The 5-year survival for all stages of nonsmall cell lung cancer (NSCLC) remains bleak, having increased from 13% to just 16% over the past 30 years. Despite promising results in nonoperative patients with NSCLC and pulmonary metastatic disease, thermal ablation appears to be limited by large tumor size and proximity to large vessels. This article discusses the particular challenges of performing thermal ablation in aerated lung tissue and reviews important considerations in performing ablation including treatment complications and imaging follow-up. The article compares and contrasts the three major thermal ablation modalities: radiofrequency ablation, microwave ablation, and cryoablation.
Lung cancer remains the leading cause of cancer death in the United States, accounting for an estimated 29% of cancer deaths in 2009. Pneumonectomy or lobectomy with hilar and mediastinal lymph node sampling is the gold standard treatment and offers the best option for cure of stage 1/2 nonsmall cell lung cancer (NSCLC). Unfortunately, only 15% of patients present with stage 1/2 disease, and many of these patients do not meet the pulmonary physiologic guidelines for lobar resection. In addition to lung cancer, pulmonary metastases are present in 25% to 30% of patients dying from all types of cancer. For some patients with oligometastatic pulmonary disease, metastectomy is associated with an improvement in survival. External beam radiation traditionally has been offered as the alternative to surgical resection for NSCLC or pulmonary metastatic disease. Unfortunately, the 5-year survival following radiation for stage 1 and 2 NSCLC remains low at 15% to 20%, with local recurrence being the most common mode of failure. Thermal ablation offers an intriguing therapeutic option to increase local tumor control and survival in patients with early stage NSCLC or with limited metastatic disease from nonlung primaries who are not surgical candidates because of poor cardiopulmonary reserve, anatomic constraints limiting resection, failure of traditional therapies, or refusal of operative approaches.
Thermal ablation has been shown to be effective in treating tumors in bone, kidney, and liver. Most preclinical and clinical trials have focused on demonstrating the feasibility of three modalities for pulmonary thermal ablation, namely radiofrequency (RF) ablation, microwave (MW) ablation, and cryoablation. This article discusses the unique challenges of performing thermal ablation in lung tissue and reviews the current literature regarding RF, MW, and cryoablation in the lung.
Thermal ablation in lung tissue
Clinical success in thermal ablation depends upon the unique characteristics of the tissue being ablated. A major obstacle for all thermal ablation techniques is the heat sink effect, which describes how bloodflow or airflow through or adjacent to the target tissue can offset the applied cooling or heating, thus limiting intended tissue damage. Experimental studies with RF ablation in liver demonstrated that perfusion-mediated cooling was responsible for the smaller ablation diameters obtained in in vivo versus in vitro experiments. Proximity to vessels larger than 3 mm also has been associated with increased risk of local tumor progression. Thermal heat sink effects are particularly important in lung ablation, as the lungs are exposed to 100% of right heart blood flow. In addition to vascular perfusion, the lungs are uniquely composed of air-filled spaces that are constantly ventilated, resulting in a second type of heat sink analogous to an air-cooled radiator.
A second factor influencing thermal ablation is the thermal conductivity of the tissue being ablated. Thermal conductivity is lower in lung than in other tissues, such as bone, liver, and kidney due to its high percentage of air by volume. Lower thermal conductivity of aerated lung would be expected to limit heat transfer into tissues adjacent to solid pulmonary masses, and if true, this could prevent adequate ablation of infiltrative margins or satellite tumors at the periphery of lung lesions. Although surrounding air-filled lung may restrict heat transfer into the partially aerated peripheral portion of lesions, it may also effectively insulate the interior of lesions and create improved tumor damage via an oven- or freezer-type effect. This has been demonstrated by animal data showing an increase in ablation zone size in implanted tumors in lung when compared with kidney. A histologic study of NSCLC showed that treatment margins of 8 mm for adenocarcinoma and 6 mm for squamous cell carcinoma are necessary to cover 95% of microscopic disease. The dichotomy of decreased transfer of heat to the periphery of lesions and the insulating effect of aerated lung can be an important consideration in choosing which lesions to treat and with what thermal ablation modality.
As with the liver, several investigators have attempted to overcome perfusion and ventilation heat sinks inherent to lung tissue. Ipsilateral mainstem bronchus occlusion and pulmonary artery occlusion have both been shown to significantly increase the mean volume of the ablation zone with RF ablation. Although the effect is larger with pulmonary artery occlusion, bronchial occlusion is much more practical to apply clinically.
Radiofrequency ablation
In RF ablation, an alternating electrical current (approximately 500 KHz) with 10 to 200 W of power is applied to the target tissue via an interstitial electrode, Two to four grounding pads on the skin surface complete the electrical circuit through the body. Current conducted through tissue adjacent to the electrode leads to ion agitation, which is converted by means of friction into heat. Heat generation is proportional to the current density and is attenuated exponentially with increasing distance from the electrode. As tissue is heated, predictable changes occur based on time and temperature. At temperatures between 42°C and 45°C, cells become susceptible to damage by outside agents like radiation and chemotherapy. Temperatures maintained above 46°C for a prolonged period of time (on the order of 30 to 60 minutes) cause irreversible cell damage. Between 50°C and 52°C, the time to cytotoxicity is reduced to 4 to 6 minutes. The goal of RF ablation is to achieve temperatures between 60°C and 100°C, where there is near instantaneous induction of protein coagulation with damage to cytosolic and mitochondrial enzymes and DNA–histone complexes leading to coagulative necrosis. Conversely, temperatures above 105°C cause boiling, desiccation, vaporization, and carbonization of tissues. The resulting impedance rise limits electrical current flow, leading to reduced coagulative necrosis volumes. Thus, the therapeutic temperature range for RF ablation is narrow (60°C to 100°C).
Early percutaneous RF ablation technology was able to create ablation zones only 1.6 cm in diameter. Many strategies since have been developed to increase the size of RF ablations. For example, internally cooled electrodes reduce tissue charring near the electrode and permit greater energy delivery. When combined with pulsed power delivery, the cooled electrodes were able to increase ablation zone size to approximately 2 cm in diameter while keeping at 17-gauge profile. Clustered, deployable, and multipolar electrode designs use a different approach by increasing the effective surface area of the electrode and distributing energy delivery over a larger volume ( Fig. 1 ). When compared directly, it has been shown that these designs produce potentially larger ablations than the single cooled electrode design, but often at the expense of irregular shape, protracted treatment times, or increased applicator diameter (14-gauge). More recently, a system that exploits the aforementioned pulsing algorithm to switch power between multiple electrodes has been shown to increase ablation zone size while retaining a relatively spherical ablation shape.
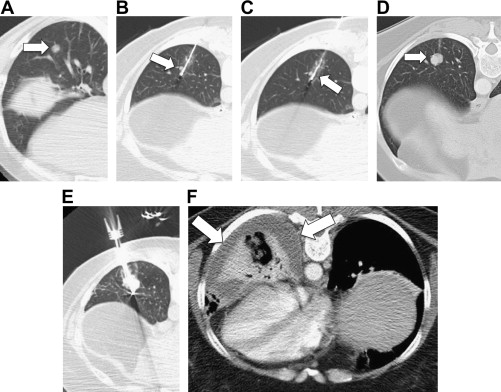
While most studies have focused on the performance of RF devices in liver models, some have demonstrated that multitined or multiple-electrode designs may produce more effective ablations in the lung due to their ability to spread out energy delivery. However, it also has been noted that deployable designs can be more problematic to use in lung. For example, ballotable solid tumors can be difficult to penetrate with a deployable device, and some studies have described difficulty retracting the tines after treatment.
Another strategy to overcome low tissue conductivity is by infusion of sodium chloride solutions into the targeted ablation zone. Sodium chloride is ionic and thus improves the electrical conductivity of the surrounding tissue. Infusion during treatment also prevents charring and keeps the ablated tissue hydrated, resulting in a larger zone of ablation. However, saline infusion can produce irregular and unpredictable ablations with potentially serious complications and thus is not routinely performed.
There are multiple US Food and Drug Administration (FDA)-approved RF ablation devices on the market with different performance characteristics. Examples include: the Angiodynamics 1500X RF generator with the StarBurst and Uniblate electrodes (Latham, NY, USA), the Boston Scientific RF3000 with LaVeen electrodes (Natick, MA, USA) (both of which use multitined, expandable electrodes) ( Fig. 2 ) and the Covidien Cool-tip system (Boulder, CO, USA), which uses either a single straight electrode, cluster of three electrodes, or up to three independent, switched straight electrodes that are actively cooled during ablation ( Fig. 3 ). Importantly, these systems were all developed for use in liver, and have been applied in lung without modification. Currently, there are no devices available for clinical use optimized for treating tumors in the lung.
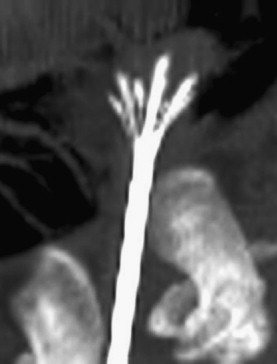
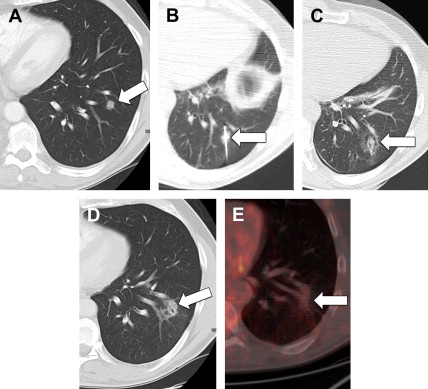
Animal models first were used to investigate RF ablation in normal lung tissue to develop treatment algorithms for people. Human ablate-and-resect studies also were performed and, although early results were mixed, they demonstrated that RF ablation was feasible for lung tumors, ultimately leading to clinical use, A systematic review published in 2008 summarized the literature regarding RF ablation. Among the 17 studies included in the review, the median complete necrosis rate was 90% (range: 38% to 97%) with 1-, 2- and 3-year survival rates of 63% to 85%, 55% to 65%, and 15%–46%, respectively. The only prospective single-arm multicenter intent-to-treat clinical trial of RF ablation in 106 patients (33 non-small cell lung cancer, 53 colorectal cancer metastases, 20 other metastases) found promising overall and cancer-specific survival rates in these patients. A summary of lung RF ablation studies is listed in Table 1 .
Study | Patient/Lesions | Pathology | Tumor Diameter (Mean and Range in cm) | Median Follow-Up (months) | Complications | Outcome/Comments |
---|---|---|---|---|---|---|
Akeoboshi et al, 2004 | 31 patients/44 lesions | NSCLC, 13 Metastatic, 41 | 2.7 (0.7–6.0) | 9.3 | Ptx: 29% Ptx with chest tube: 16% Lung abscess: 6% | Complete necrosis in 69% of lesions <3 cm and 39% of lesions >3 cm |
Ambrogi et al, 2006 | 54 patients/64 lesions | NSCLC, 40 Metastatic, 24 | 2.4 (1.0–5.0) | 23.7 | Ptx: 12.7% Ptx with chest tube: 6.4% | Complete response in 61.9%, higher (69.7%) in lesions <3 cm |
Belfiore et al, 2004 | 33 patients/35 lesions | NSCLC, 35 | 3.5 (1.8–6.0) | — | Ptx: 9%, none with chest tube Pleural effusion: 9% | Pathologic follow-up at 6 months showed complete ablation: 36%, partial necrosis: 63% with patient pain, cough, and dyspnea scores all improved at 6 and 12 months |
Gadaleta et al, 2004 | 18 patients/40 lesions | NSCLC, 4; Metastatic, 14 | 3.0 (0.6–11) | 9.2 | Ptx: 16.7% Ptx with chest tube: 12.5%, | Complete ablation 94% |
Gilliams et al, 2007 | 37 patients/72 lesions | All metastatic | 1.8 (0.4–6.6) | 11.3 | Major complication: 6% (ptx requiring chest tube, pleural effusion requiring drainage, tumor track seeding), Minor complication: 14% (ptx, postprocedural pain) | Recurrence in 100% of lesions >3.5 cm and 29% of lesions <3.5 cm Recurrence in 58% of lesions in direct contact with vessels and 23% in others ( p >.04) |
Hiraki et al, 2006 | 128 patients/342 lesions | NSCLC, 25 Metastatic, 317 | 1.7 (0.3–9.4) | 12 | — | Primary and secondary effectiveness rates: 72% and 84% at 1 y and 58% and 66% at 3 y Local progression: 27% of tumors (1–30 months), 52% of these were treated with repeat ablation Worse outcome with internally cooled electrode |
Lee et al, 2004 | 30 patients/32 lesions | NSCLC, 27 Metastatic 5 | 5.2 (0.5–12.0) | 12.5 | Ptx: 23% Ptx with chest tube: 7% | Complete necrosis: 100% lesions <3 cm, 38% of lesions 3–5 cm. and 8% of lesions >5 cm Survival with complete necrosis: 19.7 months compared with 8.7 months with partial necrosis |
Lencioni et al, 2004 | 106 patients/183 lesions | NSCLC, 33Metastatic 73 | 1.7 (0.5–3.4) | 12 | Ptx: 20.1% Ptx with chest tube 19.7% | Overall survival: 70% at 1 y and 48% at 2 y in patients with NSCLC, 89% at 1 y and 66% at 2 y in patients with colorectal metastases, and 92% at 1 y and 64% at 2 y in patients with other metastases Cancer-specific survival: 92% at 1 y and 73% at 2 y in patients with NSCLC, 91% at 1 y, and 68% at 2 y in patients with colorectal metastases Patients with other metastases: 93% at 1 y and 67% at 2 y |
Yan et al, 2006 | 55 patients | All Colorectal Metastases | 2.1 (not given) | 24 | Ptx: 29% Ptx with chest tube: 16% Lung abscess: 4% | Median survival 33 months. 1-, 2- and 3-y actuarial survival rates were 85%, 64%, and 46% Size <3 cm was signiciantly associated with improved survival ( p <.001) |
Yasui et al, 2004 | 35 patients/99 lesions | NSCLC, 3; Metastatic, 96 | 2.0 (0.3–8.0) | 7.1 | Ptx: 35.2% Ptx with chest tube: 4% Fever: 12%, Intraprocedureal pain 29% | Probable complete ablation: 91% |
There are several factors that determine the likelihood of a successful ablation, but similar to other organ systems, tumor size is one of the most important considerations. Tumors less than 3.0 cm tend to be associated with complete necrosis in most cases, with less than 50% complete necrosis in tumors from 3.0 to 5.0 cm, and a low likelihood of successful ablation in tumors greater than 5.0 cm. Importantly, these studies illustrate that complete necrosis has a positive effect on survival and should be the goal of any ablative treatment.
One advantage of RF ablation over surgical resection and radiation therapy is repeatability. The minimally invasive nature of percutaneous ablation allows for multiple treatment sessions on a given tumor or patient with relatively low complication risk. In fact, it has been shown that repeat treatment of local tumor progression after the primary treatment can improve survival. In contrast, particularly in patients with a limited pulmonary reserve, repeat surgery is often not possible, and radiotherapy is associated with a maximum tolerated dose, which limits repeat treatment. Thus, all patients with local tumor progression should be considered for retreatment unless there is evidence of regional or distant disease.
When radiation therapy is a viable treatment option, RF ablation and external beam radiation appear to be synergistic. RF ablation is most effective in the center of relatively avascular tumors, while external beam radiation and stereotactic radiosurgery are most effective at the periphery of the tumor where there is high oxygen content and a hyperthermic rim around the ablation zone. Combining the two therapies has been shown to increase survival, at no additional toxicity, as compared with radiation therapy alone, and this technique should be considered for larger tumors.
In summary, RF ablation has been shown to be a suitable means of local tumor control for selected small tumors in patients with NSCLC or pulmonary metastatic disease. Pulmonary function, as measured by forced expiratory volume in the first second of respiration (FEV 1 ) and forced vital capacity (FVC), is well preserved following ablation, giving it a significant advantage in patients with limited pulmonary reserve. Despite these promising results, RF ablation continues to be plagued by modest rates of local tumor progression, particularly in tumors greater than 3 cm and in the vicinity of larger heat sinks. Further investigation will be required to continue to improve the efficacy of this promising technique and the associated adjuvant techniques.
Radiofrequency ablation
In RF ablation, an alternating electrical current (approximately 500 KHz) with 10 to 200 W of power is applied to the target tissue via an interstitial electrode, Two to four grounding pads on the skin surface complete the electrical circuit through the body. Current conducted through tissue adjacent to the electrode leads to ion agitation, which is converted by means of friction into heat. Heat generation is proportional to the current density and is attenuated exponentially with increasing distance from the electrode. As tissue is heated, predictable changes occur based on time and temperature. At temperatures between 42°C and 45°C, cells become susceptible to damage by outside agents like radiation and chemotherapy. Temperatures maintained above 46°C for a prolonged period of time (on the order of 30 to 60 minutes) cause irreversible cell damage. Between 50°C and 52°C, the time to cytotoxicity is reduced to 4 to 6 minutes. The goal of RF ablation is to achieve temperatures between 60°C and 100°C, where there is near instantaneous induction of protein coagulation with damage to cytosolic and mitochondrial enzymes and DNA–histone complexes leading to coagulative necrosis. Conversely, temperatures above 105°C cause boiling, desiccation, vaporization, and carbonization of tissues. The resulting impedance rise limits electrical current flow, leading to reduced coagulative necrosis volumes. Thus, the therapeutic temperature range for RF ablation is narrow (60°C to 100°C).
Early percutaneous RF ablation technology was able to create ablation zones only 1.6 cm in diameter. Many strategies since have been developed to increase the size of RF ablations. For example, internally cooled electrodes reduce tissue charring near the electrode and permit greater energy delivery. When combined with pulsed power delivery, the cooled electrodes were able to increase ablation zone size to approximately 2 cm in diameter while keeping at 17-gauge profile. Clustered, deployable, and multipolar electrode designs use a different approach by increasing the effective surface area of the electrode and distributing energy delivery over a larger volume ( Fig. 1 ). When compared directly, it has been shown that these designs produce potentially larger ablations than the single cooled electrode design, but often at the expense of irregular shape, protracted treatment times, or increased applicator diameter (14-gauge). More recently, a system that exploits the aforementioned pulsing algorithm to switch power between multiple electrodes has been shown to increase ablation zone size while retaining a relatively spherical ablation shape.
While most studies have focused on the performance of RF devices in liver models, some have demonstrated that multitined or multiple-electrode designs may produce more effective ablations in the lung due to their ability to spread out energy delivery. However, it also has been noted that deployable designs can be more problematic to use in lung. For example, ballotable solid tumors can be difficult to penetrate with a deployable device, and some studies have described difficulty retracting the tines after treatment.
Another strategy to overcome low tissue conductivity is by infusion of sodium chloride solutions into the targeted ablation zone. Sodium chloride is ionic and thus improves the electrical conductivity of the surrounding tissue. Infusion during treatment also prevents charring and keeps the ablated tissue hydrated, resulting in a larger zone of ablation. However, saline infusion can produce irregular and unpredictable ablations with potentially serious complications and thus is not routinely performed.
There are multiple US Food and Drug Administration (FDA)-approved RF ablation devices on the market with different performance characteristics. Examples include: the Angiodynamics 1500X RF generator with the StarBurst and Uniblate electrodes (Latham, NY, USA), the Boston Scientific RF3000 with LaVeen electrodes (Natick, MA, USA) (both of which use multitined, expandable electrodes) ( Fig. 2 ) and the Covidien Cool-tip system (Boulder, CO, USA), which uses either a single straight electrode, cluster of three electrodes, or up to three independent, switched straight electrodes that are actively cooled during ablation ( Fig. 3 ). Importantly, these systems were all developed for use in liver, and have been applied in lung without modification. Currently, there are no devices available for clinical use optimized for treating tumors in the lung.
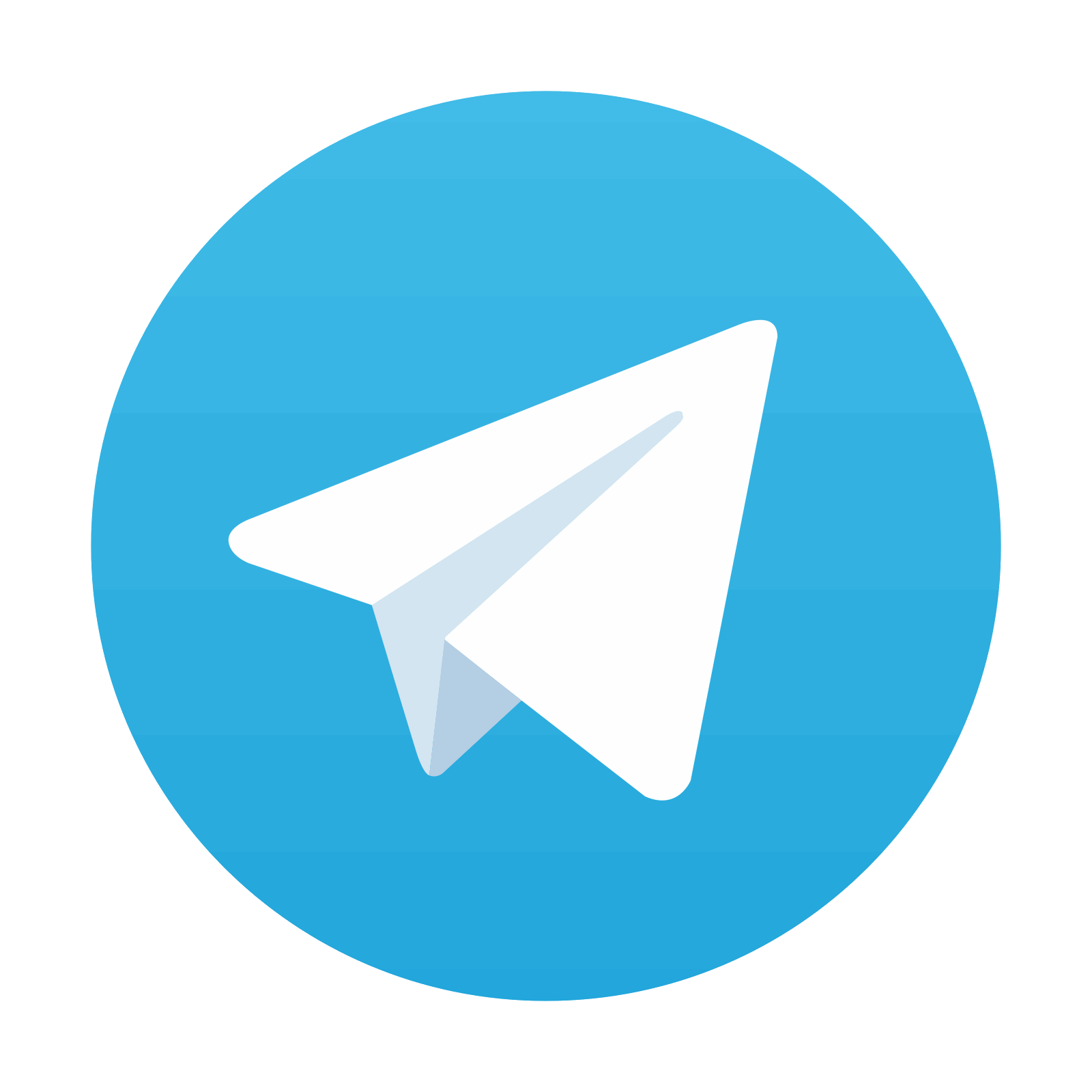
Stay updated, free articles. Join our Telegram channel

Full access? Get Clinical Tree
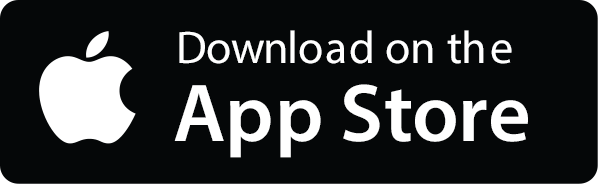
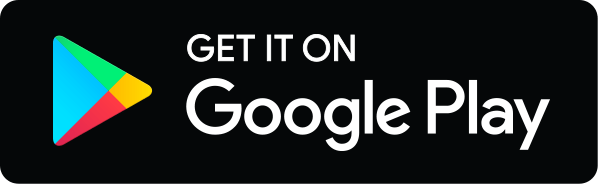
