Therapeutic gene editing is significant for medical advancement. Safety is intricately linked to the specificity of the editing tools used to cut at precise genomic targets. Improvements can be achieved by thoughtful design of nucleases and repair templates, analysis of off-target editing, and careful utilization of viral vectors. Advancements in DNA repair mechanisms and development of new generations of tools improve targeting of specific sequences while minimizing risks. It is important to plot a safe course for future clinical trials. This article reviews safety and specificity for therapeutic gene editing to spur dialogue and advancement.
Key points
- •
Safety of gene editing is closely tied to specificity.
- •
Careful design of gene editing tools can improve specificity and thus safety.
- •
A high degree of specificity is possible with the new generation of targeted nucleases.
- •
Assessing the impact of gene therapy tools during their design, study, and clinical use is essential.
Introduction
Therapeutic gene editing is advancing at an ever-increasing pace. As the list of diseases that can be treated or potentially cured with gene editing grows, it is imperative to dedicate time and energy to the topics of the safety and specificity of these technologies. This article is dedicated to a discussion of this topic in a broad sense with examples to detail how these issues affect various aspects of modifying the genetic code of patients.
As discussed in the opening article of this issue, expectations have always been high for the potential of gene therapy but early forays into its clinical application had unexpected consequences. (See Kohn DB’s article, “ Historical Perspective on the Current Renaissance for Hematopoietic Stem Cell Gene Therapy ,” in this issue.) These early setbacks in the implementation of gene therapy caused the research community and the public at large to take pause and consider the safety of this fledgling field. All involved realized that this was new and unexplored territory. Unexpected consequences can and do occur with new medical technologies. It is important to remember that expectations today may be higher for these pursuits than previous eras of medical exploration. Modifying genetic code is fundamentally different from the study of chemicals to kill a particular bacterial strain or slow the growth of a tumor. Rather than externally manipulating the biology of organisms or aberrant cells, the aim is to alter the blueprint of the disease-associated cells to restore or modify their function. Like a surgeon pinpoints a physical defect based on accepted anatomical function and makes repairs, so too are oncologists starting with a map (the normal genome) and working to correct errors. Precision and safety will be expected and demanded. Trial and error will only be acceptable to a point.
Before proceeding, a brief discussion of the stewardship of the human genome is prudent. The most fundamental elements of what defines being human are beginning to be modified. This requires an enormous amount of public support and trust. Although there is clearly support for the treatment and cure of genetic diseases, history shows that these technologies can also be misused. Part of safely developing these tools is considering the consequences of their misapplication. The pursuit or even the perception of the application of gene therapy for eugenics or genetic discrimination could be devastating. Care should be taken in the selection of disease targets and even in the words chosen to describe the diseases to be treated. Respect should be given to historic and cultural diversity. Maintaining a sense of transparency and being open to discussion of the practical impacts of genetic modifications are important elements to maintaining the integrity of gene therapy.
Introduction
Therapeutic gene editing is advancing at an ever-increasing pace. As the list of diseases that can be treated or potentially cured with gene editing grows, it is imperative to dedicate time and energy to the topics of the safety and specificity of these technologies. This article is dedicated to a discussion of this topic in a broad sense with examples to detail how these issues affect various aspects of modifying the genetic code of patients.
As discussed in the opening article of this issue, expectations have always been high for the potential of gene therapy but early forays into its clinical application had unexpected consequences. (See Kohn DB’s article, “ Historical Perspective on the Current Renaissance for Hematopoietic Stem Cell Gene Therapy ,” in this issue.) These early setbacks in the implementation of gene therapy caused the research community and the public at large to take pause and consider the safety of this fledgling field. All involved realized that this was new and unexplored territory. Unexpected consequences can and do occur with new medical technologies. It is important to remember that expectations today may be higher for these pursuits than previous eras of medical exploration. Modifying genetic code is fundamentally different from the study of chemicals to kill a particular bacterial strain or slow the growth of a tumor. Rather than externally manipulating the biology of organisms or aberrant cells, the aim is to alter the blueprint of the disease-associated cells to restore or modify their function. Like a surgeon pinpoints a physical defect based on accepted anatomical function and makes repairs, so too are oncologists starting with a map (the normal genome) and working to correct errors. Precision and safety will be expected and demanded. Trial and error will only be acceptable to a point.
Before proceeding, a brief discussion of the stewardship of the human genome is prudent. The most fundamental elements of what defines being human are beginning to be modified. This requires an enormous amount of public support and trust. Although there is clearly support for the treatment and cure of genetic diseases, history shows that these technologies can also be misused. Part of safely developing these tools is considering the consequences of their misapplication. The pursuit or even the perception of the application of gene therapy for eugenics or genetic discrimination could be devastating. Care should be taken in the selection of disease targets and even in the words chosen to describe the diseases to be treated. Respect should be given to historic and cultural diversity. Maintaining a sense of transparency and being open to discussion of the practical impacts of genetic modifications are important elements to maintaining the integrity of gene therapy.
Safety
Safe manipulation of the human genome is paramount to gene therapy because the intended effect of gene therapy is a permanent modification of cell function. Thus, unintended modifications that alter cell function may have long-lasting consequences.
The last decade has seen the rapid introduction of new tools, including zinc finger nucleases, homing endonucleases, transcription activator-like effector nucleases (TALENs), and RNA-guided nucleases that allow for the targeted modification of cellular genomes. The unifying activity for all of these tools is their nuclease activity, which is the ability to bind a specific sequence anywhere in the genome and introduce a DNA double-strand break (DSB). Once a DSB is generated, repair occurs through one of two basic types of mechanisms: nonhomologous end joining (NHEJ) or homology-directed repair, such as homologous recombination (HR). With enzymatically generated DSBs, NHEJ will typically lead to seamless religation of the break. However, NHEJ may introduce insertions or deletions at the DSB at appreciable frequencies, which can be useful for disrupting gene expression or function, or modifying regulatory functions mediated by the targeted sequences. HR involves the repair of a DSB using a repair template with homology to the sequences flanking the cut site. This template can either be endogenous, such as from a sister chromatid, or may be exogenously introduced. Thus, in addition to simple disruption of the targeted region, HR can be used to introduce complex engineered genetic elements.
When the genome is edited with therapeutic intent, it is an attempt to generate controlled genetic damage and the native repair mechanisms of the cell are relied on to repair the damage. In the safe translation of gene editing to a patient population, it is worth considering that induced genetic damage in the form of chemotherapy and radiation for has been used for decades. The underlying principle of cancer treatment is inducing genetic damage in a fashion that is toxic to cancer cells but does not overwhelm the repair mechanisms of healthy cells. An assessment of chromosomal instability has recently been shown to help predict the survival of a patient receiving chemotherapy and/or radiation. Attempts to concentrate the genetic damage to sites of disease, such as administering intrathecal chemotherapy for central nervous system malignancies or using proton beam therapy to narrow the radiation window are steps toward tissue-level specificity but the impact on treated cells is genome wide. Side effects, both short term and long term, do occur and are well known to the oncologist. Thankfully, although secondary malignancies can occur as a result of chemotherapy and radiation treatment, most patients do not develop treatment-related tumors. The cells either repair their genetic damage or undergo cell death in the presence of excessive damage. By comparison, the risk posed by introducing a transiently expressed, targeted endonuclease that has passed through extensive screening for off-target cutting should be significantly less dangerous. Although the introduction of synthetic genetic elements by HR raises the potential for unintended consequences, the editing process and integration are similarly designed for specificity and would not be predicted to approach the magnitude of risk seen with already accepted treatments for cancer therapy.
Among potential safety concerns of gene editing, the most fundamental is the potential for the nuclease to introduce DSBs at unintended sites in the genome. If the precise targeted sequence or sequence sufficiently similar exists elsewhere in the genome, that site has the potential to be cleaved in addition to the intended site. When designing a targeted endonuclease, it is critical to anticipate potential off-target cleavage sites and select sequences that minimize this potential. This is most commonly carried out initially in silico using tools such as the Predicted Report Of Genome-wide Nuclease Off-target Sites tool for zinc fingers and TALENs (designed and maintained by the Gang Bao Laboratory of Rice University; http://bao.rice.edu/Research/BioinformaticTools/prognos.html ) and the clustered regularly-interspaced short palindromic repeats (CRISPR) Design tool (designed and maintained by the Feng Zhang Laboratory of MIT; ). Unlike basic primer design or sequence alignment tools, these algorithms take into account the nature of the endonuclease platform, such as spacer length for TALENs and Protospacer Adjacent Motif sites for CRISPR, and are better suited for identifying accurate off-target sites within the genome. These tools offer rapid results to aid in the selection and design of potential nucleases. Once an endonuclease has been generated, in vitro screening has been made possible by means of Systematic Evolution of Ligands by eXponential Enrichment (SELEX). SELEX queries the propensity of the endonuclease to cleave a library of various oligonucleotides. The genome can then be probed for the presence of sequences with the highest rates of in vitro cleavage. Matching sequences are an excellent starting point for the search of off-target cleavage postediting in target cells.
Screening for off-target activity is also necessarily performed following the genetic modification of target cells using the intended manufacturing process for cells to be engrafted in patients. Sequencing at in silico predicted off-target cut sites can reveal if mutation has occurred at these specific loci. Mutation events at sites not included in the in silico prediction, as well as nonmutational on-target cleavage repairs, will be missed by this technique. Genome-wide off-target editing analysis has been analyzed either by detection of repair template integration or chromatin immunoprecipitation followed by high-throughput sequencing (ChIP-seq) analysis. Repair templates delivered by nonintegrating viruses should only be detectable at sites of endonuclease activity, therefore primers specific to the repair template but not present in the genome at large can be used to map sites of nuclease activity. ChIP-seq can be used to demonstrate sites of nuclease binding to the genome but does not necessarily indicate cleavage events have occurred. Although both methods offer insight into potential off-target cleavage sites, neither offers comprehensive coverage of the genome or quantification of the likelihood of such events occurring. A hybrid approach called Genome-wide, Unbiased Identification of DSBs Enabled by sequencing has also been described that uses blunt ended double-stranded oligodeoxynucleotide (dsODN) integration at cut sites, followed by next-generation sequencing. DNA DSBs, either on-target or off-target, also have the potential to result in translocation events that can be difficult to detect. A high-throughput genome-wide translocation sequencing (HTGTS) technique has been described that aims to both predict and monitor for these events at specific on-target or off-target sites.
Care should also be taken to consider the anticipated and unanticipated biological impact of the intended gene modification when designing a targeted endonuclease. Unintended impacts can range from decreased cell survival and function to clonal proliferation and oncologic transformation. Gene therapies have been proposed that target intronic sequence, repressor as well as promoter and enhancer elements, transcription factors, and the insertion of synthetic constructs, to name a few. Therapies that rely on the targeted disruption of a transcription factor must consider not just the impact on the gene causing the undesirable phenotype but genes in other pathways as well. The introduction of synthetic gene elements by HR requires further scrutiny to monitor for correct insertion and the potential impact on nearby genes both at the intended and off-target integration sites. One approach to mitigate the potential risk of oncologic transformation is the introduction of a suicide gene that can be activated to eradicate the offending population if needed.
Safety considerations should also extend beyond the direct safety of the patient and include laboratory staff, clinical staff, and direct contacts of the patient. Laboratory staff safety starts with the proper handling of cells and reagents based on appropriate biosafety level assignment to the reagents being used. Transfection of messenger RNA (mRNA) or DNA constructs by electroporation poses little risk to the laboratory staff member. The use of viral elements such as adeno-associated virus (AAV), Integrase-Deficient Lentivirus, or others increases the required safety precautions. Both the nature of the virus and the payload being delivered should be evaluated for the potential to cause harm if laboratory staff members are exposed. Viral carriers that introduce HR templates alone are arguably less dangerous than those containing nuclease elements. Viruses with the potential to integrate into the genome or infect epithelial cells should be handled with greater care. The risk to clinical staff, including nurses and doctors, should be relatively minimal in most cases. Certainly, if the gene therapy takes place in the laboratory (eg, editing hematopoietic stem and progenitor cells), the cells that are infused into the patient are isolated from the clinical staff and pose little risk if an exposure should occur. The risk posed by gene therapy involving the intravenous or direct tissue inoculation with an engineered virus will need to be considered for each protocol. Aerosolization or unintentional needle sticks of infectious elements have the potential to harm clinical staff and a plan to respond to such exposures will need to be part of any gene therapy protocol. Family and close contacts of the patient will need to be aware of any potential shedding of engineered virus by the patient. Fortunately, in almost all cases, the risk will be minimal.
An important topic of debate concerning therapeutic gene editing is the potential modification of germline cells. For families in which one or more members carry a pathogenic genetic trait, ridding their lineage of that trait, even if they would not be able to change their own clinical outcome, is a reasonable aspiration. At the current level of understanding of gene editing, there are both known and unknown risks that render attempts at germline gene editing ethically questionable even for the elimination of traits with unequivocal clinical benefit. First and foremost, genetic tools are not currently understand well enough to know that they can be wielded for a beneficial outcome without unknowingly causing damage. Off-target cleavage events are known potential sequelae of gene editing and, even with current sequencing technology, it is not possible to know that an attempt at beneficial editing has not also resulted in accompanying detrimental genetic alterations. Further issues would arise from any attempt at nontherapeutic genetic enhancement in which the clinical benefit of modifying a genetic trait is less clear. Although modifying a trait or combination of genetic traits in a patient may achieve a desirable phenotypic outcome, it is not known with certainty that the impact will be the same in the genetic milieu of their offspring. With these uncertainties, although a patient can consent to undertake the risk of modifying their own genome, it is not yet clear that those decisions can or should be propagated to their progeny through germline editing. Based in part on these issues, the National Institutes of Health guidelines currently prohibit the use of federal funds for research that aims to alter the germline in either mature adults or human embryos, although it is worth noting that a recent report from the National Academy of Sciences seems leave the door open for such a possibility in the future. Due to the technical and ethical issues associated with germline modification, current practice is that any potential modification of germline cells must be carefully considered and arguably avoided.
Medical monitoring in both the short and long term following a gene therapy procedure must also be carefully planned in advance. The concept of the medical home for gene therapy patients has not yet been well established. It is unlikely that there will be a gene therapy division in most hospital settings but care will instead be carried out by the specialty service caring for the underlying diagnosis. A patient with SCID (severe combined immuno-deficiency) will likely be treated by an immunology department, a sickle cell patient by hematology, and so on. The challenge is that if the curative treatment hoped for is achieved, the patient may be lost to follow-up as their symptoms resolve. This is a particularly important issue in the near future because, due to relative inexperience with gene therapy and gene editing, it is advisable (and currently a US Food and Drug Administration requirement) to monitor gene therapy patients over long periods of time for the development of therapy-related complications. Ensuring that detailed information on the exact genetic manipulation the patient undergoes follows them later in life in some form of medical record will be essential so that any adverse events could be related to general or specific aspects of gene therapy or gene editing. If the potential for germline modification exists, multigenerational access to these data may also be advisable but adds a high degree of medicolegal complexity.
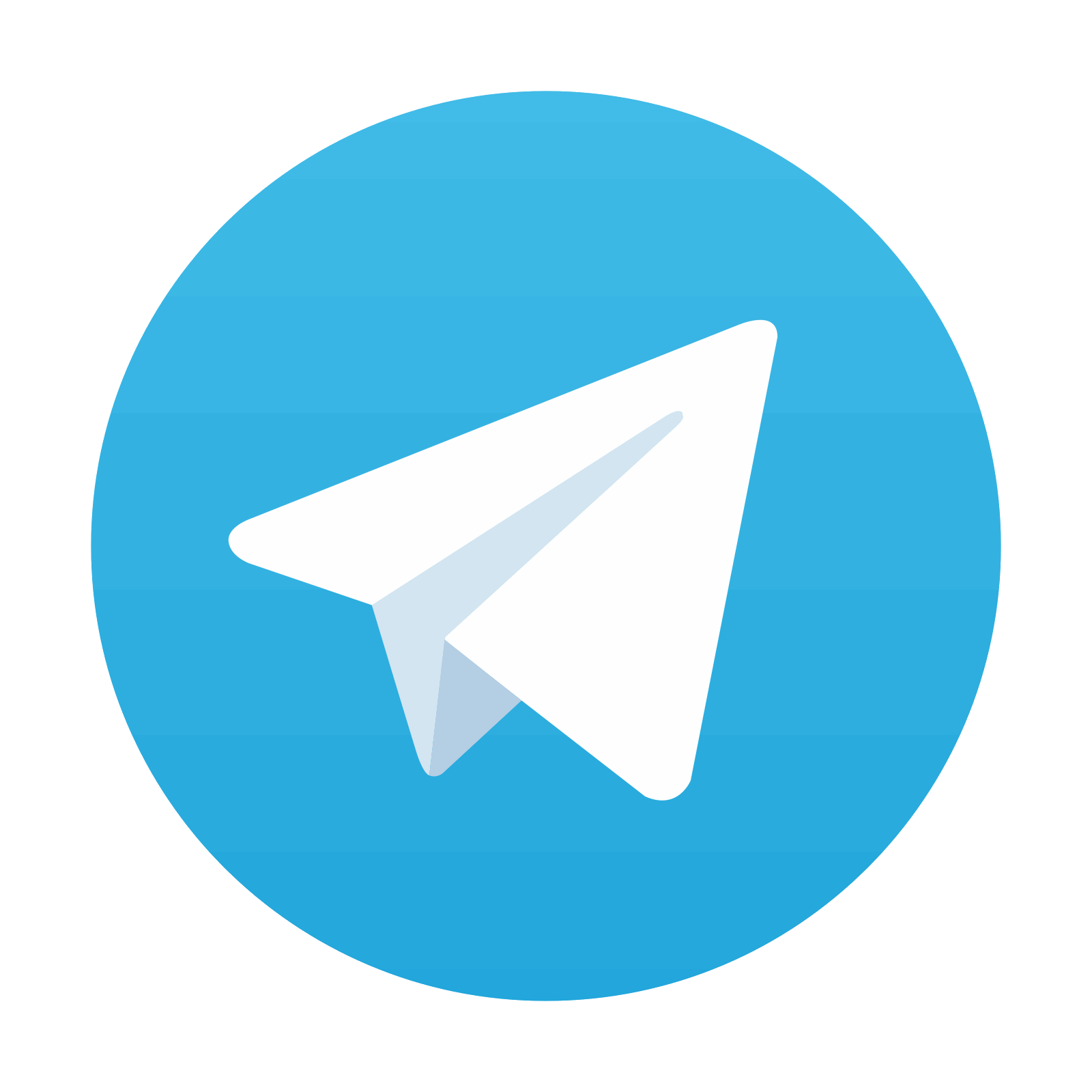
Stay updated, free articles. Join our Telegram channel

Full access? Get Clinical Tree
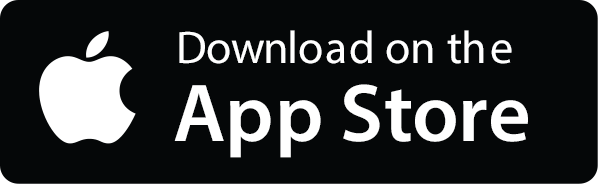
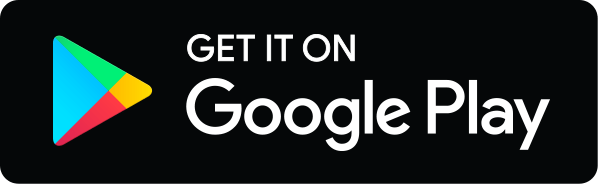