The observed increment in a recipient of one unit of blood was 5000/mm3, which indicated that a small change in the circulating platelet count is sufficient to arrest bleeding temporarily, usually for less than 24 hours. The next decade was spent in determining how to best separate and store platelets derived from whole blood (see below).
Allan Kliman developed an ingenious technique based on the concept of plasmapheresis to increase the number of platelets available for transfusion from a single volunteer donor, a procedure that came to be known as manual plateletpheresis (Kliman et al. 1961; see also Chapter 17). Subsequent studies used the defined ‘safe’ volume and frequency of donation: up to 1000 ml of plasma weekly without a significant decline in the patient’s circulating protein or blood platelet count.
Preparation of Platelet Concentrates from Single Units of Whole Blood
The first platelet concentrates derived from whole blood were prepared as platelet-rich plasma, a component containing relatively few platelets (3.0 × 1011/l) in an impractically large volume of plasma (>200 ml) (Freireich et al. 1963). Efforts to reduce volume by high-speed centrifugation resulted in a pellet of irreversibly clumped cells, and the alternative use of the low-volume anticoagulant, ethylenediamenetetraacetate (EDTA) caused the transfused platelets to undergo rapid hepatic sequestration (Aster and Jandl 1964). Technical modifications that included acidification of the suspension medium and room temperature preparation eventually led to the current methods of preparing platelet concentrates (Mourad 1968).
Meticulous attention to numerous small steps in the preparation and storage of platelet concentrates from whole blood is necessary to ensure the maximum number of viable and functional platelets. Early empirical studies defined in detail the anticoagulant, storage container plastic, initial ‘soft spin’ (1000 g for 9 min), subsequent ‘hard spin’ (3000 g for 20 min), resuspension technique, storage volume and storage conditions to achieve platelets with 46% in vivo recovery and 7.9-day survival following 72 h of storage (81% of fresh platelet viability) (Slichter and Harker 1976a,b,c). In addition, the temperature at which the blood is maintained before and during processing, the interval between collection and preparation of the platelet concentrate, the white cell and red cell contamination, and the temperature and mode of agitation during storage are all important considerations (Slichter 1985).
Two methods for preparing platelet concentrates are described in Appendix 1; one method involves an initial gentle centrifugation to obtain platelet-rich plasma (PRP) and concentration of platelets by further centrifugation and the second method involves a more vigorous initial centrifugation to obtain buffy coats from whole blood and the subsequent recovery of the platelets by gentle centrifugation. Single or previously pooled, resuspended buffy coats (4–7 units) can be stored for up to 5 days to provide an adult platelet dose of 3 × 1011 platelets in approximately 300 ml of plasma (Pietersz et al. 1985; Wildt-Eggen et al. 1996). Two types of instruments, the Optipress or Biopack, using two outlets in the primary collection pack (‘bottom and top’), and the Compomat or Ex30, using traditional multiple packs, have been developed to automate buffy coat platelet collection and plasma separation. Platelet concentrates prepared under these conditions have 0.09 ± 0.04 leucocytes × 108/unit. The full adult dose of approximately 3 × 1011 platelets in 300 ml of plasma prepared with Optipress contains the same number of leucocytes. Platelet concentrates from PRP should contain at least 5.5 × 1010 platelets in 50 ml of plasma or an appropriate platelet suspension medium. Storage of pooled whole blood-derived platelet concentrates is now accepted and both in vitro and in vivo studies suggest that component quality is maintained for at least 7 days (Slichter et al. 2012; Larsson et al. 2005).
The principles of platelet storage (see below) are probably identical regardless of the method of preparation. The pH should not be allowed to fall below 6.4 or rise above 7.4 throughout the shelf-life of any platelet concentrate. Leucocyte-reduced buffy coat platelet pools can be prepared either by filtration or by centrifugation (Wildt-Eggen et al. 2001). Leucodepletion filters especially designed for platelet concentrates remove more than 90% of leucocytes from pooled platelets or platelet concentrates collected with cell separators (Chapter 17) with a loss of less than 15% of platelets.
The Survival of Platelets in Vivo
51Cr and 111In as Labels
The introduction of 51Cr as a label for platelets (Aas and Gardner 1958) provided an objective means of defining the kinetics of platelet transfusion. Because uptake of 51Cr by platelets is poor, 51Cr has now been replaced by 111In (T1/2 2.8 days) or 113In (T1/2 100 min), complexed with a suitable chelating agent as the label of choice for platelet studies. Although oxine (8-hydroxyquinoline) was used originally (Thakur et al. 1976), tropolone proved a more convenient chelator because indium tropolonate, unlike indium oxinate, labels cells effectively in the presence of plasma. Results using the two methods do not differ significantly (Kotze et al. 1991). Optimal labelling with 111In is achieved using a tropolone concentration of 2 × 10−4 mol/l and a plasma concentration of 50% (Danpure et al. 1990). Under these conditions, autologous platelets can be used in patients with platelet counts as low as 4 × 109/l. Conditions may need to be altered when labelling platelets stored in plasma-free synthetic media (see below). Compared with 51Cr, 111In is less radiotoxic and far more suitable for quantitative scintillation camera imaging.
Storage of platelets does not appear to affect the uptake of 51Cr or 111In. Therefore radiolabelled platelet recovery and survival studies can be used to define optimal conditions for storage. The lifespan of two populations of platelets in the same subject can be compared by using 51Cr for one population and 111In for the other (Keegan et al. 1992). However, the T1/2 and mean lifespan of stored platelets are significantly longer with 51Cr than with 111In labelling, indicating that estimates of viability of stored platelets may be influenced by the choice of label (Wadenvik and Kutti 1991) or even by the use of different methods using the same the label (Rock and Tittley 1990). Other factors that obscure comparisons between labelling studies are differences in sampling and curve fitting, which substantially affect estimates of mean platelet lifespan (Wadenvik and Kutti 1991). Comparisons of results from different laboratories are valid only when identical methods have been used. Detailed methods for conducting survival studies with radiolabelled platelets have been published (Snyder et al. 1986).
Use of Biotin
Platelets labelled with biotin can be detected in a blood sample by adding streptavidin–phycoerythrin prior to flow cytometry. By labelling platelets in two populations at different strengths, the lifespan of the two populations can be compared (Franco et al. 1994).
Normal Survival of Platelets in Vivo: Venting the Spleen and Other Issues
Although in vivo characterization of platelet recovery and survival was derived initially from measurements made with 51Cr, most studies in the last 20 years have been made with 111In and γ-scintillation camera computer systems that are capable of dynamic imaging.
Initial Survival (‘Recovery’)
If fresh platelets from a normal subject are labelled with 111In, the initial rapid fall in the plasma concentration of platelets, which is also seen with 51Cr-labelled platelets (Kotilainen 1969), is complete in 20 min; at 10 min the value is about 5% above the 20-min value (Peters et al. 1980). The term recovery is used for the number remaining in the circulation after this phase of rapid disappearance, and is expressed as a percentage of the number expected if all the transfused platelets were in the circulation.
Platelet recovery has been determined as follows: with 111In, 71% (Heaton et al. 1979) and 72% (Heyns et al. 1980); with 51Cr, 66% (Aster and Jandl 1964); 67% (Aster 1965) and 64.6% (Harker and Finch 1969). In splenectomized subjects, about 90% of injected platelets are recovered (Harker and Finch 1969; Kotilainen 1969). Conversely, with splenomegaly, low values have been found (7–27% in congestive splenomegaly; Harker and Finch 1969). When the spleen is extremely enlarged, seven times as many platelets may be sequestered as circulate in the blood (Penny et al. 1966).
Although a fraction of total circulating platelets normally pools in the spleen (see below), recovery of labelled platelets from splenectomized subjects rarely exceeds 90%. The rapid disappearance of a proportion of labelled platelets from the bloodstream soon after injection may reflect damage that occurs during the labelling process.
The disappearance curve may be obtained by plotting the number of circulating labelled platelets against time after injection. In normal subjects the number decreases rapidly in the first 10–20 min but falls much more slowly thereafter. The survival time is defined as the time after injection at which survival falls to zero. Estimates of survival time using 111In-labelled platelets are 9 days (Heyns et al. 1980) and 8–10 days (Bautista et al. 1984), findings identical to those established with 51Cr-labelled platelets (Kotilainen 1969; Abrahamsen 1970). Platelet survival values obtained with 111In fit linear kinetics (Heyns et al. 1980; Bautista et al. 1984). Several mathematical models of data analysis have been investigated; however, the multiple-hit method and the weighted mean technique yield similar results (Lotter et al. 1986).
In normal subjects, two mechanisms of platelet removal have been identified: (1) a fixed number of platelets is consumed for daily vascular maintenance and (2) platelet ageing determines the remaining lifespan (Hanson and Slichter 1985). Platelet survival correlates directly with platelet count in the thrombocytopenic patients. Platelet lifespan is modestly reduced in patients with counts in the range of 50 000–100 000/μl, but markedly reduced when the count falls below 50 000/μl. The recovery of autologous platelets is normal when the platelet count exceeds 50 000/μl, but reduced in patients with lower counts because the number of platelets required for vascular maintenance becomes an increasing percentage of the total count. All patient and normal data correlate well with a model that predicts a fixed requirement for 7100 platelets per microlitre of blood per day, or about 18% of the normal rate of platelet turnover (41 200 platelets per microlitre per day on average) (Hanson and Slichter 1985). If the population of newly formed platelets is labelled with 51Cr and followed after an episode of rapid platelet destruction, a plateau type of disappearance curve is observed with 50% survival at 7 days, followed by almost complete disappearance of the labelled population by 9 days, thus demonstrating the relationship between platelet ageing and survival (Harker 1977). In addition to the physiological requirement for platelets, thrombocytopenic patients who are febrile, infected and undergoing cytoreductive therapy require additional platelets to maintain haemostasis (Harker and Slichter 1972a).
Platelets can be separated by centrifugation into ‘heavy’ cells, with a mean survival time of about 314 h, and ‘light’ cells, with a mean survival time of 75 h. The total population lifespan is about 190 h (Corash 1978). Cohort experiments confirm that the heavy platelets are the younger ones. In the same study, platelets from platelet-rich plasma had a survival time of 155 h, presumably because a percentage of young platelets were spun down with the red cells and leucocytes and excluded from the platelet-rich plasma. Examples of platelet survival curves obtained with 111In are presented in Figure 14.1.
Figure 14.1 Survival curves of autologous platelets labelled with 111In in seven normal subjects. The values at equilibrium after injection were defined as 100% and correspond to a mean value of 72% (SD 16%) of that expected if all the labelled platelets had been in the circulation. The slopes are best fitted by a linear function and mean survival is 9.0 days (SD 0.71 days). Elution of label from the platelets is negligible.
(Source: Adapted from Heyns et al. 1980. Reproduced with permission of John Wiley & Sons Ltd.)
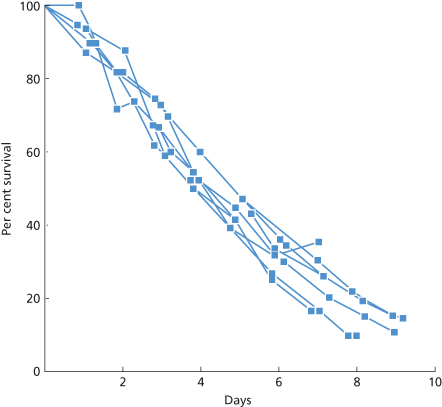
51Cr labelling studies indicate that about one-third of the total circulating platelets are concentrated in the spleen, exchanging freely with platelets circulating in the periphery (Aster 1966; Harker and Finch 1969). Using 111In-labelled platelets, a scintillation camera and computer-assisted imaging, Heyns and co-workers (1980) estimated the splenic pool to be 29.6% of the total circulating platelets. Measurements of intrasplenic platelet transit time arrive at a similar figure (Peters et al. 1984). Some pooling of platelets may occur in the liver (Heyns et al. 1980). The liver and spleen account for the clearance of 72.3% of the total radioactivity, a figure that corresponds closely to values obtained using 51Cr (Aster 1966). Most of the activity not in the liver or spleen remains in the thorax and lower abdomen indicating that these regions contain sites that sequester platelets.
Radiolabelling to Assess Viability of Transfused Platelets
Isotopic studies are used to predict the circulation of stored platelets that are transfused to amegakaryocytic, thrombocytopenic patients. Recovery and viability are reasonable surrogates for the post-transfusion increment that is used by clinicians, although none of these measurements ensures haemostatic efficacy in vivo. Unlike the case of the red cell, no generally accepted standard for survival after 24 h has been set for the radiolabelled platelet (Murphy 2002). As a result, studies that propose extending storage tend to use isotopic studies of platelets stored for currently licensed maximum periods, rather than fresh platelet values, as a control (Hogge et al. 1986; Simon et al. 1987). A recent proposal to use two-thirds survival and 50% recovery of fresh platelets (<24 h post collection) as the ‘gold standard’ has been validated for current 5-day platelet storage (AuBuchon et al. 2004; Murphy 2004).
Relation Between Platelet Count and Bleeding Time
In patients with thrombocytopenia due to underproduction, the bleeding time is prolonged when the platelet count falls below about 100 × 109/l. Below this level, the relation between the bleeding time, as determined by the template method of Mielke (Mielke et al. 1969), and the platelet count is roughly linear to a count of about 20 × 109/l as described by the following equation (Harker and Slichter 1972b):
so that for a platelet count of 10 000/μl
In patients with immune thrombocytopenic purpura (ITP), the bleeding time is shorter than that predicted from the platelet count, whereas in conditions in which platelet function is disturbed, as in uraemia or in von Willebrand disease, bleeding time is prolonged even when the platelet count is normal (Harker and Slichter 1972b).
This relationship can be used to measure in vivo function of stored platelets both at the time of transfusion and several hours later (Figure 14.2).
Figure 14.2 Bleeding times and platelet count in seven aplastic, thrombocytopenic patients following 20 transfusions of unstored platelets. Pre-transfusion bleeding time exceeded 60 min and platelet count was less than 10 × 109/l in all cases. Open circles (s) are measurements between 5 and 150 min post transfusion. Closed circles (d) after 150 min.
(Source: Slichter and Harker 1976. Reproduced with permission of John Wiley & Sons Ltd.)
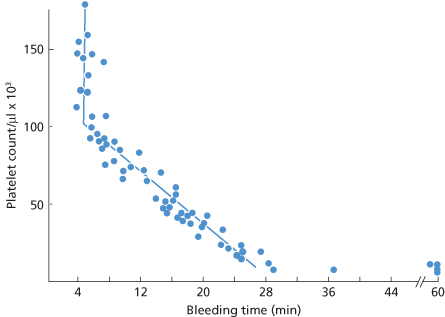
Storage of Platelets in the Liquid State
Preparation of Platelet Concentrates
Platelet-Rich Plasma (PRP) Method
In this method, the PRP is centrifuged at high speed and, after storage at room temperature for 1–1.5 h, the platelets in the pellet are resuspended in about 60 ml of autologous plasma or synthetic medium (see Appendix 1).
The Buffy Coat (BC) Method
With this method, whole blood is centrifuged at high speed, after which first the plasma and then the buffy coat is removed. To the buffy coat, 60 ml of plasma or synthetic medium is added and, by centrifugation at low speed, the platelets are separated from the remaining red cells and the leucocytes. Then, 25 ml or so of red cells from the collected unit are lost during the buffy coat preparation. Pooling of platelets from four donations provides a therapeutic platelet dose (see Appendix 1).
The BC method has several advantages over the PRP method. More plasma becomes available for fractionation; BC-PC contain fewer leucocytes than PRP-PC (Pietersz et al. 1987). Furthermore, buffy coat platelets are less activated than PRP platelets (Fijnheer et al. 1990; Bertolini et al. 1992). Activation of platelets may decrease viability in vivo (see below). Initially, a disadvantage of the BC method was a lower platelet yield (Hirosue et al. 1988), but automated systems for preparing BC have improved the yield (Högman et al. 1989; Pietersz et al. 1989). The yield improves further when the blood from which the BC is prepared is kept at 20°C for 12–24 h before centrifugation (Fijnheer et al. 1990).
Plateletpheresis
This is used to obtain an adult therapeutic dose (or more) of platelets from a single donor. For further details, see Chapter 17.
Platelet Metabolism
In plasma, platelets derive 85% of their energy from oxidative metabolism using as substrates exogenous glucose, free fatty acids, acetate and amino acids (Kilkson et al. 1984; Bertolini et al. 1992; Shimizu and Murphy 1993). Only 15% of energy is derived from anaerobic glycolysis (Kilkson et al. 1984). The carbon dioxide produced in the oxidative pathway may be converted to bicarbonate, the major buffer in plasma. During anaerobic glycolysis, lactate is produced, which, unless neutralized by bicarbonate, causes a rapid fall in pH. Lack of oxygen switches the metabolism to anaerobic glycolysis, whereas addition of acetate to the preservation medium may direct metabolism to oxidative metabolism, thus decreasing lactate formation (Guppy et al. 1990; Bertolini et al. 1992).
Storage Temperature
Originally platelets were stored at 4°C, but at that temperature platelets lose their discoid shape and survive poorly after transfusion. Platelets retain viability well when stored at 20–24°C (Murphy and Gardner 1969, 1971, 1976). Recovery and survival time are similar after storage for 72 h at 22°C in CPD, ACD, CPD-A1 or CPD-A2 plasma (Slichter and Harker 1976c; Scott and Slichter 1980; Holme et al. 1987). After storage at 4°C for 24 and 72 h, platelet viability drops to 18% and 9%, respectively, compared with that of fresh controls (Slichter and Harker 1976c; Murphy and Gardner 1969). Loss of viability is a function of both degree of chilling and length of exposure. Exposure of platelets, even for short periods of time (17 h), to 12°C or 16°C, or even to 18°C for 96 h, significantly reduces in vivo viability (Gottschall et al. 1986; Moroff and George 1994). Although chilled storage is associated with a morphological disc to sphere transformation in the platelet (White and Krivit 1967), shape change does not appear to cause the loss of viability (see below).
After storage of platelets at 22°C for 24 h, glycogen stores become depleted, toxic metabolites accumulate and the cells develop impaired aggregation and adhesion responses. These functions are well preserved at 4°C (Shively et al. 1970; Murphy and Gardner 1971; Rock and Figueredo 1976). The storage abnormalities reverse in vivo after transfusion, although the exact interval required for recovery is not known (Murphy and Gardner 1971; Becker et al. 1973). These observations have led some to suggest that an inventory of ‘activated’ refrigerated platelets be maintained to treat bleeding thrombocytopenic patients, while a separate stock of room temperature platelets with superior survival characteristics is kept for prophylactic transfusion (Valeri 1976), an imaginative if impractical proposal.
Storage Lesion: Is Platelet Activation Partly to Blame?
Glycolysis during storage at 22°C results in an increased lactate production and a fall in pH (Murphy et al. 1970). When pH reaches 6.8, platelet morphology begins to change and shape change becomes striking when pH reaches 6.0 and viability is lost. The rate of change in pH is affected by the platelet concentration and by the availability of oxygen. Therefore the gas permeability of the plastic container for carbon dioxide diffusion and the preservative solution are critical factors. When too few platelets are stored in gas-permeable plastics, excess carbon dioxide may be lost and pH may rise too high. A pH rise above 7.3 is also accompanied by platelet shape change, and at a pH above 7.5 (at 22°C), platelet viability deteriorates (Murphy 1985).
Even at normal pH, platelet function and integrity decline during storage. Activation is considered the main culprit for the storage lesion of 22°C-stored platelets, although the evidence is largely circumstantial. A negative correlation has been found between platelet viability and the expression of activation-dependent antigens such as P-selectin (Keegan et al. 1992), CD62 and lysome-associated membrane proteins 1 and 2 (Divers et al. 1995). A correlation has also been found between the amount of glycoprotein (GPIb and GPIIb/IIIa) on the platelet surface and platelet survival. The amount of these GPs decreases during storage, apparently as a consequence of platelet activation (Fijnheer 1991). Finally, addition to the anticoagulant of inhibitors of platelet activation and an inhibitor of thrombin improves the condition of platelets after 5 days of storage (Bode and Miller 1988, 1989). However, the amount of membrane GPIb and IIb/IIIa does not correlate well with platelet injury (Murphy et al. 1994). Furthermore platelets activated by thrombin ex vivo circulate well in animal models (see below). In any case, the decreased aggregation and adhesion capacity of stored platelets cannot easily be attributed to activation.
Effect of Different Plastics
The permeability of the plastic to oxygen is of great significance for oxidative metabolism and thus for the quality of stored platelets. The following ‘second generation’ plastics have been found to be satisfactory: (1) a blend of polyolefin plastic constituents without a plasticizer (PL-732, Baxter Healthcare) (Murphy et al. 1982); (2) polyvinyl chloride (PVC) plasticized with tri-(2-ethylhexyl) trimellate (CLX Cutter) (Murphy et al. 1982); (3) PVC plasticized with the conventional di-(2-ethylhexyl) phthalate (DEHP) but with a reduced thickness and a larger surface area (Teruflexa, Terumo) (Holme et al. 1989); (4) PVC with the phthalate ester analogue tri-(2-ethylhexyl)-1,2,4 benzene tricarboxylate as plasticizer (F720-BIOtrans) (Koerner 1984); (5) PVC plasticized with butyryl-n-trihexyl citrate (BTHC) (PL-2209, Baxter Healthcare). BTHC has the advantage of being suitable for both platelets and red cells and, unlike DEHP, does not leach into blood components during storage (Gullikson et al. 1991; Högman et al. 1991a). DEHP has been cited as a potential toxin (see Chapter 15); and (6) polyvinyl chloride plasticized with di-n-decyl phthalate (DNDP) (Murphy et al. 1995).
The Effects of Agitation
When platelet concentrates are not agitated during storage, pH falls rapidly (Murphy and Gardner 1976). Agitation facilitates gas exchange, which is usually cited as its mode of action, although diffusion of metabolites and maintenance of platelets in a mobile liquid medium may be an added benefit (Sweeney et al. 1995). The method of agitation makes a difference; a flat-bed agitator was found to be more effective than a rotary agitator (Murphy et al. 1982). Vertical and horizontal agitation appear equally effective (Gunson et al. 1983). Flat-bed shakers with 1.5-inch lateral movement are preferable to shakers with a 1.0-inch lateral movement because, with more rapid acceleration and deceleration, platelet buttons formed after centrifugation are resuspended more efficiently (Snyder et al. 1985). Before agitation is begun, the platelets should remain undisturbed for 1 h. When platelets are stored in polyolefin bags, discontinuation of agitation, even for 24 h, has hardly any effect on pH (Moroff and George 1990; Hunter et al. 2001). Platelets that are shipped, even with land and air transport lasting 12 h, result in adequate viability, post-transfusion increments and apparent in vivo function, equivalent to cells that are kept in storage with agitation (Simon and Sierra 1982, 1989; Haddad et al. 2006).
Figure 14.3 Platelets from 12 HLA-matched donors given to the same patient. Corrected count increment (CCI) at site of collection and storage at a hospital on the east coast of the USA (closed diamonds). CCI after surface and air transport to a hospital on the Gulf Coast, USA (open circles); transport time (without agitation) was 6–11 h.
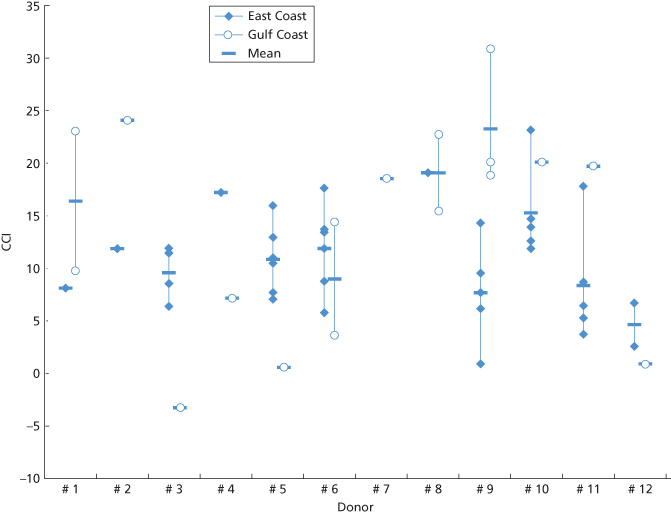
Effect of Contamination with Leucocytes
When platelet concentrates are stored in thick plastic containers, the oxygen supply is limited. Under these circumstances, leucocytes in the concentrate compete with platelets for available oxygen; anaerobic glycolysis and lactate production in the platelets are stimulated, leading to an accelerated fall in pH (Gottschall et al. 1984). When the more oxygen-permeable containers are used, a marked decrease in pH is rarely seen, regardless of the leucocyte content of the concentrate (Moroff and Holme 1991). However, proteolytic enzymes produced by monocytes and released from neutrophils during storage, may remove glycoproteins from the platelet membrane (Sloand and Klein 1990). The loss of glycoproteins may affect platelet viability (see below).
Concern has been expressed that removal of leucocytes before storage might increase the danger of bacterial growth. However, when the buffy coat is maintained for 1 h at 22°C before the platelet concentrate is prepared, most of the bacteria are ingested and removed with the leucocytes, thus considerably reducing the danger of bacterial contamination (Högman et al. 1991b).
Plasma Versus Synthetic Media for Storage of Platelets
Advantages of storage in synthetic media include the removal of plasma proteins such as thrombin, plasmin and C3a that are involved in platelet activation and, depending on the composition of the medium, less accumulation of lactate and a smaller fall in pH. The viability of platelets after storage in a plasma-free electrolyte medium containing glucose, with bicarbonate to buffer lactic acid, was substantially better than after storage in plasma (survival after 7 days’ storage 144.1 ± 15.9 h and 100 ± 32 h respectively) (Holme et al. 1987). Unfortunately, the bicarbonate has to be sterilized and stored at an alkaline pH in a separate container (Holme et al. 1987). Replacement of bicarbonate by phosphate buffer is not effective because phosphate stimulates glycolysis and the formation of lactic acid (Murphy et al. 1991). Excellent results have been obtained with glucose-free crystalloid media such as plasmalyte-A. The essential constituent of these media is acetate, which is oxidized to bicarbonate by platelets (Bertolini et al. 1992; Shimizu and Murphy 1993; Murphy et al. 1995). Metabolism of platelets stored in solutions containing acetate is significantly different from that of platelets stored in plasma. If the oxygen supply is adequate, less glucose is metabolized and less lactic acid is produced and an adequate pH is maintained for 15 days unless the number of stored platelets is exceptionally large (Bertolini et al. 1992). Acetate has been confirmed as a useful fuel for platelet metabolism (Whisson et al. 1993). Pyruvate suppresses lactic acid production to the same extent as does acetate (Murphy et al. 1995). Storage of platelets for 7 days or longer is now possible (Dumont et al. 2002; Sweeney et al. 2004), although measures must be taken to reduce the risk of bacterial growth (see Chapter 16).
Relation Between in Vitro Platelet Assays and in Vivo Recovery and Survival
The response of platelets to hypotonic shock, also called the ‘osmotic reversal reaction’, was first described by Fantl (1968). The results of this test correlate well with viability (Murphy et al. 1991). The test is based on the observation that light transmission through platelet-containing plasma increases abruptly after addition of hypotonic solutions, because the influx of water into the platelets leads to swelling and a dilution of cytoplasmic contents. Healthy platelets regain their initial volume and initial concentration of cytoplasmic colloids. Water and electrolytes are pumped out of the platelets and light transmission returns to normal values.
Morphological changes in platelets stored at 20°C correlate with post-transfusion viability; concentrates with the highest proportion of discoid platelets have the best survival (Kunicki et al. 1975). Similarly, the shape of platelets, or their change in shape and lactate production, correlate with viability (Moroff et al. 1990). The shape change is also associated with a decrease in the amount of ATP and ADP in platelets (de Korte et al. 1990).
The percentage of discoid platelets has been assessed by light-scattering measurements (Fratantoni et al. 1984) and a method has been devised for assessing platelet viability by visual inspection. If a bag containing platelet concentrate is held near a light source and given a twist, an appearance of swirling, caused by light scattering indicates that the platelets have retained their discoid shape. The swirling does not occur when platelets have been stored at 4°C and when the pH has fallen to 6.2. A scoring system to assess the magnitude of the swirling phenomenon has been devised (Fijnheer et al. 1989; George et al. 1989). In a study conducted with 11 laboratories, the reproducibility of swirling as a measure of platelet quality proved promising (Murphy et al. 1994).
Refrigerated Storage of Platelets
Storage Lesion: What’s Activation Got to Do with It?
Refrigerated storage compromises platelet recovery and survival following transfusion. Although platelets stored in the cold have better in vitro function than platelets stored at room temperature (Becker et al. 1973; Snyder et al. 1989b), the markedly shortened survival of chilled platelets offsets any functional advantage. Recent studies suggest that the storage lesion is not one of activation and that chilled platelets are removed in part by a novel hepatic mechanism (Hoffmeister et al. 2001; Rumjantseva et al. 2009).
Biochemical analyses indicate that platelet cytoskeletal alterations and increased responsiveness to agonists are detectable as the temperature falls below 37°C (Hoffmeister et al. 2001). Cooling below 15°C induces extensive platelet shape changes mediated by intracellular cytoskeletal rearrangements. These alterations can be partially reversed by rewarming. Rewarmed concentrates contain more spherical than discoid platelet forms. Conventional teaching aside, platelets activated ex vivo by thrombin, despite extensive shape changes, continue to circulate and function in mice and in non-human primates (Michelson et al. 1996; Berger et al. 1998). Chilled and rewarmed platelets, preserved as discs with pharmacological agents, are cleared with the same speed as are untreated chilled platelets; misshapen chilled and rewarmed platelets circulate just like 22°C platelets in a mouse model (Hoffmeister et al. 2003a). The cold storage lesion appears to be something other than or in addition to shape change.
The quantities of IgG or IgM bound to chilled or room temperature human platelets are identical, implying that binding of platelet-associated antibodies to Fc receptors does not mediate the clearance of cooled platelets. Chilling of platelets does not induce detectable phosphatidyl serine on the platelet surface in vitro, so that phosphatidyl serine exposure and the involvement of scavenger receptors in the clearance of chilled platelets seem unlikely. Complement type 3 (CR3) receptors on hepatic macrophages are responsible for the recognition and clearance of chilled platelets. Chilled platelets develop clustered von Willebrand (vWF) surface receptors (GP1b), enhancing recognition of mouse and human platelets by hepatic macrophage CR3 receptors. The extracellular domain of the GPIbα molecule, isolated by proteolysis from intact platelets, binds avidly to CR3 in vitro, and when immobilized on a surface, supports the rolling and firm adhesion of THP-1 cells (Simon et al. 2000). Cleavage of the extracellular domain of murine GPIbα results in normal survival of chilled platelets transfused into mice. GPIbα depletion of human chilled platelets greatly reduces ingestion of the treated platelets by macrophage-like cells in vitro. The normal clearance of chilled platelets lacking the N-terminal portion of GPIbα rules out the many other CR3 binding partners, including molecules expressed on platelet surfaces, as candidates for mediating chilled platelet clearance. Chilled platelets bind vWf and function normally in vitro and ex vivo after transfusion into CR3-deficient mice (Hoffmeister et al. 2003a).
During haemostasis, GPIbα on the surface of the resting discoid platelet functions to bind the activated form of vWF at sites of vascular injury. Cooling of platelets, however, causes GPIbα clustering rather than internalization. The observation that GPIbα interacts separately with vWF and with CR3 suggests that selective modification of GPIbα might inhibit cold-induced platelet clearance without impairing GPIbα’s haemostatically important reactivity with vWF. Prolonged refrigeration increases the density and concentration of exposed galactose residues on platelets such that hepatocytes, through Ashwell-Morell receptor binding, become increasingly involved in platelet removal (Rumjantseva et al. 2009).
Storage of Platelets in the Frozen State: Still Not Ready for Prime Time
Although several methods for cryopreservation of platelets have been described (see below), most clinical experience has been with two methods, 5% DMSO, freezing at 1°C per minute using liquid nitrogen, controlled rate freezers, and storage in the gas phase of liquid nitrogen for at least 3 years, or 6% DMSO at −80°C for 2 years (Valeri and Ragno 2006). The selection of 6% DMSO was accidental, the result of a miscalculation of the dilution (CR Valeri, personal communication). Platelets frozen with 5% DMSO and stored in the gas phase of liquid nitrogen have been infused without first removing the DMSO, or concentrated after thawing to remove the supernatant DMSO prior to transfusion (Schiffer et al. 1978; Daly et al. 1979).
Four methods of freezing platelets have been compared:
The best clinical results, obtained with method (1), showed mean platelet recovery and 24-h increment to be significantly better than with the other methods. Nevertheless, the post-transfusion increment was only about 30% of that of fresh platelets. In three out of eight patients receiving chemotherapy, episodes of epistaxis and moderately severe haemorrhage from the oral mucosa were arrested by transfusion of platelets frozen in 6% DMSO, and six of the eight patients were supported solely with such platelets until complete haematological recovery occurred (Angelini et al. 1992). Single-donor leucoreduced platelets obtained by plateletpheresis have been treated with 6% DMSO, and after the supernatant DMSO is removed, the platelets are frozen in a −80°C freezer and stored at −80°C for 2 years. After dilution of the thawed platelets with 10 ml of 0.90 NaCl the platelets can be stored at room temperature without agitation for six hours.
Post-transfusion recovery and survival do not necessarily correlate with function and efficacy. Frozen, deglycerolized platelets may be considered a ‘haemostatic’ as well as a platelet transfusion, since they appear to be effective in the bleeding patient. In a controlled study, allogeneic previously frozen washed platelets had lower post-transfusion survival values than the allogeneic liquid preserved platelets, but they resulted in significant reductions in both non-surgical blood loss and the need for RBCs and FFP during the postoperative period than did the liquid preserved platelets and they produced no untoward effects (Khuri et al. 1999). A modified method of platelet freezing with 6% DMSO where the supernatant DMSO is removed prior to freezing eliminates the need for post-thaw washing and simplifies the cryopreservation procedure (Valeri et al. 2005). The amount of residual DMSO in the previously frozen washed platelets was 400 mg/unit whereas that in the previously frozen non-washed diluted platelets was 600 mg/unit. There is no significant difference in the safety profile between 400 mg and 600 mg of DMSO in the frozen platelets processed using the old and new methods. The time required for thawing and washing previously frozen platelets resuspended in plasma was 1 h and 15 min using the old freezing method with DMSO, whereas the time required for thawing and diluting the previously frozen non-washed platelets with 0.9% NaCl was only 15 min with the new freezing method with DMSO. The in vivo recovery 1–2 h after the autotransfusion of the platelets that were frozen with the new method with DMSO, diluted with 0.9% NaCl and stored at room temperature for 4 h was about 30% with a lifespan of 7 days compared to that of 35–40% and a lifespan of 7 days for the platelets frozen with the older method with DMSO, washed and resuspended in plasma (Valeri et al. 2005).
One strategy for managing immune refractory patients scheduled to receive repeated courses of chemotherapy is to collect autologous platelets during periods of haematopoietic recovery and freeze them for use during myeloablation. In a creative modification of this method Vadhan-Raj and co-workers (2002) administered recombinant human thrombopoietin at 2.4 g/kg to chemotherapy-naive patients with gynaecological malignancies, and cryopreserved the resulting autologous apheresis platelets in a mixture of ThromboSol and 2% DMSO. Subsequent transfusion of these autologous frozen platelets resulted in good increments in corrected counts, including in patients who were alloimmunized, allowing delivery of multiple cycles of intensive chemotherapy (Vadhan-Raj et al. 2002). Logistics, cost and potential side-effects of thrombopoietin make this approach impractical for all but a few particularly vexing alloimmunized platelet recipients.
Indications for Platelet Transfusions
Hypoproliferative Thrombocytopenia
Bleeding patients with severe thrombocytopenia and impaired platelet production require platelet transfusion support. However, most platelet transfusions are administered not to stem bleeding but to prevent it (McCullough et al. 1988). An autopsy study from the early years of leukaemia chemotherapy reported that 63% of leukaemia patients suffered haemorrhagic deaths in the year before prophylactic platelet transfusions were introduced (Han et al. 1966), a statistic that must seem incredible to those who trained in the era of platelet transfusion. Nevertheless, no controlled study has demonstrated that prophylactic platelet transfusions are superior to a strategy of timely transfusion when bleeding occurs.
A substantial body of evidence supports the value of prophylactic transfusions to prevent patients with a platelet count below 20 × 109/l from bleeding. In a seminal publication, Gaydos and co-workers (1962) reported that serious bleeding on 92 children with acute leukaemia occurred on only 4% of hospital days when the child’s platelet count exceeded 20 × 109/l, but on 10–30% when the count fell below 5 × 109/l. Too often forgotten is the absence of any obvious threshold effect for increased bleeding in their data analysis (Figure 14.4).
Figure 14.4 Relationship between platelet count and percentage of days with bleeding. Percentage of days with bleeding in 92 children with acute leukaemia: I, all bleeding; II, minor bleeding defined as skin and mucous membrane (purpura, epistaxis); III, significant bleeding (haematuria, haematemesis, melaena).
(Source: Adapted from Gaydos et al. 1962. Reproduced with permission of the Massachusetts Medical Society.)
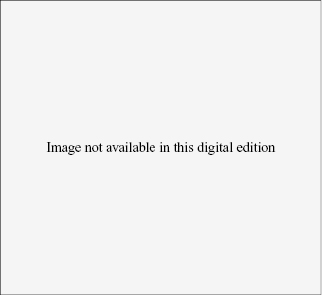
Furthermore, during this period, the patients were administered aspirin as an antipyretic, which undoubtedly increased their bleeding tendency at higher platelet levels.
Controlled studies of this era were small, and the platelet concentrates would not be equivalent to those currently used. In a study of 62 leukaemic children, serious bleeding occurred in only 5% of patients with a platelet count between 20 and 40 × 109/l, but in 26% of those with counts <10 × 109/l. A double-blind randomized study of 21 patients with acute leukaemia confirmed the benefit of prophylaxis and observed that fever preceded haemorrhage in 10 out of 13 cases (Higby et al. 1974). Using the 20 × 109/l trigger for prophylaxis, Murphy and co-workers (1982) found a significant reduction in bleeding, but no reduction in mortality, when 28 acute leukaemia patients treated with prophylactic platelets were compared with control subjects. An earlier study, documented in letter form only, of 31 consecutive patients with acute non-lymphoblastic leukaemia who were similarly randomized reported comparable mortality in both study arms (Solomon et al. 1978).
The only modern study that randomized patients between prophylactic and ‘therapeutic’ platelet transfusion involved patients undergoing intensive chemotherapy for acute myeloid leukaemia or autologous haematopoietic stem cell transplant for haematologic malignancies at eight centres in Germany (Wandt et al. 2012). Single-donor and whole blood- derived buffy coat platelets, both leucoreduced, were used. Patients refractory to transfusions, those who had experienced previous haemorrhage, or had additional haemostatic defects were excluded. Among 396 patients randomly assigned to receive either platelet transfusion when bleeding occurred (therapeutic arm, 197) or when morning platelet counts were at or below 10 × 109 per litre (prophylactic arm, 197), more severe bleeding was seen in the therapeutic patients who were being treated for leukaemia, whereas no difference in bleeding was noted between the two arms in the transplant group; six patients suffered cerebral haemorrhage in the treatment arm and two of these were fatal. Unfortunately the study was designed to evaluate platelet usage and was not powered to evaluate patient outcomes. Furthermore, some 22% of patients in the therapeutic arm received prophylactic platelets ‘off protocol.’ What is apparent from these data, although not the interpretation of the authors, is that whereas the ‘treatment’ group received significantly fewer platelet transfusions, even among stable patients, the risk of severe, even fatal haemorrhage is reduced by prophylactic treatment.
Experimental and clinical data support a transfusion trigger well below 20 × 109/l for stable, non-infected, afebrile thrombocytopenic patients who have no additional anatomic or functional defects in haemostasis. Studies of patients with aplastic anaemia found that daily stool blood loss measured with radiolabelled red cells increased strikingly when the platelet count fell below 5 × 109/l (Figure 14.5) (Slichter and Harker 1978).
Figure 14.5 Stool blood loss based on platelet count. Stool blood loss (expressed as ml/day) was determined for 20 patients with stable aplastic thrombocytopenia and radiolabelled red cells. At a platelet count >10 × 109/l, blood loss averaged 9 ml/day, whereas at a count of < 5 × 109/l, stool blood loss rose to 50 ml/day. Bleeding increased at every level when patients received prednisone (open circles) or semisynthetic penicillin (open triangles).
(Source: Adapted from Slichter and Harker 1978. Reproduced with permission of Elsevier.)
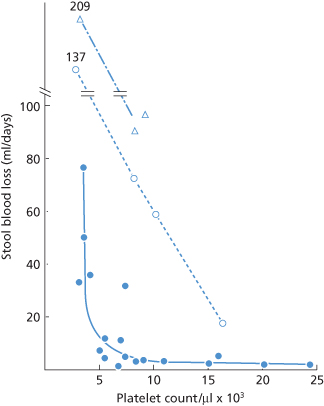
Results of a 10-year experience in 103 patients with acute myeloid leukaemia indicate that for patients without other risk factors, such as bleeding or fever, a platelet count of 5 × 109/l is adequate to prevent bleeding; for patients with such risks the count was kept above 10 × 109/l and for those with an additional coagulation defect, or with anatomical lesions, the count was kept above 20 × 109/l (Gmur et al. 1991). Three fatal haemorrhages occurred. The serious episodes of haemorrhage often occurred at relatively high platelet counts, emphasizing the importance of clinical factors as the cause of bleeding.
In 78 patients undergoing induction therapy for acute leukaemia who were randomized to receive prophylactic apheresis platelet concentrates, a 10 × 109/l threshold for prophylactic transfusion proved safe (Heckman et al. 1993). A subsequent large multicentre trial enrolled 255 assessable patients in 21 Italian centres (Rebulla et al. 1997). Adult acute leukaemia patients during the first remission induction were randomized to the traditional 20 × 109/l threshold or to a threshold of 10 × 109/l when in stable condition (but 20 × 109/l in the presence of fresh haemorrhage, fever greater than 38°C or invasive procedures). The lower threshold was associated with a 21.5% reduction in platelet requirements, yet no significant differences in the number of red cell transfusions, severe bleeding episodes or deaths during induction were detected. Overall, clinically significant haemorrhages occurred in 3.1% and 2% of the days in the lower and standard arms respectively.
The most contemporary evidence supporting the safety of the 10 × 109/l threshold involved a multicentre trial in Germany that studied 105 leukaemia patients undergoing 216 remission–induction or consolidation treatment cycles in 17 centres (Wandt et al. 1998). Individual participating centres had previously chosen to adopt either a 20 or a 10 × 109/l threshold level for their prophylactic transfusion policy. Twenty bleeding complications (18%) were recorded in 110 chemotherapy cycles in the lower threshold and 18 (17%) in 106 cycles in the higher one. Haemorrhagic deaths occurred in two patients at platelet counts of 36 and 50 × 109/l, treated in hospitals using the higher threshold. Similar data have been published for 159 patients undergoing haematopoietic stem cell transplant (Zumberg et al. 2002).
In summary, the venerated ‘twenty-thousand’ threshold represents a guideline to be modified based on the patient’s diagnosis, clinical status and treatment. No algorithm replaces bedside evaluation. A threshold of 10 × 109/l appears to be safe for prophylactic platelet transfusion in stable adult patients and children receiving therapy for acute leukaemia, although it is possible that this group of patients may not need prophylactic transfusions at all. Transfusion at higher levels may be advisable for newborns, for patients with signs of haemorrhage, high fever, mucositis, rapidly falling platelet count or other coagulation abnormalities (e.g. acute promyelocytic leukaemia) and for those undergoing invasive procedures (Schiffer et al. 2001). The importance of a post-transfusion platelet count to confirm the expected efficacy of transfusion cannot be overemphasized.
Prophylactic Platelet Transfusions for Invasive Procedures
The generally accepted platelet threshold for patients undergoing invasive procedures is 50 × 109/l. A review of 95 patients with acute leukaemia who underwent 167 procedures, of which 70% were classified as major surgery, revealed no procedure-related deaths or excess bleeding when the platelet count exceeded this level (Bishop et al. 1987). Platelet count was maintained at this level for three postoperative days in the case of surgery. No studies address either the safety of a threshold below 50 × 109/l or delicate ophthalmological or neurosurgery in which a higher threshold may be prudent.
Few data serve to guide the use of platelet transfusions for other invasive procedures. Eight thrombocytopenic patients developed spinal subdural haematoma, an otherwise relatively uncommon complication, following lumbar puncture; five out of the eight had a platelet count below 50 × 109/l; no patient with a normal platelet count developed this complication during the same 4-year period (Edelson et al. 1974). A review of 5609 consecutive lumbar punctures in 956 children with acute leukaemia over a 15-year period reported no increased risk of neurological or haemorrhagic complications, even with platelet counts as low as 20 × 109/l (Howard et al. 2002). However, all platelet counts of 100 × 109/l or less were associated with an increased risk of traumatic and bloody lumbar puncture. In a review of 291 consecutive liver biopsies (155 standard, 22 fine needle) in thrombocytopenic patients, the frequency of bleeding was the same (3.4%) for patients at a platelet count between 50 and 99 × 109/l as for patients with a mid-range normal count (McVay and Toy 1990). However, liver biopsy preceding a precipitous fall in platelet count has been associated with a lethal delayed haemorrhage (Reichert et al. 1983). Gastrointestinal endoscopy (Chu et al. 1983) and fibreoptic bronchoscopy in thrombocytopenic patients have not resulted in additional serious complications, although one life-threatening episode of severe epistaxis occurred during a transoral bronchoscopy in a patient who had prior epistaxis and a platelet count of 18 × 109/l (Weiss et al. 1993).
Studies of Dose
When a prophylactic transfusion strategy is followed, the optimal quantity of platelets, in addition to the transfusion trigger, has to be determined. Several small studies first examined dose for by comparing the ‘standard’ platelet dose (300–600 × 109/l) to a lower dose (150–300 × 109/l), a higher dose (> 600 × 109/l), or both (Norol et al. 1998; Klumpp et al. 1999; Sensebé et al. 2005). Most trials relied on surrogate endpoints such as time to next transfusion or number of platelet transfusions required. Not surprisingly, lower doses resulted in lower increments, shorter intervals, and more transfusion episodes (exposures). Two large, randomized, controlled studies of platelet dose with clinical bleeding as endpoints have been published (PLADO, Prophylactic PLAtelet Dose, and StoP, Strategies for the Transfusion of Platelets) (Slichter et al. 2010; Heddle et al. 2009). In PLADO, low dose platelets led to a decreased number of platelets transfused per patient, an increased number of transfusions, but no effect on the incidence of bleeding. The StoP trial was terminated early when a higher rate of grade 4 bleeding in patients receiving low-dose prophylactic platelet transfusions was noted. Although interpretation of these trials continues to be debated, prudent practice might counsel that the ‘standard’ dose and trigger be used for prophylaxis of at-risk patients with hypoproliferative thrombocytopenia; larger doses seem advisable for those who require longer transfusion intervals, such as outpatients or matched patients with limited numbers of available donors, whereas lower doses seem relatively safe when a standard dose is not available.
Inherited Qualitative Defects in Platelets
Patients with an inherited defect of platelet function (Wiskott–Aldrich syndrome, Bernard–Soulier syndrome and Glanzmann disease) should be treated with platelet transfusions when serious bleeding occurs, or before operation. In the last two disorders, alloimmunization, especially to GP IIb-IIIa in the case of Glanzmann disease, poses a particular problem, so that transfusions should be administered prudently (Kunicki et al. 1987). For alloimmunization related to other antigens, see Chapter 13.
Acquired Qualitative Defects in Platelets
An acquired defect of platelet function, due apparently to damage by passage through a pump oxygenator, is sometimes observed in patients who have undergone cardiac bypass procedures. Troublesome bleeding may be observed even with a platelet count above 50 × 109/l. In these circumstances, transfusion of normal platelets may be effective (Moriau et al. 1977). Platelet transfusions may also have a role in managing patients receiving medications that interfere with platelet function, particularly those who are bleeding or require urgent surgery. Thioprenidines such as the antithrombotic agent clopidogrel (Plavix), specific inhibitors of ADP-stimulated platelet function, result in increased blood loss and red cell transfusion for coronary artery bypass graft surgery (Gansera et al. 2003). However, neither this drug nor its carboxylic acid metabolite inhibits platelets in vitro, suggesting that transfused platelets are unaffected (Bennett 2001). Ordinarily suspending administration of the medication for 2–4 days prior to a surgical procedure suffices, but platelet transfusion is occasionally desirable. The monoclonal antibody Fab fragment abciximab (Reopro) blocks the platelet IIb/IIIa receptor and produces a Glanzmann-like effect. Unbound abciximab is rapidly cleared from circulation and little or no drug remains in serum or bound to tissues other than platelets. Subsequent platelet transfusion restores the platelet aggregation response, because previously bound abciximab redistributes to the new platelets, thus decreasing the overall percentage of blocked GPIIb/IIIa sites to fewer than the approximately 80% necessary to interfere with platelet aggregation and bleeding time (Coller 1997; Lemmer et al. 2000).
Thrombocytopenia Due to Immune Destruction of Platelets
ITP is a heterogeneous disorder that results most often from increased peripheral platelet destruction. Acute ITP often follows a viral infection and resolves spontaneously within a month or two. By definition, ITP that persists for more than 6 months without apparent specific cause is labelled ‘chronic ITP.’ The role of the spleen in platelet removal, first noted by a Viennese medical student in a case report of successful treatment, was confirmed in a series of patients treated with splenectomy (Kaznelson 1916; Doan et al. 1960). The seminal studies by Harrington and Moore demonstrated that the signs and symptoms of ITP could be reproduced in healthy subjects by infusing plasma from most – but not all – patients with ITP (Harrington et al. 1951). However the pathophysiology of these disorders proved to be complex and inadequate platelet production clearly plays a major role in some patients.
The classic therapeutic approach to ITP involved impeding immune sequestration of platelets, reducing antibody formation, and stimulating platelet production (see also Chapter 18). Treatments have included corticosteroids, intravenous immunoglobulin (IVIG) and IV anti-D (monoclonal antibodies against the D antigen of the Rh system) (see Chapters 18 and 19), splenectomy, danazol, azathioprine, cyclophosphamide, and rituximab. As the role of platelet production has been recognized as increasingly important, thrombopoietic agents have assumed a prominent role (Barsam et al. 2011).
Platelet transfusions are seldom indicated in immune thrombocytopenia, but even the small increment and short persistence may be life-saving for the bleeding patient. With the exception of splenectomy, most therapies take several days to take effect. Contrary to common opinion, platelet transfusions can raise the platelet count in many patients with immune thrombocytopenia, and therefore may be beneficial in actively bleeding or high-risk patients with this disorder (Abrahm and Ellman 1976; Carr et al. 1986; Salama et al. 2008). Better increments and platelet survival may be achieved if transfusion is preceded by infusions of IVIG (Baumann et al. 1986). There is little evidence that platelet transfusion is deleterious in ITP and it may provide a life-sustaining bridge for the bleeding patient until more definitive therapy becomes effective.
Platelet transfusion in neonatal alloimmune thrombocytopenia and thrombotic thrombocytopenic purpura (TTP) is discussed in Chapter 13, and in post-transfusion purpura in Chapter 15. Suffice it to say that in thrombotic thrombocytopenic purpura and in the haemolytic uraemic syndrome, the transfusion of platelets is rarely needed and may aggravate the condition (Chapter 13). The effects of platelet transfusion in DIC are less predictable but platelet transfusions are often given when serious bleeding is associated with thrombocytopenia.
Some Other Aspects of Platelet Transfusions
Expected Platelet Increment Following Transfusion: When It Counts, It Should Be Counted
Transfusion of one unit of fresh platelets (containing approximately 0.7 × 1011 platelets) to an adult weighing 70 kg produces an immediate post-transfusion increment of approximately 11 × 109/l (Schiffer 1981). In order to make proper comparisons between different recipients, the number of platelets transfused and the approximate blood volume of the recipient must be taken into account. One formula defines the ‘corrected count increment’ as the rise in platelet count observed multiplied by the surface area (in metres squared) divided by the number of platelets transfused divided by 1011. For example, for a 70-kg adult with a surface area of 1.7 m2 and the other figures given above, the corrected increment would be:
Calculation of the corrected increment at 1 h after transfusion is valuable in three circumstances: (1) when stored platelets are being used, as a check on their efficacy; (2) when platelet transfusions are being given to patients who may have formed alloantibodies; and (3) to check whether HLA-matched or crossmatch-compatible platelets are effective.
Two other methods for calculating platelet increment have been recommended. The percentage platelet recovery (PPR) also adjusts for the number of platelets transfused and for the blood volume, and a regression analysis method claims to separate the effects of dose and cell quality (Davis et al. 1999). Neither has been widely used.
Risks from Red Cell Contamination
Platelet concentrates contain variable numbers of red cells and may induce Rh0D sensitization in a D-negative subject. The risk is small, particularly in patients receiving immunosuppressive therapy for leukaemia or solid tumours (Goldfinger and McGinniss 1971; Lichtiger and Hester 1986; Cid et al. 2011). When platelet concentrates from D-positive donors are transfused repeatedly to a D-negative woman who has not yet reached the menopause, provided that the recipient is not already immunized, a dose of anti-D immunoglobulin should be considered to suppress primary Rh0D immunization. For further details, see Chapter 5.
Platelet Concentrates from Cytomegalovirus Antibody-Negative Donors
The consequences of primary infection with cytomegalovirus (CMV) can be serious, even fatal in immunosuppressed patients. For those patients with compromised immunity who are anti-CMV negative, platelet concentrates from anti-CMV-negative donors (see Chapters 13 and 16) should be made available; alternatively, the platelet concentrate should be depleted of white cells (‘CMV-safe’) to reduce the risk of CMV transmission (Gilbert et al. 1989; Bowden et al. 1995).
Platelets from Donors Ingesting Aspirin (ASA): A Headache for Blood Collectors
Aspirin irreversibly acetylates serine 530 of cyclooxygenase (COX)-1, which inhibits release from platelets of the potent platelet activator thromboxane A2. Because platelets lack the synthetic machinery to generate significant amounts of new COX, aspirin-induced COX-1 inhibition lasts for the lifetime of the platelet (Patrono et al. 2001). Within two hours of ingestion, a single dose of ASA (650 mg) inhibits a number of ex vivo platelet functions including the aggregation response induced by collagen, and the second wave of platelet aggregation induced by adenosine diphosphate and epinephrine (Weiss and Rogers 1972). The effect lasts for up to 4 days as increasing numbers of newly generated platelets correct the functional abnormalities. None of the in vitro assays of platelet function accurately predict bleeding risk. However patients who ingest ASA develop a mild defect in haemostasis and tend to lose more blood during surgery than do non-aspirinized subjects. Variability in platelet response to ASA among patients and normal volunteers is well documented. A variety of mechanisms have been postulated for this so-called ‘aspirin resistance’ (Patrono et al. 2001).
The frequency of ASA ingestion among potential platelet donors presents a conundrum to blood centers. Older studies indicate that about one third of all volunteer blood donors recall ingesting ASA in the week prior to blood donation (Mielke and Britten 1972). Daily low dose ASA (81 mg) is now commonly ingested as prophylaxis for stroke and cardiovascular disease. Few studies have addressed the impact of transfusing platelets derived from donors who have ingested ASA and relevant studies are difficult to design. Manual plateletpheresis concentrates calculated to raise the platelet count by approximately 100 000/μl were transfused to thrombocytopenic children with aplastic anaemia or leukaemia (Stuart et al. 1972). Prolonged bleeding time was corrected to control levels within 1 h in recipients of platelets from donors who had ingested 600 mg ASA 36 h prior to donation, but remained prolonged and unchanged at 1 h and 4 h in those who received platelets from donors who had ingested 600 mg ASA 12 h and 1 h before donation. These bleeding time data have been the basis of the 36 hour deferral for donors who have ingested ASA. Of additional interest was a correlation between the bleeding time and the number of circulating native platelets indicating a significant decrease toward normal in patients whose native platelets represented 10% or more of the 1 h total platelet count. In a separate series of volunteers, these investigators also noted that aspirin dose (600–3600 mg over 24 h) did not affect the return toward normal of bleeding time (36 h) or aggregation response (24–72 h). In an often overlooked study of platelet storage, Slichter and Harker studied paired transfusions of apheresis platelets from 6 donors before ASA ingestion and after 1.2 g ASA twice daily for 3 days and on the morning of apheresis. Two SAA recipients were evaluated carefully for BT correction. Stored or unstored aspirinized platelet transfusions showed a 7 h delay in correcting the bleeding time in one patient and an 18 h delay in the second. While this study does not address the donor deferral issue, it does suggest that platelets from donors who have ingested ASA show a time-dependent reversal of their dysfunction which is most marked in the first 4–6 h post-transfusion (Slichter and Harker 1976). This finding suggests that alternative pathways may be responsible for platelet function from aspirinized donors.
The Transfusion of Granulocytes
A direct relationship between the number of circulating granulocytes and the risk of bacterial infection has been recognized for 40 years (Bodey et al. 1966). Early studies of granulocyte transfusions were limited by the short survival of circulating granulocytes, their poor viability when stored for more than a few hours and the difficulty in collecting sufficient numbers of cells to measure a post-transfusion increment. Freireich and co-workers (1964) elected to study the dose response of transfused granulocytes by collecting cells from adult donors (patients) with chronic myelocytic leukaemia (CML) and infusing them into granulocytopenic children. The initial 118 transfusions established several fundamental precepts: (1) the 1-h post-transfusion increment correlates directly with the number of granulocytes infused; (2) an estimated 1011 granulocytes per square metre of body surface area, more than the total number calculated to circulate in normal subjects, are needed to raise the recipient’s circulating granulocytes by 1000/μl; (3) no increment is detected if fewer than 1010 cells are infused; and (4) clearance of circulating Gram-negative bacteria and resolution of fever occurred in most (10 out of 11) children who received more than 1010 granulocytes/m2 (Frei et al. 1965). These studies established the basic principles of granulocyte transfusion. However, cells from patients with CML had two advantages over the mature granulocytes collected from normal volunteers during the following 30 years. First, these collections contained granulocyte precursors that circulated and matured for 24 h or more. Second, some of these cells clearly engrafted transiently in some of the immunosuppressed patients and proliferated for several days; in one case, Philadelphia chromosome- positive cells were still circulating 52 days after transfusion. Both factors probably contributed to the efficacy of the early treatments and made comparisons with these data difficult until methods to mobilize cells from normal subjects became available (Frei et al. 1965).
Labelling of Granulocytes: distribution in the Circulation
When labelled granulocytes are injected into the bloodstream, they equilibrate with the total blood granulocyte pool, consisting of cells that ‘marginate’ along, or adhere to, the walls of venules and others that circulate freely. Following intravenous injection of DF32P-labelled granulocytes, the concentration in the bloodstream is only 45% of that expected if all the granulocytes circulated freely (Cartwright et al. 1964). This figure may slightly underestimate the true percentage of neutrophils that circulate freely, because some DF32P elutes rapidly from labelled granulocytes (Dancey et al. 1976). Moreover, when granulocytes were labelled in vivo with 3H-thymidine and then transfused to another normal subject, the maximum recovery was 57%, suggesting that only 43% of the granulocytes (rather than 55%) were marginated (Dancey et al. 1976). However, using 111In-labelled granulocytes, the marginating granulocyte pool was calculated to be 60% of the total blood granulocyte pool (Peters et al. 1985). Granulocytes labelled with 3H-thymidine disappear from the bloodstream with a T1/2 of 7.6 h. Based on this finding and other data, daily production of granulocytes was estimated to be 0.85 × 109/kg, about one-half of the previous estimate obtained with DF32P. The total marrow metamyelocyte–granulocyte pool was estimated to be 6 × 109/kg (Dancey et al. 1976). In a 70-kg man the total pool of marrow granulocytes is thus about 4.2 × 1011. Taking the granulocyte count in circulating blood at 4.0 × 109/l and assuming a blood volume of 5 litres in a 70-kg man, total circulating granulocytes are estimated to be 2 × 1010 or about one-twentieth of the total marrow granulocyte pool. This estimate agrees well with data obtained following leucapheresis, which indicate an ‘extracirculatory’ reservoir of granulocytes of about 18 times the total number of granulocytes in the circulation (Bierman et al. 1962).
111In combined with a suitable chelating agent is the best label for granulocytes: little elution of the label occurs in vivo and labelled granulocytes can be detected readily by surface scanning. Following the injection of 111In-labelled granulocytes, label accumulates immediately in the lungs, but granulocytes then redistribute to the liver and spleen. Granulocytes labelled with 111In were cleared from the circulating blood with a T1/2 of 7.5 h, confirming results obtained with 3H-thymidine (Thakur et al. 1977a). 111In-labelled granulocytes have been used successfully for the localization of abscesses (Thakur et al. 1977b; Dutcher et al. 1981) and, combined with imaging, have been used to study the distribution and sites of destruction of granulocytes in normal subjects (Saverymuttu et al. 1985).
Storage of Granulocytes
Storage in the Liquid State
Granulocytes do not store well. The effect of liquid storage on granulocyte function has been studied by measuring a variety of morphological markers, chemical changes in the supernatant, alterations in cell function in vitro, and cell recovery and circulation in vivo. Functional assays such as chemotaxis, phagocytosis, chemiluminescence and superoxide anion formation are not practical measurements for routine quality control. However, when the method of collection or of storage has been modified, granulocyte testing by a panel of functional assays should be undertaken. The definitive measure of efficacy is control of infection in the compromised host.
Of the in vitro assays, granulocyte chemotaxis is most often used as the surrogate measure of cell quality. Chemotaxis of neutrophils is maintained satisfactorily for about 8 h at 20–24°C, but is rapidly impaired by storage at 4°C (Lane and Windle 1979). The severe impairment of chemotaxis of granulocytes after prolonged storage is probably caused by a lesion of the cytoskeleton. After storage at 4°C, microfilaments are disorganized and few microtubes are visible (Palm et al. 1981). At room temperature, neutrophils consume glucose and maintenance of glucose concentration is essential for maintaining function (Glasser et al. 1985). The addition of bicarbonate to neutralize lactic acid formed as a result of glycolysis, and thus to maintain pH at acceptable levels, helps to maintain chemotactic function (Lane and Lamkin 1984). Granulocytes stored in the absence of protein deteriorate rapidly, but chemotaxis is maintained just as well in a synthetic medium supplemented with albumin as in autologous plasma (Glasser et al. 1985). Granulocytes stored for 24 h at either 1–6°C or 20–24°C have significantly reduced intravascular recovery and migration into skin windows. Granulocytes stored for only 8 h at 20–24°C have normal intravascular recovery and migration into skin windows (McCullough et al. 1983). Agitation of granulocytes during storage rapidly increases the rate at which their function deteriorates (McCullough et al. 1978).
Stimulating donors with granulocyte colony stimulating factor (G-CSF), or G-CSF with dexamethasone, increases the yield of granulocytes compared with the yield from the traditional collection regimen of dexamethasone alone (Dale et al. 1998; Liles et al. 2000; Stroncek et al. 2001). When granulocytes are incubated with G-CSF or dexamethasone, apoptosis is delayed and in vitro survival prolonged. However, the effect does not appear to extend to cells collected from donors mobilized with these agents. The high cell counts in G-CSF- and G-CSF-plus-dexamethasone-mobilized concentrates cause a rapid decrease in pH, which limits component shelf-life. Dilutions of 1:8 to 1:16 with autologous plasma can maintain the pH of stored granulocyte concentrates, but the component volumes are impractical (Lightfoot et al. 2000). For the large number and high concentration of granulocytes in collections mobilized with G-CSF (see below), cell counts, viability and pH can be well maintained after 2, 24 and 48 h when cells are stored in the three cell culture media (Lightfoot et al. 2001). None of these media is approved for in vivo use. Standard infusible solutions are not buffered adequately and lack sufficient protein, but infusible solutions, such as lactated Ringer’s solution or PlasmaLyte A supplemented with buffers and albumin, hold promise as effective and licensable solutions for granulocyte storage.
Storage in the Frozen State
Critical granulocyte functions are impaired severely after freezing and subsequent thawing (Frim and Mazur 1980). Cryopreserved granulocytes are not satisfactory for transfusion purposes.
Irradiation of Granulocyte Concentrates
Granulocyte concentrates inevitably contain large numbers of immunocompetent T lymphocytes. Because many potential granulocyte recipients, especially those undergoing allogeneic transplantation procedures, are susceptible to transfusion-associated graft-versus-host disease, these cells are routinely exposed to 25-Gy gamma irradiation. Some early studies suggested that granulocytes collected by centrifugation are damaged by this irradiation dose (Buescher and Gallin 1987); however, studies of mobilized cells indicate that both phagocytosis and migration are preserved (Caspar et al. 1993).
Indications for Granulocyte Transfusions
Under normal circumstances, two and a half times the number of granulocytes present in the circulation at any given time is consumed each day. Granulocyte turnover can increase by an order of magnitude in the face of infection. Thus, for transfusions to be effective in neutropenic patients with life-threatening bacterial or fungal infections, large numbers of granulocytes must be administered repeatedly. If normal production in an adult is about 1011 granulocytes per day and if only 5–10% of the granulocyte pool is in the circulation, at least 2.1 × 1010 granulocytes should be transfused per day (Strauss 1993). To obtain such numbers without resorting to granulocytes from patients with chronic granulocyte leukaemia, the donor must be stimulated (mobilized) with cytokines, steroids or both, and blood cell separators must use an erythrocyte sedimenting agent such as hydroxyethyl starch during collection (Dale et al. 1998; Liles et al. 2000; Stroncek et al. 2001, 2002) (see also Chapter 17). Once granulocyte therapy has been started, transfusions should be continued for 4–7 days before reassessing patient status.
Therapeutic Granulocyte Transfusion in Infected Neutropenic Patients
In Adults and Children
In the era prior to cytokine stimulation of donors, several prospective, randomized, controlled clinical trials have shown an increased survival in neutropenic patients who are given leucocyte transfusions in addition to antibiotics (Higby et al. 1975; Alavi and Root 1977; Herzig et al. 1977; Vogler and Winton 1977). Seven controlled studies have been reviewed in detail (Klein et al. 1996). Most of the patients studied suffered from Gram-negative septicaemia. Significant benefit from the transfusions was seen only when bone marrow recovery was delayed for more than 1 week, but did occur within 2 or 3 weeks when leucocyte transfusions were given daily for at least 4–7 days in patients with proven infections. However, the number of patients studied in these trials was small, the infections were heterogeneous, and the duration of granulocytopenia differed. In a later trial, no benefit was conferred by transfusion of granulocytes; patients treated with antibiotics alone survived just as well (Winston et al. 1982), but the number of granulocytes transfused (approximately 5 × 109 per transfusion) was totally inadequate (Schiffer 1990). Granulocyte transfusions have been reported to produce favourable results in patients with chronic granulomatous disease with pyogenic or fungal infections (Yomtovian et al. 1981). Several weeks of therapy may be required.
Although cytokine mobilization of donors has resulted in concentrates containing impressive numbers of granulocytes, evidence for clinical efficacy still remains largely anecdotal. In patients with haematological malignancies, bacterial infections appear to respond well to a course of granulocyte therapy, even given the lack of controls and the difficulty in separating the effect of transfusion from that of antibiotics and systemic growth factor administration (Peters et al. 1999; Price et al. 2000; Quillen et al. 2009; Cherif et al. 2012). Although these infusions appear to be more effective against bacterial sepsis than against deep-seated fungal infections, case reports and uncontrolled small series are sufficiently promising that controlled studies with adequate numbers of compatible granulocytes should be carried out (Catalano et al. 1997; Dignani et al. 1997; Ozsahin et al. 1998). Quillen et al. (2009) performed a retrospective analysis of all patients with severe aplastic anaemia who had received granulocyte transfusions at NIH between 1997 and 2007. Survival to hospital discharge was the primary outcome, but secondary outcomes included microbiological, radiographic and clinical responses of the infection at 7 and 30 days after initiating granulocyte therapy, and post-transfusion absolute neutrophil count. Thirty-two patients with severe aplastic anaemia received granulocyte transfusions; the majority had been administered horse antithymocyte globulin and cyclosporine A. One quarter of patients had been alloimmunized to HLA antigens to granulocyte therapy. The infections were evenly divided between invasive bacterial and fungal infections unresponsive to optimal antibiotic and/or antifungal therapy. Patients received a median of 9 granulocyte transfusions per patient and overall survival to hospital discharge was 58%. Survival correlated strongly with haematopoietic recovery. Among the 18 patients who had invasive fungal infections, 44% survived to hospital discharge. Response at 7 and 30 days correlated with survival. The mean post-transfusion absolute neutrophil count did not differ significantly among patients who had complete or partial resolution of infection, stable disease or progressive infection. There was also no difference in mean post-transfusion absolute neutrophil count between the patients analyzed according to HLA alloimmunization status. Granulocyte transfusions likely played a role as a ‘bridge’ for managing the infections in these patients with severe aplastic anaemia. Unlike the patients with CGD, HLA alloimmunization did not regularly result in severe reactions and poor granulocyte recovery/survival and should not be an absolute contraindication to granulocyte therapy.
A patient treated at NIH illustrates the benefits and risks of granulocyte therapy:
Figure 14.6 Sequential CT scans of the thorax and response to granulocyte transfusions. CT scan before granulocyte transfusions showed nodules in the right upper lobe (Ai, solid arrow, 2 cm) and right middle lobe (Aii, dotted arrow, 3.5 cm). CT after 4 transfusions of granulocytes revealed a new right pleural effusion, new right lower and middle lobe consolidation, and left lower lobe airspace disease, and enlargement of existing nodules (Bi-ii). CT after 5 additional granulocyte transfusions showed improvement in lung consolidations (Ci-ii). The granulocyte (× 1010) and lymphocyte (× 109) content of each of the 9 transfused granulocyte components (arrows) is shown in the boxes above the arrows, with the corresponding increment in ANC shown below the arrows (D).
(Source: O’Donghaile et al. (2012).)
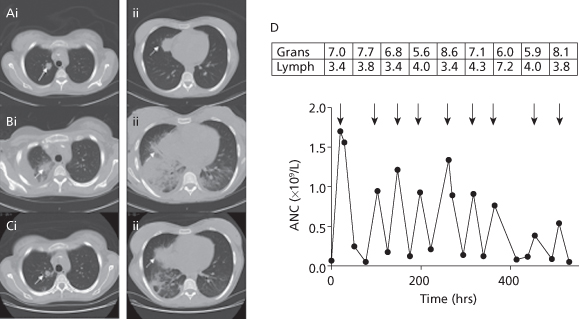
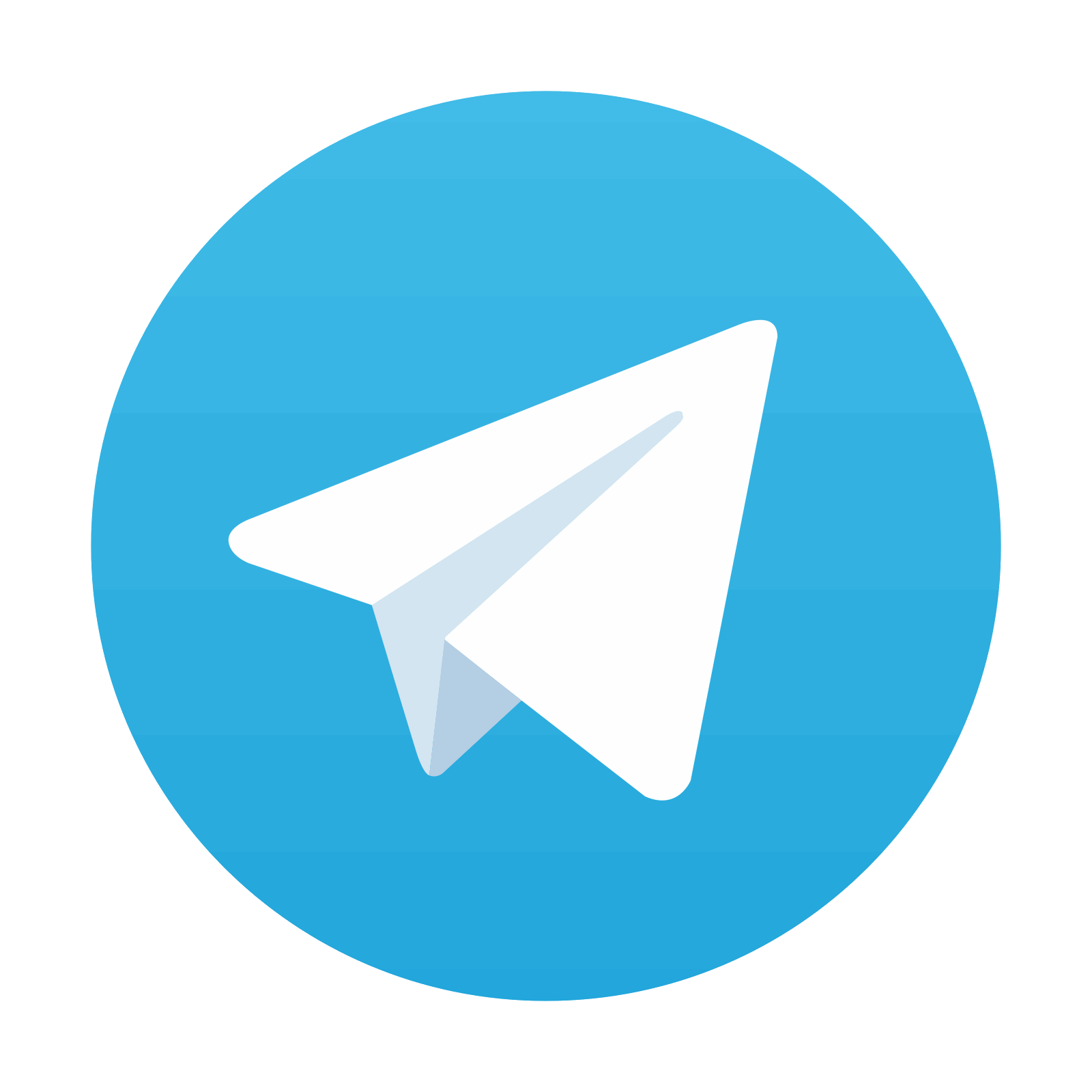
Stay updated, free articles. Join our Telegram channel

Full access? Get Clinical Tree
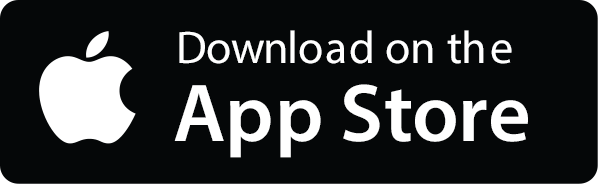
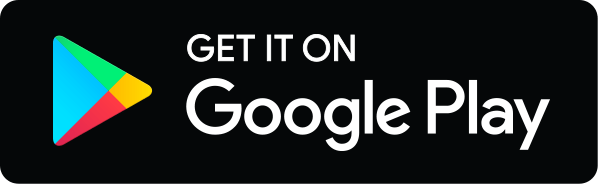