Fig. 18.1
Schematic representation of targets of telomerase and telomere structure for various anticancer strategies
Table 18.1
Telomere- and telomerase-associated proteins and their inhibitors
Target | Approach | Mechanism and consequence | Examples |
---|---|---|---|
hTERT | RNAi-based TERT knockdown | TERT mRNA degradation and gene silencing | Small-interfering RNAs (siRNA) |
Oligonucleotide antagonist (biochemical active site inhibitors) | TERT enzymatic inhibition | AZT (3′-azido-2′,3′-dideoxythymidine), BIBR1532 | |
Telomerase immunotherapy | Activate immune system to kill telomerase-positive tumor cells that express TERT | CD8 +-induced TERT antigen-expressed cancer cell lysis | |
hTERC | Antisense oligonucleotides | hTR degradation and telomerase transcriptase template dysfunction | 2′,5′-oligoadenylate (2–5A) antisense system |
Hammerhead ribozymes | |||
RNAi technique | |||
Telomerase-associated proteins | Interfere telomerase-associated proteins and relative signal pathways | Influence telomerase activity and/or TERT expression | Tankyrase I MAP signal pathway |
TRF1 | Telomeric repeat-binding factor 1 | Small-interfering RNAs (siRNA), natural killer cells | |
TRF2 | Telomeric repeat-binding factor 2 | Small-interfering RNAs (siRNA), natural killer cells | |
TIN2 | TRF1-interacting protein 2, negative regulator of telomere length | Small-interfering RNAs (siRNA), natural killer cells | |
POT1 | Telomere end-binding protein 1 | Telomestatin, BRAC019 RHPS4 |
18.2.1 Strategies Targeting hTERT Inhibition
The hTERT is the key catalytic component that regulates the activity of telomerase. Its expression is tightly controlled which involves a complex transcription network. It cannot usually be detected in normal somatic cells, while it overexpresses in most cancer cells, and its expression level correlates very well with the activity of telomerase. Thus, telomerase inhibition targeted at hTERT has been considered as an ideal direction for cancer treatment.
18.2.1.1 Immunotherapeutic Approaches
Anticancer immunotherapeutic approaches involve the use of peptides derived from hTERT (Chen et al. 2009). The overall concept of this approach is to target telomerase-positive tumors with hTERT-specific T lymphocytes. As telomerase-expressing cancer cells may present epitopes of hTERT through human leukocyte antigen, these cells can be eliminated by using a vaccine, that consists of antigen-presenting cells that were either exposed to high levels of an immunogenic hTERT peptide or modified to overexpress an immunogenic fragment of hTERT to stimulate the patient’s own immune system to attack tumor cells if they display hTERT peptides at their surface through major histocompatibility complex (MHC) presentation.
Vaccines specific for both classes of human leukocyte antigen have been developed, and at least 25 peptides are known to induce hTERT-specific immune responses (Liu et al. 2010). For example, I540 (ILAKFLHWL) and Vx-001 (9-mer cryptic TERT572 peptide) were developed as tumor-associated antigens of hTERT to induce cytotoxic T lymphocyte responses via human leukocyte antigen-A (Roh et al. 2013). Several hTERT-based vaccines have been developed but the most advanced vaccines are the GV1001 (GemVex & KAEL) and GRN-VAC1 (Geron vaccine). GV1001 is a 16-mer peptide from the active site of human TERT (aa 611–626) (Bernhardt et al. 2006; Brunsvig et al. 2006). Vaccination with autologous dendritic cells transfected with hTERT mRNA also triggers CD4+ and CD8+ T cell responses in mice and humans (Nair et al. 2000; Su et al. 2002, 2005).
18.2.1.2 Synthetic Nucleic Acids
The principle of the mechanism is based on the specificity of the synthetic nucleic acids to bind to the mRNA of hTERT and then degrade it. It includes two strategies which are antisense oligodeoxynucleotides (AS-ODNs) and small-interfering RNAs (siRNA). Single-stranded AS-ODNs function by interfering with the translation of hTERT which can lead to degradation of hTERT mRNA via RNase H-mediated cleavage (Crooke 1999).
The antisense oligonucleotides (AS-ODNs) approach for targeting telomerase was first described using AS-ODNs to block the translation of mRNA with a sequence complementary to sense RNA (Phatak and Burger 2007; Cunningham et al. 2006). AS-ODNs is used to target the catalytic component of telomerase (hTERT) and are composed of short single-stranded DNA (ss-DNA) sequences that inhibit telomerase activity by complementary binding to the RNA template. AS-ODNs have been studied intensively and their structure has been modified and significantly improved over the past decade. One of the advantages of this approach is that AS-ODNs do not promote multidrug resistance mechanisms (Harley 2008; Roth et al. 2010). Currently the most successful AS-ODNs is GRN163L. Treatment of human bladder cancer cells through AS-ODNs targeted to hTERT in vitro leads to inhibition of the proliferation of these cells (Kraemer et al. 2007).
siRNA is based on the ability of short double-stranded RNA molecules to form the RNA-induced silencing complex (RISC) which can then hybridize with specific mRNA and cleave it, thereby silencing expression (Agrawal et al. 2003). This technique is especially effective for short-term hTERT knockdown because the dsRNA is degraded in the cells (Lai et al. 2007a). RNAi of hTERT has also been successful with the use of plasmid constructs that exogenously express short hairpin RNA sequences to the hTERT transcript. This technique serves as an alternative approach to gene therapy using viral vectors and allows long-term and permanent gene knockdown (Lai et al. 2007b). The use of retroviral vectors that express short hairpin RNA specific to a segment of the hTERT transcript is also effective for long-term knockdown of hTERT. These RNAi-based techniques can provide effective knockdown of hTERT and involves incorporation of the anti-telomerase sequence into the host genome (Chen et al. 2009).
18.2.1.3 Reverse Transcriptase Inhibitors
Because the hTERT protein is a reverse transcriptase, several well-known reverse transcriptase inhibitors (RTIs) have been tested as telomerase inhibitors. Several studies have reported the effects of azidothymidine triphosphate (AZT-TP), arabinofura-nyl-guanosine triphosphate (Ara-GTP), dideoxyinosine triphosphate (ddITP), ddTTP, and ddGTP on telomerase activity (Strahl and Blackburn 1994, 1996).
18.2.1.4 Gene Therapy-Based Approaches
Another approach using hTERT as a cancer-specific target that has been pursued is gene-directed enzyme prodrug therapy (GDEPT). In cancer cells, hTERT promoter activity is 300-fold higher compared to normal cells. One approach that has been reported involves using the hTERT promoter to drive the expression of a proapoptotic “suicide” gene (such as tumor necrosis factor-related apoptosis-inducing ligand (TRAIL) (Katz et al. 2003) or caspase 6 (Komata et al. 2001). The hTERT-driven suicide gene is then introduced to a tumor where expression of the suicide gene in telomerase-positive cells induces apoptosis. In an alternate version of this telomerase-driven gene therapy, tumors are provided with a hTERT-driven prodrug activating enzyme (Plumb et al. 2001; Majumdar et al. 2001; Liu et al. 2002; Zhou et al. 2007), which results in apoptosis of telomerase-positive cells when administered in conjunction with a prodrug that is metabolized into a toxin. A second strategy which utilizes the specificity of the hTERT promoter directs the replication of a lytic adenovirus in telomerase-positive cells (Kyo et al. 2008). Preclinical studies showed selective targeting and effective cytolysis of telomerase-positive cells derived from a variety of human cancer cell lines, while normal telomerase-negative cells showed reduced viral replication and cytotoxicity. In addition, an adenovirus expressing a ribozyme directed against the T-motif of hTERT significantly reduced telomerase activity, and this was accompanied by a massive cell loss in ovarian cancer cell lines, but not in telomerase-negative human fibroblasts (Saretzki et al. 2001).
18.2.1.5 Inhibition of Signaling Pathways
Signaling pathways such as those carried out by MAP kinase can result in stimulation of the hTERT gene. For example, Ets and AP-1 may play a role in MAP kinase signaling to the hTERT gene, and inhibition of this pathway could be a novel approach to reducing hTERT expression and telomerase activity (Xu et al. 2007). Our lab has also reported hTERT inhibition induced apoptosis in RCC cells (A498 and ACHN) by inhibiting MAP kinase signaling (Prasad and Pal 2014).
18.2.2 Strategies Targeting hTERC Inhibition
The RNA component of telomerase has also been a popular and effective mode of inhibiting telomerase activity to produce cytotoxicity of cancer cells. There are various approaches to target the RNA component of telomerase, hTERC.
18.2.2.1 Antisense Oligonucleotide Approach
As in the case for hTERT transcript knockdown, antisense oligonucleotides against the hTERC template can also be employed to reduce or eliminate telomerase activity. In this approach, a 2′,5′-oligoadenylate (2–5A) antisense system can be used as a mediator of interferon actions via RNase L activation. The result of this approach is that single-stranded templates, such as the TR component, are specifically cleaved. The anticancer utility of this approach has been proven not only in vitro but also in vivo (Kondo and Kondo 2007).
Another strategy is to use oligonucleotides that are complementary to the RNA component of telomerase. To help elongate telomeres, this RNA component must be accessible for binding by telomere ends. It is logical that it should also be accessible for binding by synthetic oligonucleotides and that such binding should block interactions with telomeres and inhibit telomerase function. The use of antisense oligonucleotides (ODNs) for targeting hTERC represents the first telomerase inhibitors. ODNs are short single-stranded DNA sequences that are complementary to a target RNA such as hTERC. ODNs tagged with 20–50-oligoadenylate (2–5A) bind and cleave hTERC that caused apoptosis in glioma, prostate, cervical, bladder, and ovarian cancer cells (Kondo et al. 1998; Kushner et al. 2000; Koga et al. 2001; Yatabe et al. 2002). Two oligonucleotides are approved drugs and other promising candidates are advancing through clinical trials (Corey 2007), suggesting that nucleic acids are a feasible option for clinical development of anti-telomerase agents.
18.2.2.2 Hammerhead Ribozymes and RNAi Approach
Hammerhead ribozymes and RNAi can be used to inhibit the RNA of this ribonucleoprotein, telomerase (Li et al. 2007). Both of these methods lead to degradation of the RNA component of telomerase, hTERC. The effect is rapid cell growth inhibition both in vitro and in vivo independent of telomere length of the target cancer cells. Several studies have shown hammerhead ribozyme to target the template region of hTERC resulting in cleavage of hTERC in vitro and telomerase inhibition in various cancers, i.e., hepatocellular carcinoma and breast cancer (Kanazawa et al. 1996; Yeo et al. 2005).
RNAi-mediated knockdown of hTERC expression has been shown in various cancers cell types (Goldkorn and Blackburn 2006; Zheng et al. 2006). 2–5A (5′-phoshorylated 2′–5′-linked oligoadenylate)-linked antisense is a promising approach to degrade the hTERC transcript by activation of RNAseL. A 2–5A antisense construct suppressed hTERC expression resulting in rapid inhibition of cell viability. The advantage of this technique is that it greatly reduces the lag period that is often encountered in approaches that are dependent upon the shortening of telomeres to result in cancer cell growth inhibition. Thus, methods to inhibit the RNA component of telomerase by using antisense oligonucleotides, hammerhead ribozymes, or RNAi also show great promise in anti-telomerase approaches to cancer therapy.
It has been demonstrated that single-stranded peptide nucleic acid (PNA) oligomers that are complementary to the template regions of the telomerase RNA subunit could inhibit telomerase activity in cancer cell extracts. Rather than function like a standard antisense oligomer to degrade RNA or block translation, the oligomer acts like an enzyme inhibitor to block the telomerase active site. Both PNAs, 2′-O-methyl, 2′-methoxyethyl, and locked nucleic acid (LNA) oligomers could be introduced into cells and inhibit intracellular telomerase (Corey 2009).
18.2.2.3 Gene-Directed Enzyme Prodrug Therapy
Another approach to using hTERC as a cancer-specific target that has been pursued is gene-directed enzyme prodrug therapy (GDEPT). Keith and co-workers (2002) have exploited the transcriptional regulatory sequences of the hTERC gene to regulate expression of the bacterial nitroreductase enzyme in combination with the prodrug CB1954 in a suicide gene therapy strategy. In cancer cells, hTERC promoter activity is 300-fold higher compared to that of in normal cells; thus, by placing the nitroreductase gene under the control of the telomerase gene promoter, sensitization to the prodrug (CB1954 is a potent alkylating agent when released) the cell death was observed. The latter was restricted to cancer cells exhibiting high levels of promoter activity and this effect was retained in xenograft models in vivo (Plumb et al. 2001). The hTERC GDEPT concept is promising and currently under advanced preclinical investigation.
18.3 Potential Problems with Targeting Telomerase
As with most biological pathways, telomere maintenance may be much more complex than previously thought. In fact, telomere maintenance may not be simply a result of the activation of telomerase activity. It does appear that all immortalized cell lines have a telomere maintenance mechanism, but that mechanism can be either telomerase or an alternative mechanism called “alternative lengthening of telomeres” (ALT) (Niida et al. 2000). There is a growing list of immortalized lines that have no detectable telomerase activity. Notwithstanding the absence of telomerase activity, these human cell lines maintain very long telomeres. This raises a crucial question for the future use of telomerase inhibitors as anticancer agents. If telomerase is inhibited in cancer cells that are maintaining their telomeres by telomerase, will these cells be able to activate the ALT pathway and therefore become resistant to the anti-telomerase agents? This question has yet to be answered in vivo, but it raises the possibility that resistance mechanisms may be available and selected for in cancer cells treated with telomerase inhibitors.
18.4 Strategies to Target Telomeres
Experimental evidences have shown that directly targeting telomere structure by telomere uncapping will lead to rapid cell death. DNA damage signals at the G-strand overhang and the corresponding lagging strand could lead to uncapping of the chromosome and rapidly occurring apoptosis or genomic instability. Here, we discuss various approaches which target telomere structure.
18.4.1 Targeting T-Loops
One strategy for targeting the telomere maintenance mechanisms is to take advantage of the distinctive DNA structures that have been shown to form with telomeric DNA sequences. The unique structure and dynamics of T-loops found at the ends of telomeres provide a number of opportunities to selectively target telomere maintenance mechanisms. The T-loop consists of a duplex 6-base pair repeat (TTAGGG)n with a G-rich single-stranded end that invades and closes into the duplex region to form an as yet undetermined secondary DNA structure (Griffith et al. 1999). In addition to the nucleic acid component, a number of proteins, including TRF1, TRF2, and probably telomerase, are associated with the T-loop structure. Telomere extension by telomerase involves both structural and dynamic changes in the T-loop that go beyond the telomerase cycle of elongation and translocation. The T-loop and postulated D-loop duplex and single-strand invasion junction are ideal targets for DNA- interactive agents (Bearss et al. 2000).
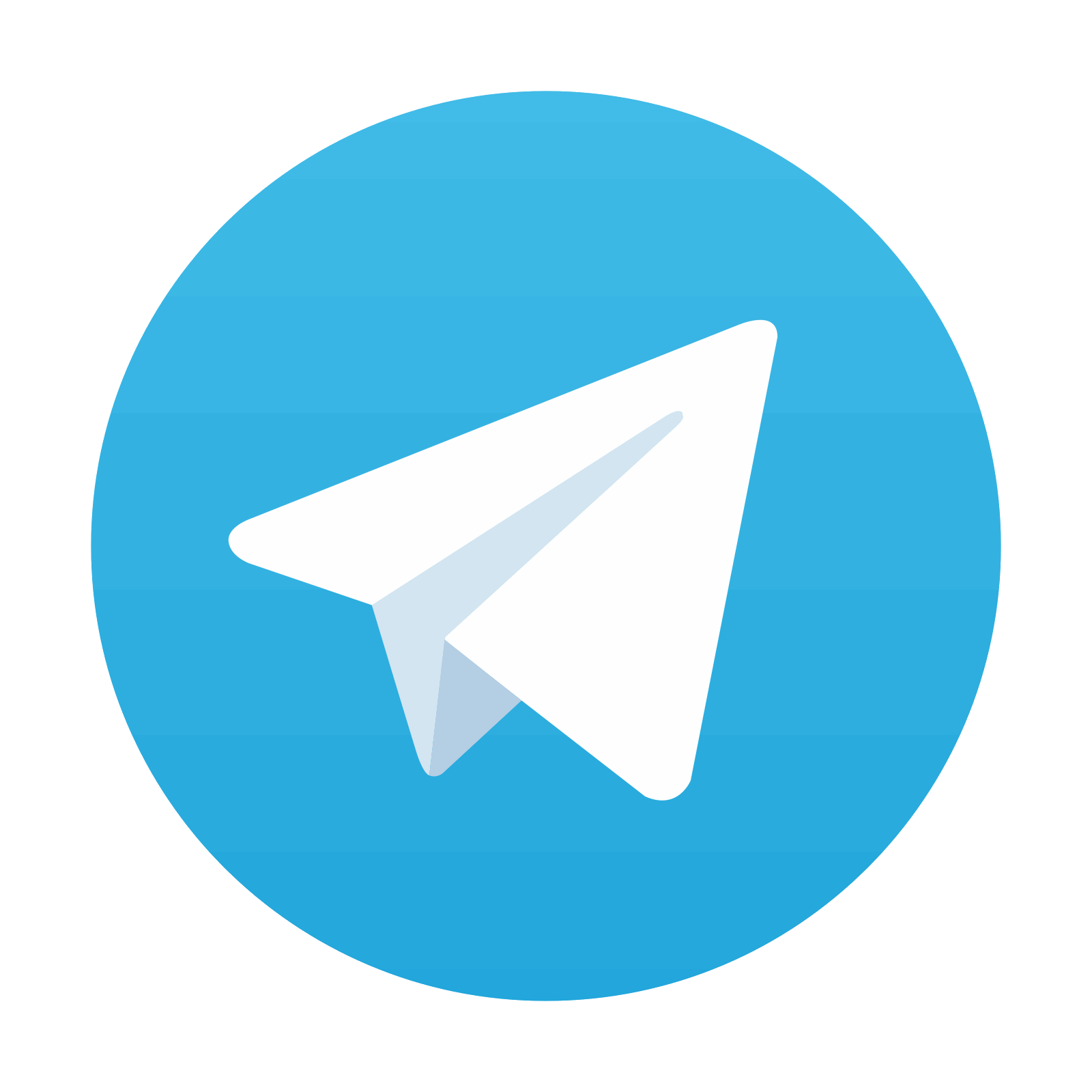
Stay updated, free articles. Join our Telegram channel

Full access? Get Clinical Tree
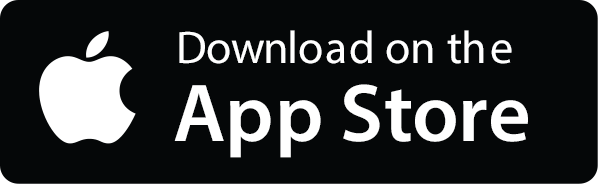
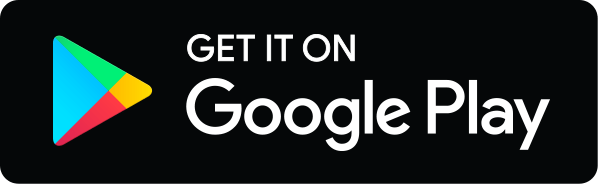