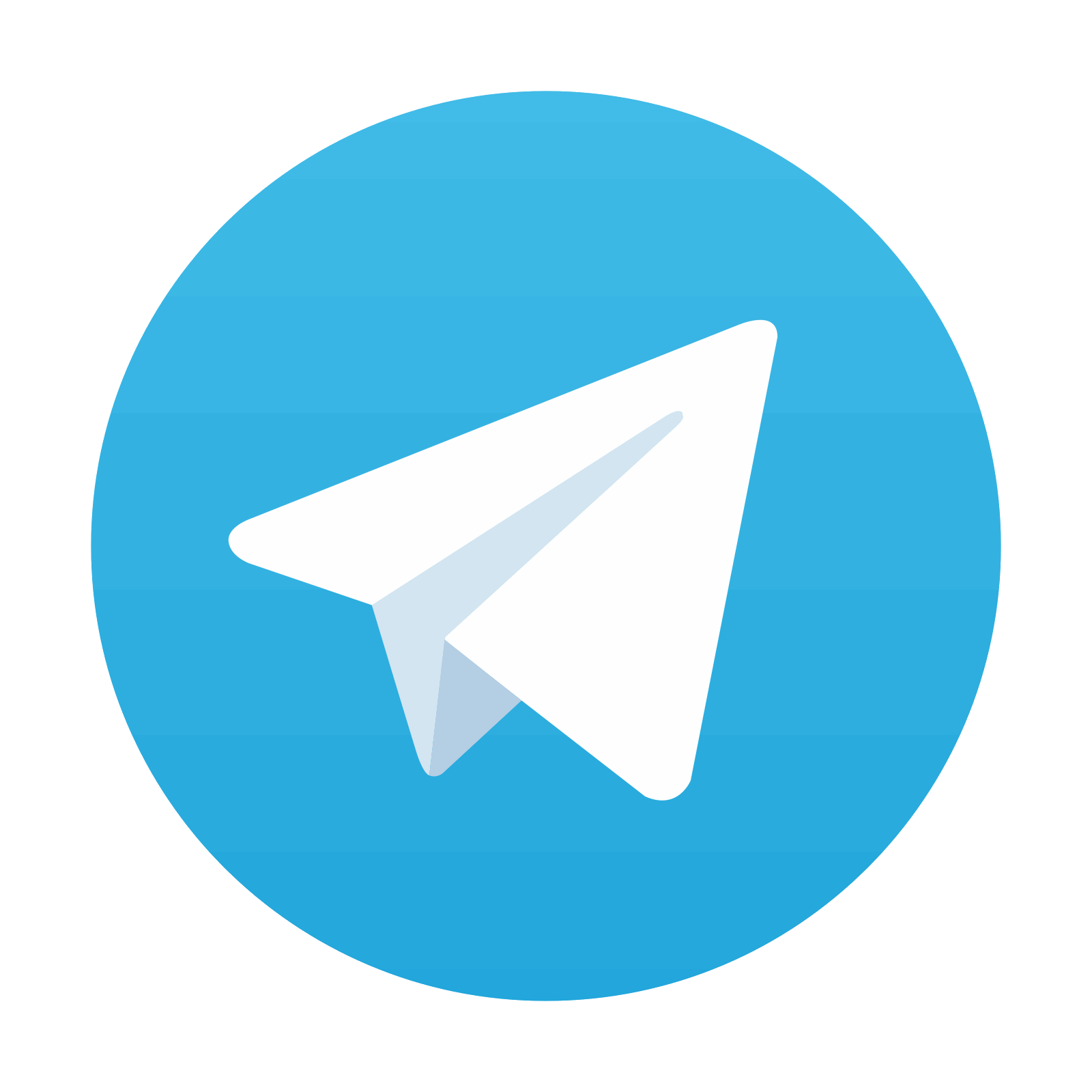
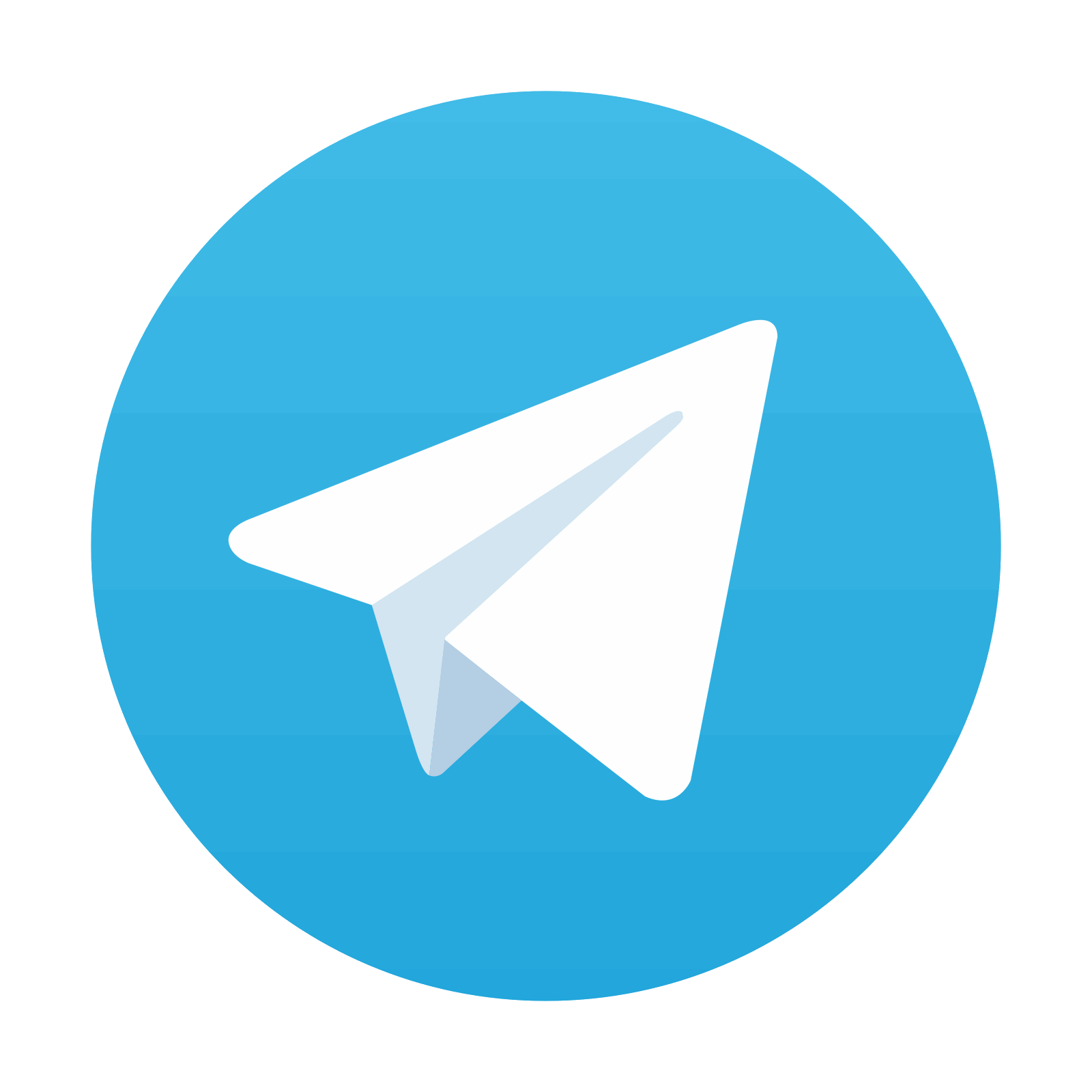
Stay updated, free articles. Join our Telegram channel

Full access? Get Clinical Tree
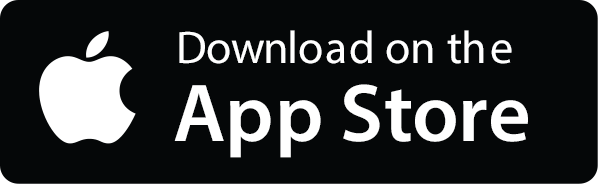
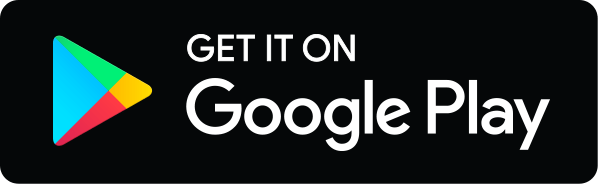
