Alkylating agent therapy
DNA-topoisomerase II inhibitor therapy (epipodophyllotoxins and anthracyclines)
Intense therapy
High doses of chemotherapy for prolonged periods as in therapy for Hodgkin’s disease and non-Hodgkin’s lymphoma
Direct correlation between intensity of original therapy and latency period to development of myelodysplasia
High energy β-emitters: 32P for polycythemia rubra vera
Occupational exposure: benzene, xylene
For this subtype of leukemia, such terms as therapy- or treatment-related leukemia are descriptive because patients previously received chemo- or radiation therapy, or both, for a primary disease. These terms are restrictive because the leukemia that develops after exposure to either benzene or several other occupational mutagens is very similar, if not identical, to the therapy-related leukemia syndrome. The term secondary leukemia correctly denotes that the disease did not develop spontaneously or de novo. The term is too broad, however, because a number of pathways, including genetic predisposition, probably lead to the development of AML.
According to the revised 2008 World Health Organization (WHO) classification, this syndrome is named therapy-related myeloid neoplasms including t-MDS/t-AML and t-MDS/t-AML/t-myeloproliferative neoplasm (MPN), and remains as a distinct subgroup in the AML classification. Although cases may be designated as t-AML, or as t-MDS or t-MDS/t-MPN depending on the blast count, it is useful to think of them as a single biologic disease with similar genetic features, so that the designation as t-MDS/t-AML is appropriate as well [1].
Clinical and Laboratory Features
Many of the clinical and laboratory features of t-MDS and t-AML are similar to MDS and AML, when they occur de novo. Most of these individuals experience various symptoms of bone marrow failure at diagnosis, such as fatigue, weakness, and fever. Nearly all affected individuals show physical signs of anemia, such as pallor and exertional dyspnea. Signs of thrombocytopenia, bruising, and hemorrhage are also relatively common, as are signs of infection. Less frequently, patients may have clinical manifestations of hepatomegaly, splenomegaly, lymphadenopathy, gingival hypertrophy, skin infiltration, and neurological abnormalities.
Preleukemic Phase
A preleukemic or myelodysplastic phase occurs in over 70 % of patients in whom AML develops following chemotherapy and/or radiation therapy for another disease [2–10], whereas about 20 % of patients with de novo AML have a similar preleukemic phase (Table 24.2). Indeed, the data suggest that a preleukemic period can be observed in nearly all patients with therapy-related leukemia, when these patients are monitored closely. Exceptions are those individuals in whom t-AML develops after therapy with epipodophyllotoxins (VP16 and VM26) or other DNA-topoisomerase II inhibitors. In these patients, t-AML often develops with no preleukemic phase [11, 12]. The mean duration of the preleukemic phase is 11.2 months in typical t-AML. The preleukemic phase in individuals with de novo MDS who go on to develop AML is similar, about 14 months [3, 4, 10].
Table 24.2
Comparison of therapy-related and de novo acute myelogenous leukemia
Therapy-related AML 1.Frequent preleukemic phase (70 %) 2.Frequent chromosomal abnormality (80–90 %); especially -5/5q- and/or -7/7q- 3.Hypodiploid modal number of chromosomes most common; often complex chromosomal abnormalities 4.Refractory to antileukemic therapy (80 %) 5.Short survival after leukemia diagnosis (mean 4–6 months) |
De novo AML 1.Less frequent preleukemic phase (20 %) 2.Chromosomal abnormality less frequent, especially +8, −7, t(8,21), and t(15,17) 3.Diploid or hyperdiploid modal number of chromosomes (95 %) 4.Remission induced by antileukemic therapy (75 %) 5.Longer survival after leukemia diagnosis (mean 20 months) |
The clinical manifestation of the preleukemic phase in t-AML is marked by ineffective hematopoiesis. The bone marrow morphology is characterized by trilineage dysplasia. The degree of dysplasia is usually very prominent. Interestingly, the RAEB and RAEB-t subgroups are more frequent in t-MDS (73 %) than in de novo MDS (53 %) [13]. Prominent abnormalities are observed in the red blood cells and their precursors. Most patients show decreased red cell production with low reticulocyte counts [5]. Oval macrocytosis and nucleated red cells are often the earliest recognizable changes observed in the peripheral blood in the preleukemic phase [14]. Macrocytosis after therapy for Hodgkin’s disease was retrospectively found to be associated with a high risk of the development of leukemia [15]. Mild neutropenia is present in 75 % of the individuals [5]. Neutrophils may be poorly granulated, and their nuclei can be hyposegmented (pseudo-Pelger-Hüet anomaly) [16]. Thrombocytopenia occurs in approximately 60 % of patients [5], and they may be abnormally large and degranulated. Both the neutrophils and platelets can have a variety of qualitative defects. The bone marrow is often hypercellular, although hypo- and normocellular marrow can occur. Erythroid hyperplasia, megaloblastoid features, and occasionally ringed sideroblasts dominate the marrow picture [16–18]. Abnormalities of the marrow granulocytic and megakaryocytic series are usually more subtle. Micromegakaryocytes may be seen, particularly with monosomy 7. The percentage of immature granulocytic and megakaryocytic cells may be increased. The primary and specific granules of the granulocyte precursors occasionally are either deficient or abnormally large. Marrow fibrosis often is present during the preleukemic phase.
In summary, the development of unexplained pancytopenia and the finding of karyotypic abnormalities in the marrow cells of patients who received chemotherapy and/or radiation therapy for another disease are pathognomonic of preleukemia. Evolution to overt leukemia is universal if the preleukemic individual survives the complications of hemorrhage and infection. t-MDS can be viewed as an early phase of t-AML in which the malignant hematopoietic clone is established and becomes predominant.
Acute Leukemia Phase
Clinical manifestations of individuals with t-AML are typical of bone marrow failure, and their clinical course is rapidly fatal often from complications of bleeding and infection. The bone marrow morphology of t-AML has been difficult to classify according to FAB criteria for AML, as most of the leukemias demonstrate trilineage involvement and appear to bridge several subtypes. Nevertheless, the blast cells of patients with t-AML most often are myeloblastic in appearance according to AML without maturation or AML with minimal differentiation in agreement with the 2008 WHO classification. A lower frequency of acute monocytic forms of leukemia has been reported in several studies as compared to de novo AML [9, 19].
Auer rods are rarely observed in the blast cells in t-AML, but are seen in blast cells of 35 % of patients with de novo AML. Many of the blast cells in t-AML lack myeloperoxidase and other granulocyte-specific enzymes. In one series, only one of ten patients with secondary AML had more than 10 % peroxidase-positive blast cells compared with nearly 100 % peroxidase-positive blast cells in 95 % of patients with de novo AML [19]. In addition, less than 20 % of the t-AML patients have either greater than or equal to 10 % naphthol ASD chloroacetate esterase-positive blast cells compared with 47 % of patients with de novo AML. These histochemical data suggest that the leukemic cells from secondary leukemia patients are blocked at an earlier stage of differentiation than the leukemic cells from most de novo AML patients.
Karyotypic Abnormalities in t-MDS/t-AML
Clonal chromosomal abnormalities can be detected in the blast cells of 80–95 % of t-MDS/t-AML patients by routinely available techniques [2, 6, 7, 9, 20–22]. Although the same abnormalities have been reported in primary MDS/AML, their frequency is clearly higher in t-MDS/t-AML patients (Table 24.2). A hypodiploid modal number of chromosomes occur most frequently in t-MDS/t-AML patients. Hyperdiploidy, mainly trisomy 8 is rare and is often observed as an inconsistent aberration present in only a subclone of cells [21]. The most common abnormalities are losses of all or part of chromosomes 5 and/or 7, especially in patients previously treated with high doses of alkylating agents [20, 23–26]. Review of the literature demonstrates that more than 70 % of the t-MDS/t-AML patients have lost either part or all of chromosome 5 and/or 7 associated with an extremely short survival. Chromosomes 5q and 7q probably contain critical myeloid tumor suppressor genes in de novo and t-AML. The breakpoints for the deletions are variable, but a common chromosome region, the so-called critical region, is almost always deleted.
For chromosome 5, Le Beau et al. have narrowed down the critical region to 5q31.1, which includes the early growth response gene (EGR-1) [27]. Other genes located on the long arm of chromosome 5 include many growth factor genes, namely granulocyte-macrophage colony-stimulating factor (GM-CSF), and interleukins-3, -4, and -5 (IL-3, -4, -5) [20, 28–31], and the growth factor receptor genes known to be present on the long arm of chromosome 5, namely macrophage colony-stimulating factor (M-CSF or FMS), platelet-derived growth factor (PDGF), glucocorticoid, alpha1-adrenergic, beta2-adrenergic, and D1-dopamine receptors [32, 33]. Analyses of 29 MDS/AML samples with 5q- revealed marked decreases in expression of alpha-catenin (CTNNA1) on 5q31, as compared to non-5q- MDS samples [34]. Moreover, Lehmann et al. identified several candidate genes within the commonly deleted region on 5q32 in twelve 5q- MDS samples that showed the most pronounced decrease in expression compared to non-5q- samples, namely solute carrier family 36, member 1 (SLC36A1), Ras-GTPase-activating protein SH3 domain-binding (G3BP), antioxidant protein 1 (ATOX1), colony-stimulating factor-1 receptor precursor (CSF1R), ribosomal protein S14 (RPS14), Nef-associated factor 1 (TNIP1), secreted protein, acidic and rich in cysteine (SPARC), annexin VI (ANAX6), NSDT, and TIGD [35]. The loss or abnormal regulation of these proteins could certainly contribute to hematopoietic dysregulation. However, no evidence implicates these proteins.
The breakpoints for the deletions of 7q are variable, but a common chromosome region, the so-called critical region, is located at band 7q22 proximally with the distal breakpoint varying from q31 to q36. Potentially important genes have been mapped to 7q, including genes for erythropoietin, p glycoprotein 1/multiple drug resistance 1 (MDR-1), and MDR-3 [36]. However, none has yet been shown to be involved1 in the development of t-MDS/t-AML [27, 32, 33, 36, 38]. In familial MDS with chromosome 7q abnormality (the “monosomy 7 syndrome”), the predisposition locus for development of MDS was mapped to a chromosomal location that is not 7q but to an unidentified location [39, 40]. These yet unidentified genetic loci may control predisposition to chromosomal instability leading to secondary losses of specific chromosomal regions such as 5q, 7q, and others, and MDS and leukemia ultimately develop.
Although most of the chromosomal abnormalities reported in t-MDS/t-AML are either complete or partial deletion of chromosome 7 or 7q [del(-7/7q)], and/or 5, or 5q del(-5/5q)], in recent years recurring unbalanced translocations that also result in loss of the long arm of 7 and/or 5 have been reported with increasing frequency. These include t(1;7)(p11;q11), t(5;7)(q11.2;p11.2), and t(7;17)(p11;p11) for chromosome 7, and t(5;7)(q11.2;p11.2) and t(5;17)(p11;p11) for chromosome 5 [41]. While the loss of function of a single gene in each of these relatively large regions is possibly responsible for the development of t-MDS/t-AML, hemizygous loss of the function of several genes in each of these regions could also contribute to the disease phenotype. Another, not mutually exclusive hypothesis is that an unknown initiating abnormality causes genomic instability leading to the deletion and rearrangement of particularly susceptible chromosome regions, such as those on chromosome 5q and 7q.
A review of 431 cases of secondary leukemia found 16 nonrandom chromosomal changes involving chromosomes 3, 5, 7, 8, 9, 11, 14, 17, and 21. These changes were dependent on the type of primary disease, previous therapy, age, and gender [42]. In another single institution study consisting of 63 patients, additional abnormalities in chromosomes 1, 4, 5, 7, 12, 14, and 18 occurred, with significantly increased frequency of these changes in t-AML as compared to de novo AML [20]. Abnormalities in chromosome 17, especially translocations involving bands 17p11-p13 and 17q21 are occasionally observed in t-AML, for example, t(15;17)(q22;q11–21) [24, 25]. Other chromosomes often reported to be abnormal in t-MDS/t-AML are chromosomes 21 and 11, particularly involving balanced translocations of chromosome bands 11q23 and 21q22 in t-AML [i.e., t(4;11), t(6;11), t(9;11), t(11;19), t(3;21), and t(8;21)] [21, 32, 41, 42]. These translocations are associated with previous therapy targeting DNA-topoisomerase II, primarily the epipodophyllotoxins and the anthracyclines. The 11q23 reciprocal translocations and interstitial deletions structurally interrupt a small region of the MLL (also known as HRX, ALL-1, HTRX1) gene that codes for a human homolog of the Drosophila trithorax gene [43, 44]. A fragment of the MLL gene translocates to more than 60 other chromosomal regions, resulting in the creation of a fusion protein with the partner gene.2
The t(9;11) that results in a fusion between MLL and AF9 is a recurring chromosomal translocation in de novo AML and is one of the most common recurring chromosome translocations detected in about 50 % of t-AML patients with MLL involvement [48]. In addition, involvement of the AF9 gene in the development of t-AML is linked to the treatment with topoisomerase inhibitors [49]. Also, in children with acute lymphocytic leukemia (ALL) translocations to chromosome band 11q23 predominated in children with t-AML after therapy with the epipodophyllotoxins [11]. Interestingly, the unbalanced rearrangements of the same two bands, 11q23 and 21q22, were most often associated with therapy with alkylating agents alone or in combination with radiation therapy [32]. In Chinese patients treated for psoriasis with bimolane, t(15;17) has been frequently reported. Also, therapy with doxorubicin has been associated with an increased incidence of t-AML with balanced translocations at chromosome band 21q22, in particular t(3;21) [21, 42]. In addition to balanced translocations involving chromosome bands 11q23 and 21q22, other balanced aberrations such as inv(16), t(8;16), t(15;17), and t(6;9) have been observed in t-AML after previous therapy with drugs targeting DNA topoisomerase II (Table 24.3) [21, 41].
Table 24.3
Features of therapy-related acute myelogenous leukemia associated with prior exposure to DNA-topoisomerase II inhibitors
Short median latency period (33 months) |
Frequently no preleukemic phase; if preleukemic phase, it rapidly evolves to acute leukemia |
Morphologic phenotype often M4/M5 (by former FAB classification) |
Chromosomal abnormalities usually do not involve chromosome 5 and/or 7, but often occur at chromosome 11q23 or 21q22 |
Response to cytotoxic therapy similar to de novo AML, according to karyotypic abnormalities |
In a recent study of 491 t-MDS/t-AML patients with at least one balanced translocation, Rowley and Olney reported that 149 of the patients were positive for the 11q23 translocation (30.3 %), followed by the 21q22 rearrangement seen in 15 %, inv(16) in 9 %, and t(15;17) in 8 % of the patients [48]. Interestingly, no significant difference occurred in the gender distribution of patients within the subgroups, and patients in the 11q23 subgroup were the youngest age at their primary and secondary diagnosis. Moreover, the translocation 11q23, inv(16), and t(15;17) subgroups had the shortest latency, with a median latency of 25.9 months for translocation 11q23, 22.0 months for inv(16), and 28.9 months for t(15;17) [48].
Chromosome studies have shown that when t-MDS becomes clinically diagnosable, the preleukemic clone represents a majority of the hematopoietic cells [2, 9, 20]. Additional chromosomal abnormalities occur in the original abnormal clone in 60–70 % of cases as the disease evolves to frank leukemia [50, 51]. Karyotypic evolution usually involves further deletions or losses of chromosomes and a change to a lower modal chromosome number; rarely, the evolution is associated with a gain of chromosome 8. Evidence suggests that t-MDS patients who have a mixture of karyotypically normal and abnormal cells (AN) survive longer than those who have only abnormal cells (AA) [52]. Most individuals who are AN in the preleukemic phase become AA as the disease progresses [20]. Notably, Rowley and Olney observed in their study that patients presenting with a t-MDS had significantly more frequent abnormalities of chromosomes 5 and/or 7 (49 %) than did patients presenting with a t-AML (16 %), and that this subgroup also presented with the highest percentage of complex karyotypes (45 % vs. ca. 20 % for both 1 and 2 aberrations) [48].
Relationship of Particular Karyotypic Pattern in Acute Leukemia to Age and Occupation
Specific karyotypic abnormalities of the leukemic cells predominate at specific ages of the patient [53]. For instance, loss of chromosome 5 is very rare in children with acute nonlymphocytic leukemia and loss of chromosome 7 is relatively uncommon; on the other hand, the translocation of chromosomal segments between chromosomes 8 and 21 involving AML1/ETO in nonlymphocytic leukemia cells is very common in children, but rare in adults. Loss of chromosome 5 and/or 7 more than doubles in individuals over the age of 50 compared with those under 50 years of age. A gain of chromosome 8 increases with age, but not quite so dramatically. In the few studies in which the occupation of the leukemic patient has been correlated with the karyotype of the leukemic cells, losses of chromosome 5 and/or 7 or gain of chromosome 8 were increased by 50 % in the occupationally exposed compared with the nonexposed patients [53–55]. The observation that loss of chromosome 5 and/or 7 is rare in children and increases with age and that these same changes are much more common in leukemic patients who are classified as occupationally exposed to mutagenic agents lends support to our hypothesis that these chromosomal aberrations are both particularly sensitive indicators of exposure to mutagens and are probably critical to the development of therapy-related leukemia.
Latency and Risk of Therapy-Related Leukemia
An increased incidence of acute leukemia following the treatment of Hodgkin’s disease was first observed in the early 1970s. Numerous studies have since clearly shown that chemotherapy or radiation therapy, or both, for the primary disease is strongly linked to an elevated risk of acute leukemia. However, the incidence and risk of t-MDS/t-AML remain difficult to estimate and highly variable between different studies and different primary diseases. This variability is due in part to cohort differences in age, duration, intensity, and the types of treatment, as well as the rigor of the follow-up. Nonetheless, the problem is considerable and is likely to increase, as we are more successful in treating various malignancies.
Median latency to the development of t-MDS/t-AML reported over the last 20 years has been quite consistent among studies, about 4–5 years after chemotherapy and/or radiation therapy for a primary disease [32]. The incidence is highest between 24 and 60 months after therapy, declining steadily thereafter.
The risk of developing t-AML depends on the type of treatment. Chemotherapy both with or without radiation therapy carries a higher risk, and radiation therapy alone carries the smallest risk. TBI is riskier than high dose, limited-field therapy. A positive correlation exists between the intensity of therapy given to an individual or experimental animal and their risk of developing leukemia. An inverse correlation may exist between the amount of therapy given to an individual and their latency period for development of secondary leukemia. For example, those who received the largest amount of alkylating agents [56–59], or etoposide [11, 12, 60, 61], had the greatest risk of t-AML.
Although not the subject of this chapter, the incidence of several neoplasms, such as non-Hodgkin’s lymphoma (NHL), breast cancer, lung cancer, and others, is also clearly increased in individuals who received therapy for another disease [62–64]. The heavily irradiated survivors of Hiroshima and Nagasaki had an increased incidence of chronic myelogenous leukemia (CML) and ALL, as well as AML [65]. Curiously, only rare reports have associated the development of CML with prior exposure to chemotherapy, benzene, or other industrial toxins. For example, two studies reported about one patient with Hodgkin disease and two patients with NHL who developed treatment-related CML, respectively [66, 67].
The assessment of risk of leukemia in relationship to type of primary disease is not available. One composite report suggests that the leading disease categories associated with t-AML are Hodgkin’s disease, multiple myeloma, NHL, breast, and ovarian cancer. The risk of leukemia, however, is related more to the type of therapy given for the primary disease than to the category of primary disease.
Hodgkin’s Disease
Leukemia that follows treatment of Hodgkin’s disease has been the most studied t-MDS/t-AML (Table 24.4) [68–77, 80–84]. The actuarial risk of t-MDS/t-AML is 3–4 % at 9–10 years after intensive chemotherapy and/or radiation therapy for Hodgkin’s disease [85–87]. Most of these cases occurred within 2–10 years following therapy for Hodgkin’s disease, with a median latency of 4–6 years and a plateau of leukemia incidence after 10 years.
Table 24.4
t-MDS/t-AML following the treatment of Hodgkin’s disease
Investigators | No. of patients | Median follow-up (years) | No. with t-MDS/t-AML | Relative risk (95 % confidence interval) | Absolute riska | Actuarial risk | Median latency (range) | |
---|---|---|---|---|---|---|---|---|
Percent | Years | |||||||
Devereux et al. (1990) [68] | 2,676 | NS | 17b | NS | NS | 1.7 | 15 | 79.9 months (35–155) |
Kaldor et al. (1990) [69]c | 29,552 | NS | 149 b | RT 1.0 | NS | NS | NS | |
CT 9.0 (4.1–20) | ||||||||
Cimino et al. (1991) [70] | 947 | 10.5 | 23 | RT + CT 7.7 (3.9–15) NS | NS | 2.8 | 10 | 58 months (13–209) |
3.6 | 15 | |||||||
10.0 | 19 | |||||||
Abrahamsen et al. (1993) [71] | 1,152 | 8 | 9 | 24.3 (11.1–46.2) | 10.4 | 1.5 | 18 | 5.8 years |
Swerdlow et al. (1993) [72] | 2,846 | 6.1 | 16 | 16.0 (9.2–26.0) | 8.7 | NS | NS | |
Dietrich et al. (1994) [73] | 892 | NS | 8 | 27.59 (11.91–54) | 15 | 1.7 | 10 | NS |
1.7 | 15 | |||||||
van Leeuwen et al. (1994) [74] | 1,939 | 9.2 | 31d (12 MDS) | 34.7 (23.6–49.3) | 18.1 | 4.0e | 20 | NS |
48.2 (34.9–64.9) e | ||||||||
Bhatia et al. (1996) [75] | 1,380 | 11.4 | 26 | NS | NS | 2.8 | 14 | NS |
Mauch et al. (1996) [76] | 794 | 11 | 8 | 66.2 (28.1–130.7) | 9.3 | NS | 5 years | |
Wolden et al. (1998) [77] | 694 | 13.1 | 8 | 40.9 (17.7–80.7) | 9.3 | NS | 4.3 years | |
Dores et al. (2002) [78] | 32.591 | NS | 169 | 21.5 (18.3–25.0) | 6.3 | 27.7 | 1–9 | NS |
2.6 | 15–19 | |||||||
Smith et al. (2003) [79] | 306(42–85) | NS | 75 | NS | NS | NS | 62 months (42–85) |
A correlation exists between the amount of chemotherapy and the risk of developing t-AML in individuals treated for Hodgkin’s disease. Also, intensive therapy for Hodgkin’s disease decreased the latent period between the diagnosis of Hodgkin’s disease and the development of leukemia. Intensive chemotherapy is defined as at least six cycles of mechlorethamine, vincristine (Oncovin), procarbazine, and prednisone (MOPP) or an equivalent group of drugs; intensive radiation therapy is defined as either at least total nodal or extended-field irradiation. In one series, patients who received intensive chemo- or radiation therapy, or both, had a mean interval from initiation of therapy to diagnosis of leukemia of 3.4 years, which was significantly shorter than the mean interval of 7.6 years in patients who received less intensive therapy [88]. The National Cancer Institute (NCI) has reported that patients receiving salvage chemotherapy for refractory Hodgkin’s disease have a 10 % incidence of AML, compared with 5 % at 5 years for all Hodgkin’s patients treated with combined modality therapy [51]. A significantly increased risk for t-AML (3.0 %) has also been associated with the escalated-BEACOPP regimen in patients treated by the German Hodgkin Study [87].
Chemotherapy alone especially as combinations containing MOPP or MOPP-like regimens significantly increased the risk of leukemia. Significant reduction in the risk of developing t-MDS/t-AML following the treatment of Hodgkin’s disease observed in the 1980s is largely due to the introduction of adriamycin, bleomycin, vinblastine, and dacarbazine (ABVD) regimen in the treatment of Hodgkin’s disease [70, 74, 85, 89, 90]. For example, a higher long-term leukemia risk has been observed in patients treated with the MOPP regimen, corresponding to 3.4 % as compared to 1.3 % for ABVD followed by high-dose irradiation [86]. This result has been corroborated by the long-term follow-up of 120 patients with nonbulky stage I–IIA Hodgkin lymphoma, treated with 4 cycles of ABVD and limited radiotherapy, where no cases of secondary leukemias have been observed [91].
Concerning the impact of radiation on the risk to develop t-MDS/t-AML in patients with Hodgkin’s disease, most of the studies showed either no or little risk of developing t-MDS/t-AML with radiation therapy alone, even though relatively high doses of radiation therapy are used in the treatment of Hodgkin’s disease [69, 70]. Contrary, Tucker et al. reported an increased risk of t-MDS/t-AML after radiation therapy alone with a relative risk (RR) of 11 % (95 % confidence interval, 1.2–38) [84].
In terms of modality therapy, a case-control study of 1,939 patients found no significant difference in the relative risk of leukemia among patients with Hodgkin’s disease who received combined modality therapy as compared with those who received chemotherapy alone [74, 81], but recent data clearly confirm previous studies [70, 71, 80] that addition of radiation therapy to chemotherapy increases the risk of leukemia, when compared to ABVD alone.
Prior studies identified age at diagnosis as a potential risk factor for development of leukemia [59, 92, 93]. In one series, the cumulative risk of leukemic complications 9 years after beginning chemotherapy for Hodgkin’s disease was 8.6 % for individuals under 40 years of age compared with 40 % for those aged 40 years or older. In another series, the actuarial risk of leukemia was 20.7 % at 7 years after therapy for Hodgkin’s disease patients aged 40 years or older and was approximately 6.6 % at 7 years for all patients [59]. Paradoxically, several comprehensive studies in children showed a significant trend of greater risk with younger age at first treatment when the observed risks at various age groups were compared to the expected risks [71, 72]. A large study by Dores et al. on 32,591 patients with Hodgkin’s disease, out of which 1,111 were observed for 25 years, confirmed this new trend: The relative risk of all secondary cancers taken together decreased significantly with increasing age at diagnosis of Hodgkin’s disease, whereas the absolute excess risks tended to increase [78]. The apparent decline in both parameters observed in the ≥61 age category, though, might have reflected the shorter follow-up in this age group (mean = 4.1 years). Similar trends by age were observed for all solid tumors considered together, acute nonlymphocytic leukemia, and cancers of the digestive tract and lung [78].
Since van Leeuwen et al. suggested in 1987 that splenectomy in Hodgkin’s disease patients may contribute to the risk of leukemia, many studies reported a similar significantly increased risk [69, 73, 81, 94]. Investigators have suggested that the increased risk may be due to loss of splenic immunosurveillance. Others have reported that splenic irradiation is a significant risk factor as well [73]. However, a number of reports also stated that splenectomy did not pose a significant risk for development of leukemia [72, 83, 84].
Non-Hodgkin’s Lymphoma
The risk of therapy-related leukemia in patients treated for NHL has been extensively studied, and Table 24.5 lists some of the recent reports [67, 95–101]. As in Hodgkin’s disease, alkylating agents such as prednimustine, mechlorethamine, procarbazine, and chlorambucil are associated with higher risks of t-MDS/ t-AML [67, 99, 100]. For example, within a cohort study of 11,386 2-year survivors of NHL, 35 patients with t-AML were matched to 140 controls. Of 10,000 NHL patients treated for 6 months with selected regimens including low cumulative doses of cyclophosphamide and followed for 10 years, an excess of four leukemias might be expected [100]. Cyclophosphamide regimens were associated with a small, nonsignificantly increased risk of t-AML (RR = 1.8; 95 % CI, 0.7–4.9). Also, treatment with epipodophyllotoxins, doxorubicin, or bleomycin was not associated with elevated leukemic risk when adjusted for the effects of alkylating agents. The median cumulative dose of epipodophyllotoxins was relatively small: 453 mg for the leukemic group and 833 g for the control cohort [100]. These data are conflicting with the recent report of a British study, which documented 123 second malignancies in 2,456 NHL patients treated between 1973 and 2000 [99]. The relative risk to develop a secondary leukemia was 10.5 [95 % confidence interval (95 % CI), 5.0–19.3]. For cyclophosphamide–hydroxydaunomycin/doxorubicin–Oncovin–prednisone (CHOP), it was 14.2 (95 % CI, 6.8–26.2), and for chlorambucil-treated patients, the relative risk was 19.2 (95 % CI, 9.6–34.3). A significantly increased risk of leukemia was associated with receiving chemotherapy, combined or not with radiotherapy, while no cases of leukemia were observed in patients treated with radiotherapy alone [99].
Table 24.5
t-MDS/t-AML following the treatment of non-Hodgkin’s lymphoma
Investigators | No. of patients | Median follow-up (years) | No. with t-MDS/t-AML | Relative risk (95 % confidence interval) | Actuarial risk | Median latency (range) | |
---|---|---|---|---|---|---|---|
Percent | Years | ||||||
Pedersen-Bjergaard et al. (1985) [95] | 602 | NS | 9 | 76 | 1.8 | 4 | 51 months (26–81) |
6.3 | 7 | ||||||
8.0 | 9 | ||||||
Ingram et al. (1987) [96] | 261 a | 3–9 | 6 | NS | 7.8 | 7 | 3 years |
Travis et al. (1991) [97] | 29,153 | 3.12 | 29 | 2.88 (1.93–4.13) | NS | 61 months (2–160) | |
Travis et al. (1993) [98] | 6,171 | 7.4 | 14 | 4.83 (2.64–8.10) | NS | 7.6 years (2.4–18.2) | |
Smith et al. (2003) [26] | 309 | NS | 69 | NS | NS | 68 months (40–115) | |
Andre et al. (2004) [67] | 2,387 | 6.16 | 7 (fem.) | 19.89 (7.98–40.97) | NS | NS | |
4 (male) | 5.65 (1.54–14.46) | NS | NS | ||||
Mudie et al. (2006) [99] | 2,456 | 7.7 | 9 | 13.1 (6.0–24.9) | 1.5b | 15 | NS |
The role of radiation therapy in leukemogenesis in NHL is unclear. In one study, low-dose total body or hemibody irradiation was more leukemogenic than high-dose irradiation [102]. However, conflicting reports exist concerning the risk associated with high-dose irradiation [32, 100].
An increased risk of secondary malignancies including therapy-related myeloid neoplasms has been identified in patients with indolent NHL, in particular when fludarabine-containing regimens or bone marrow transplantation (BMT) was used. At a median follow-up of 62 months, the cumulative incidence of secondary cancer including t-MDS and t-AML at 12 years was 10.5 % in 563 Italian patients with indolent NHL treated with different regimens, including BMT. Significant risk factors were older age at the time of diagnosis, male sex, and fludarabine-containing therapy [103]. On the other hand, Maudie et al. stated that the relative risk of t-AML was greater with patients who are younger at first treatment [99].
Recently, the Groupe d’Etude des Lymphomes de l’Adulte (GELA) published the results of three large studies on secondary malignancies of 2,837 patients with aggressive NHL treated with the adriamycin–cyclophosphamide–vindesine–bleomycin–prednisone (ACVBP) regimen. Twelve of 81 reported secondary malignomes (14.8 %) were t-MDS/t-AML, which developed within a median time of 40 months from NHL diagnosis (range, 18–100 months). The median survival time from t-MDS/t-AML diagnosis was 1 year (95 % CI, 0.45–1.62). Notably, the standardized incidence ratio (SIR) for t-MDS/t-AML was much higher for females (SIR = 19.9) than for males (SIR = 5.65) [67].
Multiple Myeloma
Some investigators have suggested that acute leukemia, other than plasma cell leukemia, may be part of the natural history of multiple myeloma. However, little evidence exists to support this concept [32, 104]. The typical treatment regimen for multiple myeloma includes prolonged use of alkylating drug therapy, such as melphalan, which has been clearly shown to be leukemogenic. The risk of t-MDS/t-AML in multiple myeloma should therefore be considered to result from its therapy. Table 24.6 lists some of the studies that illustrate the leukemogenic risks in patients with multiple myeloma [62, 105, 106]. All therapies used in these studies included an alkylating agent. The actuarial risk t-MDS/t-AML reported in multiple myeloma was as high as 20 %. Cuzick et al. [105] studied the relationship between the duration and dose of treatment with either melphalan or cyclophosphamide in a total of 648 multiple myeloma patients. Twelve cases of myelodysplasia or acute leukemia were observed in the entire cohort. The duration and cumulative dose of melphalan therapy were the most significant determinant of risk. Cyclophosphamide therapy was not associated with the increased leukemic risk [105]. In another study of 364 multiple myeloma patients, actuarial risk of developing t-AML was 4 % after 3 years and 19.6 % after 4.2 years of alkylating drug therapy (cyclophosphamide, melphalan, carmustine) [106]. No significant difference in risk of leukemia was observed between different alkylators. Another study of 908 myeloma patients receiving alkylating agent therapy found an actuarial risk of t-MDS/t-AML of 2.8 % and 10.1 % after 5 and 10 years, respectively [62]. Seven additional patients developed t-MDS who were not included in the analysis of leukemia risk. Prolonged use of alkylating agents in multiple myeloma poses a clear leukemogenic risk. However, there are no current results from large trials on the cumulative risk of t-MDS/AML in patients with multiple myeloma. In a smaller study, Rund et al. reported that among 96 patients with multiple myeloma, 4 % developed t-MDS/t-AML [107]. Nevertheless, the authors did not provide detailed information, neither on therapy nor on overall outcome of this subgroup. In a recent study by Smith et al., 22 of 306 patients (7.1 %) with t-MDS/t-AML were diagnosed with preceding multiple myeloma. The median latency to the diagnosis of t-MDS/t-AML was 52 months (range 34–72 months) [26].
Table 24.6
Risk of t-MDS/t-AML following treatment of multiple myeloma
Investigators | No. of patients | Median follow-up (years) | No. with t-MDS/t-AML | Median latency | Relative risk | Actuarial risk | |
---|---|---|---|---|---|---|---|
Percent | Years | ||||||
Cuzick et al. (1987) [105] | 648 | 97 months | 12 | 85.5 months | NS | 10 | 8 |
Kyle (1983) [62] | 908 | NS | 17 | NS | NS | 10.1 | 10 |
Bergsagel et al. (1982) [106] | 364 | NS | 14 | NS | 230 | 19.6 | 4.2 |
Smith et al. (2003) [26] | 306 | NS | 22 | 52 months (34–72) | NS | NS |
Ovarian Cancer
Individuals who have received alkylating agent chemotherapy for ovarian carcinoma are at increased risk of acute leukemia with a cumulative risk of 7–10 % at 5–10 years after therapy (Table 24.7) [56, 58, 108–112]. Most of these prior reports studied individuals who received single alkylating agents for prolonged periods. The risk appeared to correlate with the type, dose, and duration of alkylating agents used. In one series of 773 ovarian carcinoma patients, nine of the ten cases of leukemia developed in patients who received more than 700 mg of melphalan [58]. A case-control study identified 101 patients with leukemia among 99,113 survivors of ovarian cancer [ 111]. Radiation therapy alone did not produce a significant increase in risk, as compared with surgery alone. Chemotherapy alone was significantly associated with a relative risk of 12.0 (95 % CI, 4.4–32). The addition of radiation therapy to chemotherapy did not produce any additional risk (RR 9.8 [95 % CI, 3.4–28]). Among various alkylating agents, chlorambucil and melphalan were the most leukemogenic, followed by thiotepa, cyclophosphamide, and treosulfan [111]. Patients who received the combination of doxorubicin and cisplatin also showed an increase in leukemogenic risk (RR 6.5).
Table 24.7
t-MDS/t-AML following therapy for ovarian carcinoma
Investigators | No. of patients | Therapy | Median follow-up (years) | No. with t-MDS/t-AML | Relative risk (95 percent confidence interval) | Median latency (range) | ||
---|---|---|---|---|---|---|---|---|
Actuarial risk | Percent | Years | ||||||
Reimer et al. (1977) [108] | 5,455 | CT ± RT | NS | 13 | 36.1 (19.2–61.8) 171.4 (88.5–299.5) a | NS | 41.5 months (30–90) | |
Pedersen-Bjergaard et al. (1980) [109] | 553 | Dihydroxybusulfan | NS | 7 | 125 | 7.6 | 5 | 50 months |
Greene et al. (1986) [110] | 3,363 | No RT or CT (595) RT (955) CT (1,179) RT + CT (539) | 5.7 | 0 2 21 12 | 93 (61–136) b | 0 0.1 8.6 8.3 | 10 10 10 10 | (21–58) NS |
Kaldor et al. (1990) [111]c | 99,113 | Surgery RT CT RT + CT | NS | 6 15 41 39 | 1.0 1.6 (0.51–4.8) 12.0 (4.4–32) 9.8 (3.4–28) | NS | 4–5 years | |
Travis et al. (1999) [112]c | 28,971 3 | RT CT d | NS NS | 1 65 | 0.4 (0.04–3.5) 6.5 (2.3–18.5) | NS | 3.3 years | |
Smith et al. (2003) [34] | 306 | RT + CT CT (12) RT (1) RT + CT (2) | NS | 25 15 | 8.1 (2.6–25.6) NS | NS | 53 months (18–74) |
Many of the earlier mentioned drugs are no longer in common use, and the trend in treatment for ovarian cancer today is toward pulse combination chemotherapy rather than more continuous single-agent therapies. Platinum-based combinations are often used for advanced ovarian cancer. A case-control study of 28,971 women with invasive ovarian cancer receiving alkylating agents including platinum compounds found that 90 patients developed t-MDS/t-AML resulting in a relative risk of 6.5 (95 % CI, 2.3–18.5) [112]. If patients received a platinum agent as the principal alkylating drug, the relative risk was 4.0 (95 % CI, 1.4–11.4), which was considerably lower than the risk after treatment with melphalan (RR 20.8; 95 % CI, 6.3–68.3). The relative risk with carboplatin and cisplatin was 6.5 (95 % CI, 1.2–36.6) and 3.3 (95 % CI, 1.1–9.4), respectively. A clear increase in the risk occurred with higher cumulative dose and longer duration of platinum treatment. Added risk occurred, when platinum compounds were used in combination with radiation or other alkylating agents such as melphalan. In this series [112], 42 % of the women who developed t-MDS/t-AML had early-stage ovarian cancer emphasizing the importance of risk–benefits evaluation of the adjuvant therapies in early-stage disease.
Paclitaxel is often used in combination with platinum, but the leukemogenic risk of paclitaxel has not yet been defined. Sixty-eight patients with ovarian, tubal, or peritoneal adenocarcinoma (26 platinum-resistant/-refractory and 42 platinum-sensitive) were treated with gefitinib in combination with paclitaxel and carboplatin. During treatment, two patients developed t-MDS, one patient t-AML, and another patient developed t-MDS 33 months after treatment discontinuation [113]. The Roswell Park Cancer Institute (RPCI) retrospectively reviewed 10 t-MDS/t-AML (2 MDS, 8 AML) patients with preceding ovarian cancer. All had received platinum chemotherapy, whereas 8 had also received taxanes (80 %), 4 cyclophosphamide (40 %), and 3 adriamycin (30 %). The median interval from diagnosis of ovarian cancer to t-MDS/t-AML was 5.5 (range, 3–11) years, and the median overall survival for all 10 patients after diagnosis of t-MDS/t-AML was 4 months (range, 0.5 months to 9.5 months). The authors concluded that patients with t-MDS/t-AML following therapy for ovarian cancer have unfavorable cytogenetic abnormalities, including complex karyotypes and involvement of chromosomes 5 and 7, which are typical findings following alkylating agent therapy, and that these patients have a very poor prognosis [114].
A randomized, phase III noninferiority trial from the German Ovarian Cancer Study Group compared outcome and toxicity of paclitaxel plus cisplatin with paclitaxel plus carboplatin in 798 patients with advanced ovarian cancer (International Federation of Gynecology and Obstetrics stage IIB–IV). Notably, no secondary leukemias were observed during a follow-up of approximately 49 months [115].
Testicular Cancer
Approximately 80 % of patients with testicular cancer can be cured with combination chemotherapy regimens, even those patients with an advanced stage of disease. Increasingly, long-term complications of therapy are emerging as an important problem in testicular cancer patients including t-MDS/t-AML, but the overall risk appears to be relatively low (Table 24.8) [116–121]. No secondary leukemias have been reported following four cycles of standard dose cisplatin, vinblastine, and bleomycin (PVB) combination chemotherapy, and only one case was reported after prolonged therapy with PVB with a total dose of 1.3 g of cisplatin over 12 months [122]. Other retrospective analyses have also shown no increased risk of t-AML after PVB therapy [116, 118, 123, 124]. In the 1980s, the epipodophyllotoxin derivative etoposide was introduced in the first-line treatment of germ cell tumors resulting in excellent therapeutic outcome in patients with advanced disease [125]. An early report of chemotherapeutic regimens with etoposide, cisplatin, bleomycin (PEB) found a markedly increased risk of AML (RR 336, 95 % CI, 92–861) [116]. Since this initial report, several studies have addressed the leukemogenic potential of etoposide-containing chemotherapy in germ cell tumors. A cumulative etoposide dose of 2 g/m [2] or less conferred a relatively low incidence of t-AML (approximately 0.5 % after 5 years) [116–119, 126, 127]. In contrast, a cumulative etoposide dose greater than 2 g/m [2] resulted in an overall incidence of 2 % (range 0.8–8 %) at 5 years [116, 126–129]. The high relative risk in the initial report may have resulted from the very high doses of cisplatin in addition to high cumulative dose of etoposide administered to these individuals [116]. A risk–benefit analysis for germ cell tumor patients estimated that etoposide-containing regimens like PEB (compared to PVB) at standard doses can cure approximately 20 additional individuals per every case of t-AML [130], suggesting that the benefit of standard dose etoposide-containing regimens outweighs the risk of developing t-AML in those with advanced germ cell tumors. However, less aggressive therapies should be considered when the disease has been diagnosed at an earlier stage.
Table 24.8
t-MDS/t-AML following testicular cancer therapy
Investigators | No. of patients | Therapy | Median follow-up (years) | No. with t-MDS/t-AML | Relative risk (95 % confidence interval) | Actuarial risk | |
---|---|---|---|---|---|---|---|
Percent | Years | ||||||
Pedersen-Bjergaard et al. (1991) [116] | 212 127 | Etoposide No etoposide | NS | 5 0 | 336 (92–861) | 4.7 | 5.7 |
Bajorin et al. (1993) [117] | 503 | Etoposide | NS | 6 | NS | <1 | |
Bokemeyer et al. (1993) [118] | 1,025 | Surgery (22 %) RT (32.6 %) CT (40.5 %) | 5 years (1–20) | 0 | NS | NS | |
Nichols et al. (1993) [119] | 538 | RT + CT (4.8 %) Etoposide | NS | 2 | 66 (8–238) | NS | |
van Leeuwen et al. (1993) [120] | 1,909 | Surgery RT CT RT + CT | 7.7 years | 0 3a 1a 2a | 0 5.2 (1.1–15.1) 20.0 (0.51–111) 66.7 (8.1–241) | NS | |
Howard et al. (2006) [79] | 42,722 | Surgery (17 %) RT (38 %) CT (14 %) RT + CT (4 %) Unknown (27 %) | NS | 53 | 2.1 (0.3–2.9) | 0.23b | 30 |
A large study performed by the National Cancer Institute on 42,722 1-year survivors of testicular cancer revealed that 89 patients developed secondary leukemia with an excess absolute risk (EAR) of 10.8 per 100,000 person-years (PY) (95 % CI, 7.6–14.6) and an excess relative risk (ERR) of 1.6 (95 % CI, 1.0–2.2). Altogether, the excess cumulative leukemia risk was approximately 0.23 % by 30 years after testicular cancer diagnosis. Moreover, in multivariate analyses, t-AML risk was higher among patients whose initial management included chemotherapy compared to those receiving radiotherapy alone [79].
Extragonadal germ cell tumors comprise only 2–3 % of all germ cell tumors. Recently, 635 patients with extragonadal germ cell tumors were analyzed for the incidence of secondary malignancies [131]. Seventeen hematologic disorders were identified among 287 individuals with primary nonseminomatous mediastinal germ cell tumors. None of either 283 individuals with retroperitoneal germ cell tumors or 51 individuals with mediastinal seminomas developed a hematologic disorder. All of the 17 cases showed features being typical of hematological disorders that are associated with nonseminomatous mediastinal germ cell tumors, such as isochromosome of the short arm of chromosome 12 [i(12p)], but not typical of t-MDS/t-AML. This study suggested that apart from a recognized occurrence of hematologic malignancies associated with germ cell tumors, the risk of developing therapy-related leukemias appeared to be low in those with extragonadal germ cell tumors treated with cisplatin-based chemotherapy (median follow-up of 55 months) [131, 132].
Breast Cancer
Increasing numbers of individuals with early-stage breast cancer are receiving adjuvant chemotherapy containing potential leukemogenic agents such as cyclophosphamide and anthracyclines. The Milan Cancer Institute reported a study of 845 women who were included in two consecutive cyclophosphamide, methotrexate, and 5-fluorouracil (CMF) adjuvant trials. Women were treated either with surgery alone or surgery followed by 6–12 cycles of CMF. After a median follow-up in excess of 10 years, no cases of t-AML were observed [133]. Similarly, the Eastern Cooperative Oncology Group (ECOG) has reported that the risk of t-MDS/t-AML among 2,638 women receiving standard dose cyclophosphamide-containing adjuvant chemotherapy was not much higher than in the general population [134]. The addition of anthracyclines, such as doxorubicin or mitoxantrone either with or without radiation therapy may, however, slightly increase the risks. Fourteen cases of t-AML were observed among 1,107 patients, who were treated with 5-fluorouracil, doxorubicin, and cyclophosphamide (FAC) either with or without other agents in an adjuvant or neoadjuvant setting [135]. The 10-year estimated leukemia rate was 1.5 % (95 % CI, 0.7–2.9) for all patients, 2.5 % (95 % CI, 1.0–5.1) for the radiation and chemotherapy group, and 0.5 % (95 % CI, 0.1–2.4) for the chemotherapy only group. These differences were statistically significant.
Furthermore, two recent studies on breast cancer patients treated with epirubicin-based regimens showed a relatively small risk of t-MDS/t-AML. Campone et al. reported 6 cases (0.02 %) of t-AML in 3,653 patients with operable breast cancer treated with the 5-fluorouracil, epirubicin, and cyclophosphamide (FEC) regimen in eight French Adjuvant Study Group trials. The median follow-up was 104 months. After 9 years, the risk of developing leukemia was 0.34 % (95 % CI, 0.11–0.57) in epirubicin-exposed patients [136]. Praga et al. reviewed 7,110 patients with early breast cancer that were treated with epirubicin-containing regimens (92 % of whom also received cyclophosphamide), in 19 randomized trials [137]. The 8-year cumulative probability of t-MDS/t-AML was 0.55 % (95 % CI, 0.3–0.78). Moreover, the risk of developing t-MDS/t-AML increased in relation to planned epirubicin dose per cycle, planned epirubicin dose intensity, and administered cumulative doses of epirubicin and cyclophosphamide [137].
The NCI very recently analyzed the incidence of t-AML by age and stage at diagnosis of breast cancer in 420,076 female patients [138]. In turn, there was a significant age-dependent risk of a subsequent diagnosis of t-AML in women younger than 50 years old (RR = 4.14) and women 50–64 years old (RR = 2.19), when compared with the expected incidence of t-AML. There was also a significant stage-dependent increase in risk of subsequent t-AML in younger women with stage III disease, when compared with stage I disease (RR = 2.92), and to a lesser extent in middle age women (RR = 2.24), but not in older women (RR = 0.79). The authors concluded that younger age and stage III disease at the time of breast cancer diagnosis were associated with increased risk of a subsequent diagnosis of t-AML. This association might be explained by either greater chemotherapy exposure or an interaction between therapy and genetic predisposition [138].
Several studies reported an increased risk of t-MDS/t-AML after the use of G-CSF, especially following high-dose regimens [139–141]. A case-control study from France compared 182 patients, who developed t-AML after breast cancer treatment, with 534 matched controls. The relative risk of leukemia was significantly increased after chemotherapy that included a topoisomerase-II inhibitor and was higher after mitoxantrone (RR = 15.6) than after anthracyclines. Moreover, the risk was significantly increased up to 3.9-fold after breast radiotherapy. Interestingly, the risk was also significantly increased among those who received G-CSF (RR = 6.3), even when controlling for chemotherapy doses [138]. In another study, 5,510 women older than 65 years, who received chemotherapy for stage I to III breast cancer, were analyzed. Sixteen (1.77 %) of the 906 patients who also received G-CSF or GM-CSF developed t-AML compared to 1.04 % of those who did not receive myeloid growth factors. The hazard rate was 2.59 (95 % CI, 1.30–5.15) for the development of leukemia within 4 years [141]. Six trials completed by the National Surgical Adjuvant Breast and Bowel Project corroborated this hypothesis [140].
The influence of adjuvant radiation therapy (RT) on the leukemic risk was analyzed in a large, institutional study of the Institut Curie in Paris, and 16,705 patients with nonmetastatic breast cancer were retrospectively analyzed. RT was given to 13,472 of these patients, whereas 3,233 patients received no RT. At a median follow-up of 10.5 years, 709 patients were diagnosed with secondary malignancies (113 in the non-RT and 596 in the RT group), but radiotherapy did not increase the risk of secondary leukemia in this study [142]. On the other hand, a large study by Smith et al. stated an increased RR of 2.38 after breast radiotherapy [140].
Autologous Hematopoietic Stem Cell Transplantation
High-dose therapy (HDT) followed by autologous hematopoietic stem cell transplantation (ASCT) is increasingly used to treat various malignancies including Hodgkin’s disease, NHL, germ cell tumor, breast cancer, and multiple myeloma. In the majority of cases, HDT is utilized in an attempt to salvage relapsed or refractory diseases or to consolidate remissions in patients with high risk of recurrence of disease. The short-term treatment-related mortality and morbidity of HDT-ASCT have improved significantly with modern supportive care measures and better patient selection. However, during the last decade, the increasing risk of t-MDS/t-AML following high-dose chemotherapy and ASCT has emerged as one of the most significant long-term complications of this treatment.
For Hodgkin’s disease and NHL patients, a study of HDT-ASCT from New Castle found that the cumulative risk for t-MDS/t-AML was only 1.1 % at 20 months in the cohort of patients who were predominantly transplanted in first relapse [143]. In contrast, HDT-ASCT for 76 Hodgkins’s disease and NHL patients was associated with an actuarial risk of 24.3 % at 43 months [144]. The reason for these variations is a reflection of many differences between these studies, such as the primary diagnoses, the duration and kinds of pretransplant treatments, type of priming to collect the stem cells and conditioning used to treat the cancer, and the duration as well as methods of follow-up of the patients. For example, the overall outcome of ASCT was equivalent between the two sources of hematopoietic stem cells, bone marrow or peripheral blood. However, several studies suggested that the risk of t-MDS/t-AML was higher following ASCT using stem cells harvested from peripheral blood (after chemotherapy priming and growth factors) compared to those from the bone marrow [145–147]. Whether the differences in risk were due to biological differences of the stem cells themselves, toxicity of the priming used to harvest the stem cells, and/or other unknown factors are not clear.
In a large study on 1,732 consecutive patients with Hodgkin’s disease treated at the British Columbia Cancer Agency, 202 patients underwent ASCT. The median follow-up duration was 9.8 years for the whole cohort, 9.7 years for those patients treated with conventional therapy, and 7.8 years from AHSCT. Eighteen of the 1,732 patients developed t-MDS/t-AML. Fourteen of the 18 t-MDS/t-AML patients were treated with conventional therapy alone and four occurred after ASCT. Importantly, most of these patients had previously received radiotherapy and chemotherapy (83 %, including MOPP in 72 %). For the patients undergoing ASCT, the median interval from ASCT to t-MDS/t-AML was 3.9 years (range, 2.1–4.2 years). The 15-year cumulative incidence of developing t-MDS/t-AML was 1.1 % (95 % CI, 0.6–1.8) for patients treated with conventional therapy alone and 3.6 % (95 % CI, 0.9–9.6) for those undergoing ASCT. Seventeen patients died within a median of 5.5 months (range, 0–18.5 months) from the diagnosis of t-MDS/t-AML. A significantly increased risk of t-MDS/t-AML was also seen for patients older than 35 years and stage III/IV [49].
The use of dose-intensive therapy has clearly benefited many patients who were otherwise incurable; however, the risk of t-MDS/t-AML after ASCT raises a serious concern. Table 24.9 summarizes the data from recently reported studies [129, 144–161]. The reported actuarial risk of t-MDS/t-AML varies significantly depending on the series. For example, in a study of 229 breast cancer patients, no cases of t-MDS/t-AML occurred with a median follow-up of 42 months after ASCT [158]. Interestingly, the risk of t-MDS/t-AML appeared to be relatively low after HDT-ASCT in patients with nonhematologic malignancies such as breast cancer and germ cell tumors [129, 157, 158]. However, this may reflect the paucity of pretransplant therapy given to many breast cancer patients or the younger age among those with germ cell tumors.
Table 24.9
t-MDS/t-AML following autologous transplantation
Investigators | Primary diagnosis | No. of patients | No. with t-MDS/t-AML | Actuarial risk | Median latency | ||
---|---|---|---|---|---|---|---|
Percent | Year | Primary diagnosis | HDT-ASCT | ||||
Darrington et al. (1994) [148] | HD NHL | 249 262 | 6 6 | 4 | 5 | 80 months | 44 months |
Bhatia et al. (1996) [145] | HD NHL | 82 176 | 4 6 | 13.5 | 6 | NS | 4 months |
Pedersen-Bjergaard et al. (1997) [144] | HD NHL | 27 49 | 4 2 | 24.5 | 3.6 | 16–74 months | 4–43 months |
André et al. (1998) [146] | HD | 467 | 8 | 4.3 | 5 | NS | |
Friedberg et al. (1999) [149] | NHL | 552 | 41 | 19.8 | 10 | NS | 47 months |
Brown et al. (2005) [150] | NHL | 552 | 68 | 14.2 | 15 | NS | 9.5 years |
Sobecks et al. (1999) [151] | HD NHL | 79 103 | 5 1 | NS | 5.5 years | 1.5 years | |
Milligan et al. (1999) [152] | HD NHL | 1,715 3,168 | 28 37 | 4.6 3.0 | 5 5 | NS NS | |
Harrison et al. (1999) [153] | HD | 595 | 8 | 3.1 | 5 | NS | |
Krishnan et al. (2000) [147] | HD NHL | 218 394 | 11 11 | 8.6 | 6 | 3.8 years | 1.9 years |
Micallef et al. (2000) [154] | NHL | 230 | 27 | 14.2 | 5 | 9.1 years | 4.4 years |
Metayer et al. (2003) [155] | HD NHL | 955 1,784 | 19 37 | 7.1 8.9 | 7 7 | NS NS | |
Lenz et al. (2004) [156] | NHL | 195 | 5 | 3.8 | 5 | NS | 44 months |
Laughlin et al. (1998) [157] | Breast cancer | 864 | 5 | 1.6 | 4 | NS | |
Martinez-Climent et al. (2000) [158] | Breast cancer | 229 | 0 | 0 | NS | ||
Kollmannsberger et al. (1998) [129] | Germ cell tumors | 302 | 6 | 1.3 | 4.3 | NS | |
Govindarajan et al. (1996) [159] | Multiple myeloma | 71a 117b | 0 7 | 0 12 | NS |
Similar to the results of conventional therapy, increased risk of t-MDS/t-AML with HDT-ASCT correlated with increasing age of the patient and increasing cumulative dose of alkylating agents given before the ASCT. Age at the time of HDT-ASCT was an independent and significant risk factor in a study by the EBMTR [152]. Other significant risk factors included a lengthy duration of pretransplant therapy, a prolonged interval from primary diagnosis to ASCT, a number of prior relapses, treatment with an alkylating agent, and chemotherapy with MOPP and with lomustine.
The leukemogenic potential of radiation therapy alone has been controversial. Several studies have shown no increased risks in the setting of conventional treatment. However, in the setting of HDT-ASCT, previous radiation therapy, in particular pelvic radiation, was a significant risk factor [162]. In addition, TBI used as part of the conditioning regimen for ASCT appears to increase the risk of t-MDS/t-AML [148]. At Dana Farber Institute, Hodgkin’s disease and NHL patients transplanted after receiving TBI-containing regimen had a very high cumulative risk of t-MDS/t-AML: 18 % at 6 years and 19.8 % at 10 years [149, 162]. In the previous report by Friedberg et al. [149], 41 cases of 552 NHL patients developed t-MDS/t-AML after uniform conditioning with cyclophosphamide and TBI followed by ASCT. The median follow-up was 5 years (Table 24.9). In a recently updated follow-up of these patients, altogether, 68 cases (11 %) of t-MDS/t-AML have been observed within a median of 9.5 years. The incidence of t-MDS/t-AML was 14.2 % at 15 years, and an expected number of cases with 0.27 per 100,000 person-years at risk, with 68 observed [150].
In contrast, a similar group of patients at the same institution who were treated only with a chemotherapy conditioning regimen had a lower risk of 4.2 % at 5 years. Multiple transplants in patients with multiple myeloma, Hodgkins’s disease, or NHL resulted in an enhanced risk. Also, the German Low Grade Lymphoma Study Group recently reported on 431 patients with indolent lymphoma who were randomly assigned after a cyclophosphamide, doxorubicin, vincristine, and prednisone-like induction therapy regimen to myeloablative radiochemotherapy followed by ASCT (195 patients) or IFN-alpha maintenance (236 patients). After a median follow-up of 44 months, 2.6 % of the patients developed t-MDS after ASCT, and two of these patients transformed into t-AML. Accordingly, the estimated 5-year risk for t-MDS/t-AML after ASCT was 3.8 %. In contrast, in the IFN-alpha arm, the 5-year risk of for t-MDS/t-AML was 0 %. Though, no data about progression-free survival especially of the cohort receiving IFN-alpha maintenance were included [156].
Clinical presentation of t-MDS/t-AML following HDT-ASCT is usually similar to other causes of t-MDS/t-AML. However, the bone marrow following HDT-ASCT in some patients can be hypoplastic and fibrotic with a low percentage of blasts despite refractory cytopenias. Dysplastic changes are usually observed. Cytogenetic evaluation of the bone marrow may be required for the definitive diagnosis of t-MDS/t-AML in these cases. Similar to most causes of t-MDS/t-AML, cytogenetic abnormalities include del(-7/7q) and/or del(-5/5q), or a complex karyotype. The leukemia that develops in those individuals exposed to a DNA topoisomerase II inhibitor such as etoposide prior to ASCT often has a balanced translocation that involves either chromosome 11q23 or 21q22 [147]. Some investigators advocate cytogenetic screening of the bone marrow or examining for clonality such as X-chromosome inactivation using the human androgen receptor gene prior to harvesting stem cells for HDT-ASCT especially in heavily pretreated patients to avoid transplanting patients with preexisting t-MDS [163]. The latent period to t-MDS/t-AML after HDT-ASCT has been as short as 4–8 months, and more than 25 % of cases occurred within 12 months. In these cases, the malignant clone might have been established by therapy prior to ASCT. The survival of these patients is very poor. Allogeneic BMT in these cases is usually unsuccessful.
Myeloproliferative Neoplasms
Individuals with polycythemia vera (PV) have an increased incidence of AML as part of the natural history of their disease. However, the risk of AML is greater if the individuals with PV are treated with either chlorambucil or 32P as compared to phlebotomy alone [57]. The Polycythemia Vera Study Group (PVSG) has found that leukemia developed in 1.5 % of patients in the phlebotomy treatment group, in 10 % of the patients in the 32P group, and in 13 % of the chlorambucil treatment group among 431 previously untreated patients with a maximum follow-up of 18 years (PVSG-01 trial). A large number of individuals withdrew from the phlebotomy alone treatment arm, which probably resulted in the underestimation of the risk of leukemia in the phlebotomy only arm. Recent “intention to treat” analysis revealed a slightly higher incidence of leukemia (3.7 %) [164] in the phlebotomy only treated patients. The relative risk of leukemia developing in PV patients was four times greater with daily doses of chlorambucil above 4 mg than with doses of 4 mg or less, and the leukemia risk was five times greater when the drug was given for more than 50 % of the days of the study compared with 50 % or less [57]. In search of a less mutagenic agent, PVSG-08 trial evaluated hydroxyurea in the treatment of PV [164]. Updated analysis revealed that the hydroxyurea group (median follow-up of 8.6 years) showed a tendency to the development of more cases of acute leukemia (9.8 % vs. 3.7 % in the historical control group of phlebotomy only arm from the PVSG-01 trial). This result, however, is not statistically significant.
In another study, a slightly lower incidence of t-AML (5.6 %) was observed in 71 PRV patients treated with hydroxyurea (median and maximum treatment duration of 7.3 and 15 years, respectively) [165]. Other studies have also suggested that the therapy with hydroxyurea may increase the tendency of PV to transform to t-AML [166, 167]. Interestingly, in a study from the Hadassah Hospital of 96 patients with t-MDS/t-leukemia (66 patients had t-AML, 23 had t-MDS, and seven had a phenotype of t-ALL), 13 % had a preceding MPN [107]. Of interest, our group recently detected deletions of the long arm of chromosome 7 in bone marrow during the blast phase of 6 of 45 patients (13 %) with prior MPN, who were pretreated with hydroxyurea and/or alkylating agents. Also, del(17p) occurred in 5 of 45 cases pretreated with hydroxyurea [168].
Specific Therapies Associated with the Development of T-Mds/T-Aml
Chemotherapeutic Drugs
Alkylating agents have long been among the most frequently used chemotherapeutic agents and have been clearly shown to cause second malignancies, especially leukemias. These agents interact with DNA in a variety of ways: monoadduct formation, inter- and intrastrand cross-links, as well as alkylation of free DNA bases. These actions not only can lead to cell death, but also can cause termination of DNA replication and chromosome loss, leading to mutagenesis and particularly leukemogenesis. Alkylation events can also change the stereometric configuration of DNA bases, causing them to mispair resulting in single base mutations. Many of the alkylating agents have been clearly implicated in leukemogenesis. These include nitrogen mustards, chlorambucil, cyclophosphamide, and melphalan, as well as nitrosoureas (BCNU, CCNU, and methyl CCNU), busulfan, platinum compounds, and the nonclassic alkylating agent, procarbazine. Virtually every alkylating agent has been implicated in the development of leukemia.
Alkylating agents are probably the most potent chemotherapeutic inducers of leukemia; more than 85 % of patients who developed chemotherapy-related leukemia had received an alkylator [169]. Melphalan, chlorambucil, or cyclophosphamide was the offending agent in nearly 65 % of patients. Alkylating agents may be associated with varying risks of leukemogenesis. For example, one study compared the rates of mutagen-related leukemia in ovarian cancer patients treated with either melphalan or cyclophosphamide, and found that melphalan may be a more potent leukemogen than cyclophosphamide. This may mean that different mutagenic potentials exist within broad groups of antineoplastic agents [110].
The nitrosourea compounds including semustine (methyl-CCNU) are mechanistically related to the alkylating agents, and they are leukemogenic in humans. Analysis of nine prospectively controlled trials comparing the combination of methyl-CCNU plus 5-FU with other therapies without methyl-CCNU in the surgical adjuvant treatments of gastric, colon, and rectal cancers (more than 3,600 patients) showed that the combined therapy including methyl-CCNU was associated with a greater relative risk of 14.4 and a 6-year actuarial risk of 4 % of developing either t-MDS or t-AML. The absolute risk was 2.3 cases/1,000 persons/year. Another chemotherapeutic agent, procarbazine, is often used in combination chemotherapy for Hodgkin’s disease. The drug is a strong mutagen and is leukemogenic in monkeys [170], and probably in humans.
Purine and pyrimidine antimetabolites have rarely been associated with the development of leukemia in either humans or experimental animals [4, 171, 172]. As mentioned previously, prolonged treatment with hydroxyurea in PV patients has been associated with slightly higher incidence of AML. Adriamycin and bleomycin have only rarely been associated with secondary leukemia [85, 173]. In a large series of Hodgkin’s disease patients, t-AML developed in 17 of 606 patients who received combined chemo- and radiation therapy that included an alkylating agent or procarbazine, or both; no leukemias developed in 84 patients who received radiation therapy and the combination of ABVD [173]. Few cases of t-AML have been reported in patients who have received ABVD in combination with radiation therapy.
Recent data from more than 170,000 patients indicated that organ transplantation is associated with a significantly increased risk for t-AML and that the immunosuppressive purine antimetabolite, azathioprine, which is frequently used in recipients of organ transplantation, might play a role in the development of t-MDS/t-AML [174].
Several studies have reported t-AML in patients treated with a cytostatic drug not belonging to the alkylating family of agents. These include drugs targeting DNA-topoisomerase II, such as epipodophyllotoxins (etoposide and teniposide), doxorubicin, 4-epidoxorubicin, mitoxantrone, razoxane, and bimolane. At the molecular level, these compounds act by formation of stabilized topoisomerase II-DNA complexes, which inhibit DNA repair and produce single- and double-strand DNA breaks. These drugs are important components of modern-day chemotherapy regimens for many tumors, such as testicular cancer, ALL, NHL, lung cancer, and many others. Razoxane and bimolane, which have been used in the treatment of psoriasis, have also been demonstrated to be leukemogenic. Those patients in whom t-AML develops after therapy with DNA-topoisomerase II inhibitors often have acute leukemia with no t-MDS phase [11, 12], and a short latency period, in contrast to alkylating agent-induced AML.
The predominant chromosomal aberrations associated with t-AML after therapy with DNA-topoisomerase II inhibitors are balanced translocations involving either chromosome 11q23 or 21q22. Less frequently, other balanced aberrations, such as inv(16), t(8;16), t(15;17), and t(6;9), have been observed. The t(15;17) has been found in patients who were treated in China for psoriasis with bimolane [175].
The DNA-topoisomerase II inhibitors are increasingly cited as leukemogenic agents, but their precise impact on leukemogenesis requires further study. Therapy-related leukemias observed in these individuals are not restricted to the myeloid lineage or to the exclusive use of high-dose etoposide. Occasionally, ALL is an observed second malignancy in these patients. The increased leukemic risk may be directly associated with the cumulative exposure to this class of agents.
Carcinogens in our environment including benzene and other chemical solvents, petrol products, and insecticides are leukemogenic [52, 54, 55, 176]. Humans chronically exposed to benzene have a 5- to 20-fold excess risk of AML compared with nonexposed individuals [52, 176]. Most t-AML patients have had contact with benzene for 1–15 years with a mean of 9.7 years, and many have 6 months to 6 years of pancytopenia before the development of acute leukemia. Many similarities are shared between the leukemia that develops after benzene exposure and the one that develops after chemotherapy. Both have a similar latent period, a t-MDS phase, a specific karyotypic abnormality, and a failure to respond to leukemic treatment.
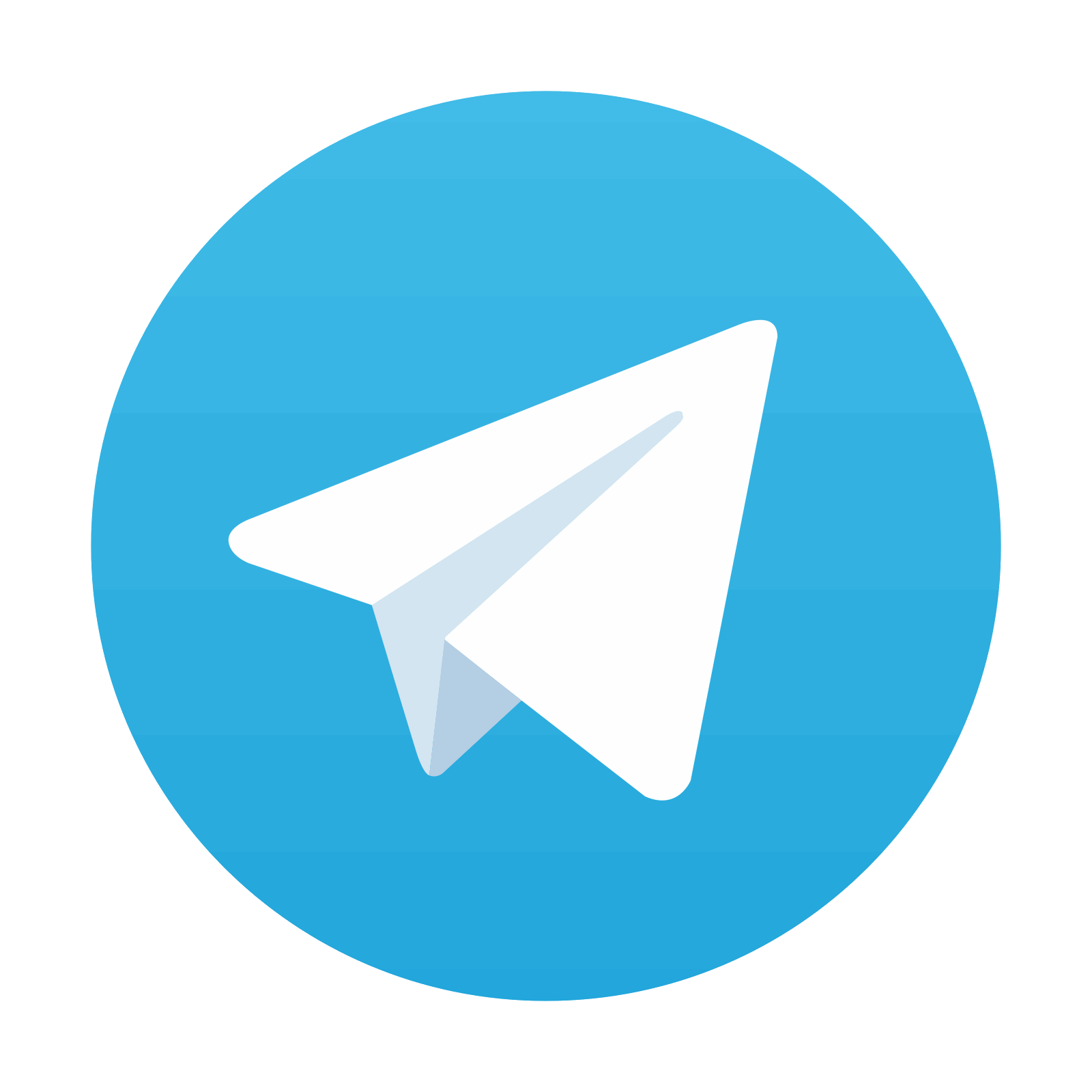
Stay updated, free articles. Join our Telegram channel

Full access? Get Clinical Tree
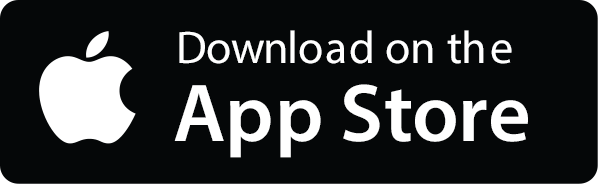
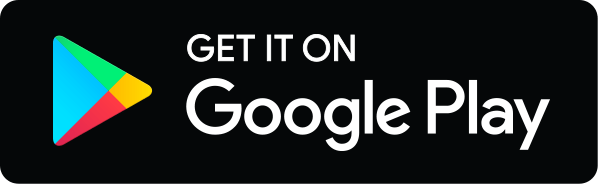